Abstract
Background:
In Streptococcus mutans, ComCDE, a peptide-induced two-component signal transduction system, forms a closed signal transduction, and even if difunctional ComE closes this signal at its headstream to avoid its infinite amplification, it is not enough for ComE to work in a concentration-dependent manner. CslAB has a chance to regulate ComCDE by controlling extracellular competence-stimulating peptide (CSP) concentration through its processing and secretion.Objectives:
To first confirm the binding properties of cslAB promoter (PcslAB) with ComE, then to uncover in vivo need of cslAB expression, and finally to unveil the role of CslAB.Materials and Methods:
Electrophoretic mobility shift assay was used to confirm the binding properties of PcslAB with ComE. In vivo cslAB transcription was detected by β-galactosidase activity because its gene has been fused to cslAB operon, and finally the role of CslAB was reviewed.Results:
PcslAB is a weak promoter responding to ComE and its binding appears to be negative cooperative. Although PcslAB is partially controlled by ComCDE, it can respond to ComCDE regulation. Supported by the obtained molecular evidence, CslAB acts as a stabilizer of ComCDE signal on the patterns of its expression.Conclusions:
PcslAB is partially controlled by ComCDE. CslAB is a stabilizer of ComCDE signal to ensure that ComE works in a concentration-dependent manner.Keywords
CslAB ComCDE Two Component Signal Transduction System Electrophoretic Mobility Shift Assay Streptococcus mutans
1. Background
Streptococcus mutans is the primary cause of dental caries; its pathogenicity is determined by ComCDE, a peptide-induced two-component signal transduction system (TCSTS), as this signal is involved in 4 vital physiological processes: competence development, bacteriocin synthesis, biofilm formation (dental plaque), and acid production (1-7). ComED consists of a response regulator, ComE; a histidine kinase sensor, ComD; while ComC is the competence-stimulating peptide (CSP) precursor (1-4, 8-12). By analogy to the model of S. pneumonia, CSP is firstly detected by ComD, then ComD activates its response regulator ComE, and finally ComE drives their downstream genes transcription (13, 14). In S. mutans, although ComCDE working mode is similar to that of S. pneumonia, its architecture appears to be very different. Firstly, ComCDE is organized in two operons (comC and comED), compared to one operon in S. pneumonia (8-10, 12-14), secondly, ComE binding sites in S. pneumonia work as direct repeat sequences, but in S. mutans have two patches, one patch of the direct repeat sequences can also work well (8-10, 12, 13, 15).
Another protein closely related to ComCDE signal is ABC transporter ComAB, as this complex processes and secrets CSP (13, 14). In S. mutans, ComAB (SMU.1897 and SMU.1898) was designated as CslAB, in which CslA is an ATP-binding cassette transporter and CslB acts as an accessory; their genes are organized in operon cslAB (16). Another homolog of ComAB (SMU.286 and SMU.287) was re-designated as NlmTE because it works in nonlantibiotic mutacin transport but not in genetic transformation (17). Given that CslAB processes and secrets CSP from ComC (CSP precursor) and ComE binding sites have been found in all promoters of comC, comED, and cslAB (9-12), then all three operons driven by ComE, ComCDE would form a closed signal transduction.
Recently, it was reported that comC expression could be inhibited by difunctional regulator ComE, so ComCDE could be closed at its headstream lest it was amplified limitlessly (9, 10, 12, 18). Furthermore, to expound the work patterns of ComCDE, It is suggested that ComE functions in a concentration-dependent manner (9, 10, 12). Obviously, it is necessary for ComCDE to be controlled precisely. CslAB determines extracellular CSP concentration since it is responsible for the processing and exporting of CSP (16, 17). Therefore, cslAB transcription was investigated to clarify the control principles of ComCDE. The promoter of nlmAB (PnlmAB) has a typical ComE binding site and is strictly regulated by ComCDE. As nlmAB transcription in vivo is clear (9, 11, 12, 15), it was used as a positive control.
2. Objectives
The binding properties of cslAB promoter (PcslAB) with ComE should be confirmed at first, then cslAB expression in vivo was investigated, and finally the role of CslAB in ComCDE signal would be confirmed.
3. Materials and Methods
3.1. Bacterial Strain and Medium
Bacterial strains were listed in Table 1. Escherichia coli was cultivated in LB medium at 37°C, if needed, kanamycin (50 μg/mL), ampicillin (100 μg/mL), or isopropyl β-D-thiogalactopyranoside (1 mM) were added to the medium. S. mutans and its derivatives were cultivated in Todd-Hewitt broth medium with 0.3% yeast extract and 0.5% sucrose at 37°C, if required either 750 μg/mL kanamycin or 25 μg/mL erythromycin was supplied. S.mutans was transformed according to Li et al. protocol (2). Todd-Hewitt broth medium (CM0189) was purchased from Oxoid Ltd, UK (http://www.oxoid.com/UK); the other reagents, if nonspecifically annotated, were obtained from Sangon Biotech., China (http://www.sangon.com).
Bacterial Strain and Vector
Strain and Vector | Relevant Characteristics | Reference (s) |
---|---|---|
Escherichia coli | ||
DH5α | supE44 lacU169 (80lacZ M15) hsdR17 recA1 endA1 gyrA96 thi-1 relA1 luxS | (19) |
BL21/p41ComE | BL21 (DE3) pLysS harbouring p41ComE to express fusion protein GST-ComE, KanR | (11) |
Streptococcus mutans | ||
WT | Wild type UA159, KanS ErmS | (20) |
WT/pA-LacZ | WT with LacZ drove by promoter PnlmAB via single cross integration, KanR | (11) |
ΔcomED/pA-LacZ | as WT/pA-LacZ but comED was knocked out by double cross of erm cassette substitution, KanR ErmR | (11) |
ΔcomC/pA-LacZ | as WT/pA-LacZ but comC was knocked out by double cross of erm cassette substitution, KanR ErmR | (11) |
WT/pAB-LacZ | WT with LacZ drove by promoter PcslAB | This work |
ΔcomED/pAB-LacZ | as WT/pAB-LacZ but comED was knocked out by double cross of erm cassette substitution, KanR ErmR | This work |
ΔcomC/pAB-LacZ | as WT/pAB-LacZ but comC was knocked out by double cross of erm cassette substitution, KanR ErmR | This work |
Vector | ||
pUCm-T | cloning T-vector, AmpR | Sangon (SK2211) |
pLacZ | a derivation of pSF151, lacZ was integrated in BamHI and SalI, KanR | (11) |
3.2. DNA Manipulation
Standard molecular cloning techniques were used. Restriction enzymes and T4 DNA ligase (EL0011) were obtained from Thermo Fisher Scientific Inc., China (http://www.thermo.com.cn). Taq DNA polymerase (R001B) was purchased from Takara Biotechnology (Dalian) CO., LTD., China (http://www.takara.com.cn). All plasmid extraction, DNA fragment purification, and DNA recovered from agarose gel were operated, respectively with SK8192, SK8142, and SK8132 kits obtained from Sangon Biotech., China.
3.3. Electrophoretic Mobility Shift Assay
ComE proteins were prepared according to Liu et al.’s protocol (11). The primers for amplification of the promoters were synthesized in Sangon Biotech., China (summarized in Table 2). Electrophoretic Mobility Shift Assay (EMSA) was set up according to Liu et al. and Jing et al. protocols (11, 21), but promoter DNA was fixed to 50 nM. In native PAGE (5% gel, V/V), 20 μL of binding mixture was directly loaded, then the gel was run in Tris-Glycine-EDTA buffer (25 mM) at 4°C. Finally, the gel was stained with SYBR Green I for 10 minutes. Hill equation was described with (Equation 1):

where ‘n’ is Hill coefficient and ‘Kd’ is microscopic dissociation constant (12, 22). To calculate Hill equation, retarded and free DNAs were quantified by Scion Image Alpha 4.03, then log (DNA bound/free) were regressed against log [ComE (nM)] with Linear Fit of OriginPro 8.07.
Primer | Primer Sequence (5' → 3’) | Restriction Enzyme Site | Position Targeted, bp | Application |
---|---|---|---|---|
PcslAB-F | 5’-AACAGCAATATCGTAAACGG-3’ | 1784148 to 1784405 | PcslAB promoter | |
PcslAB-B | 5’-GGAGTCTATCTGCGGAACAT-3’ | |||
PnlmAB-F | 5’-AAATTAGCTGGTAATGATAGTT-3’ | 153650 to 153770 | PnlmAB promoter | |
PnlmAB-R | 5’-GCAACCAACATCTTTAGTATAA-3’ | |||
CslB-BamHI | 5’-caGGATCCAGACTGTTGCTCAGTATCTC-3’ | GGATCC | 1786341 to 1787546 | cslB for WT/pAB-LacZ construction |
CslB-XbaI | 5’-ggTCTAGAACTATTGGTAAAGGCTAA-3’ | TCTAGA | ||
UpComED-F | 5’-GAACATAATTTACAGCGGTTCATA-3’ | 1796995 to 1797594 | upstream fragment of comED erm cassette | |
UpComED-HindIII | 5’-ggAAGCTTCAATGCGGTGGGAGAACT-3’ | AAGCTT | ||
DownComED-XhoI | 5’-gaCTCGAGTTAGGCGGGCAATCATATTC-3’ | CTCGAG | 1795545 to 1796323 | downstream fragment of comED erm cassette |
DownComED-R | 5’-AGCAGCCTCAATGGCATTAT-3’ | |||
UpComC-F | 5’-ATCTGAACAAGCAGGGGAGA-3’ | 1794256 to 1795020 | upstream fragment of comC erm cassette | |
UpComC-HindIII | 5’-gcAAGCTTGTGTTTTTTTCATTTTATATCTCC-3’ | AAGCTT | ||
DownComC-XhoI | 5’-taCTCGAGTCCGGCTGTTTAACAGAAGTT-3’ | CTCGAG | 1795105 to 1795947 | downstream fragment of comC erm cassette |
DownComC-R | 5’-GGCACAAAAGGAAGCTCAGA-3’ | |||
Erm-HindIII | 5’-gaAAGCTTCCGGGCCCAAAATTTGTTTGAT-3’ | AAGCTT | erythromycin gene | erm cassette construction |
Erm-XhoI | 5’-aaCTCGAGTCGGCAGCGACTCATAGAAT-3’ | CTCGAG |
3.4. Construction of S. mutans Derivations
All primers used here were listed in Table 2. WT/pA-LacZ, ΔcomED/pA-LacZ, and ΔcomC/pA-LacZ strains have been described before (11). A cslB fragment was amplified by PCR from strain UA159 and then cloned into pLacZ to construct plasmid pCslB-LacZ. S. mutans UA159 was transformed with pCslB-LacZ to get WT/pAB-LacZ via single crossover in which cslA, cslB and lacZ were fused together. To knock out comED and comC, erythromycin cassettes of comED and comC were prepared according to Liu et al. method (11). Then, they were transformed into WT/pAB-LacZ to knock out comC or comED by double crossover (15).
3.5. Determination of Promoter Activity
Streptococcus mutans overnight cultures were diluted 20-fold with fresh medium, if required CSP (1.0 μg/mL) and antibiotics were added, and then incubated for 2 or 3 hours at 37°C. Promoter activity was determined by β-galactosidase assay (LacZ) and calculated with the formula: [1000 × A420 / reaction time (min)] × OD675, in which the value of 420 nm absorbance for O-nitrophenyl-β-D-galactopyranoside color reaction and 675 nm absorbance for cell density were described with A420 and OD675 (23).
4. Results
4.1. PcslAB, a Weak Promoter Responding to ComCDE
EMSAs showed 3 retarded bands of BD1 to BD3 for PnlmAB and 2 bands of BD1 and BD2 for PcslAB. BD1 was firstly detected at 68.13 nM, ComE in lane 3 for PnlmAB and at 137.97 nM, ComE in lane 4 for PcslAB (Figure 1). For PcslAB and PnlmAB, Hill coefficients were 0.6946 and 0.9356, respectively, while microscopic dissociation constants (Kd) were 879.32 nM and 445.44 nM, respectively (Figure 1). All data suggested that to bind with ComE, the affinity of PcslAB was weaker than that of PnlmAB.
Electrophoretic Mobility Shift Assays (EMSAs) on PnlmAB and PcslAB
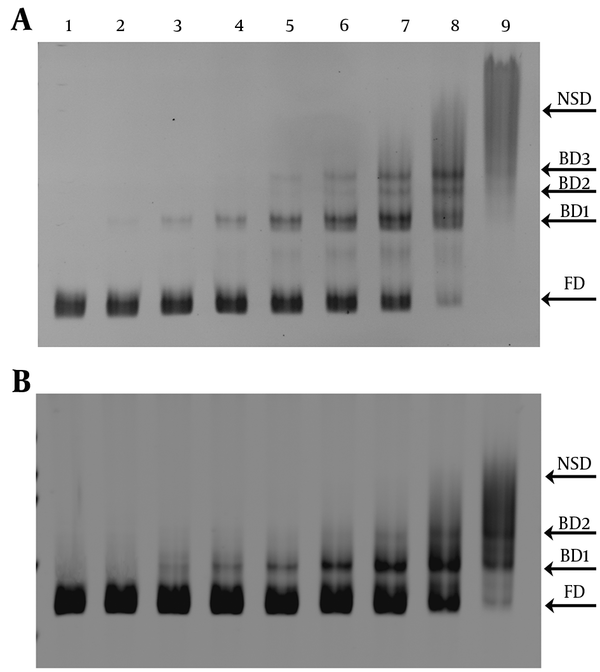
EMSAs on PnlmAB (A) and PcslAB (B) both adopt 50 nM promoter DNAs. Lane 1 to 9: ComE concentration gradient (0, 34, 68, 138, 278, 555, 1111, 2223, and 4446 nM). NSD, BD, and FD: Non-specifically binding DNA, retarded DNA and free DNA. Hill coefficient and Kd were 0.9356 and 445.44 nM for PnlmAB, and 0.6946 and 879.32 nM for PcslAB.
4.2. PcslAB Partially Controlled by ComCDE
PnlmAB was strictly controlled by ComCDE because the deletions of comED and comC both abolished PnlmAB activity, while exogenous CSP almost rescued the defects of comC but not the comED’s (Figure 2: PnlmAB). PcslAB is partially controlled by ComCDE. Either comED or comC was knocked out, PcslAB activity decreased 34.66% and 17.64%, respectively but the leftovers were about 552.53 and 696.47 Miller units (Figure 3: “-” of PcslAB). Exogenous CSP partly rescued the defects of ΔcomED and ΔcomC because PcslAB activity was recovered about 48.39% in ΔcomED and 62.05% in ΔcomC against wild type (Figure 2: “+” of PcslAB).
PcslAB Partially Controlled by ComCDE
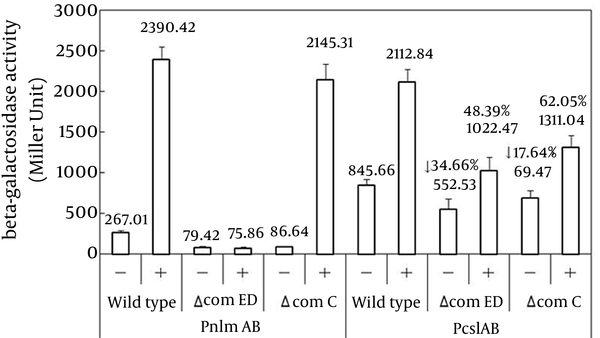
CslAB, a Stabilizer of ComCDE Signal on Its Expression
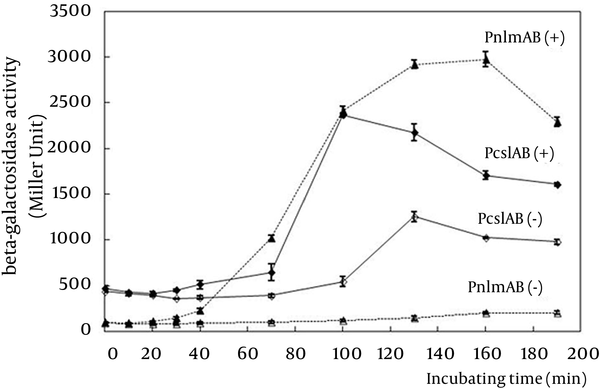
PcslAB and PnlmAB show that strains are harboring lacZ drove by PcslAB and PnlmAB. Wild type, ΔcomED and ΔcomC are strain genetic background (wild type, knock-out of comED and comC). “-” and “+” show that the strains are cultivated in the medium without exogenous CSP or with 1.0 μg/mL CSP. Bars and error bars represent the mean ± SD of results in triplicate experiments.
4.3. CslAB, a Stabilizer of ComCDE Signal on Expression of Itself
When S. mutans cells were cultivated in the medium without exogenous CSP, PcslAB activity curve was steeper than that of PnlmAB and the increasing activity rate was 8.98 Miller units/minute for PcslAB and 0.71 Miller units/minute for PnlmAB. Obviously, PcslAB more effectively drove cslAB transcription than PnlmAB did for nlmAB (Figure 3: “-” curves). When S. mutans cells were cultivated in the medium with 1.0 μg/mL CSP, the increasing activity rates were 24.38 Miller units/minute for PcslAB and 19.21 Miller units/minute for PnlmAB. In other words, PnlmAB activity increased 31.69 folds from 40th to 160th min (232.80 to 2974.94 Miller units), but PcslAB activity only increased 5.70 folds from 40th to 100th minutes (513.58 to 2365.39 Miller unit); PcslAB did not work as well as PnlmAB did (Figure 3: “+” curves). These data together suggested that cslAB expression was obviously buffered by the change of PcslAB activity.
PcslAB and PnlmAB activities in strains of WT/pAB-LacZ and WT/pA-LacZ are determined by β-galactosidase activity and are shown with solid and dash line. Samples from the medium with 1.0 μg/mL CSP and without exogenous CSP are marked with “+” and “-”. Promoter activity values and error bars represent the mean ± SD of results in triplicate experiments.
5. Discussion
ComCDE can be closed at its headstream because difunctional ComE can inhibit comC expression (9, 11, 12, 19), but cslAB transcription really offers ComCDE a more subtle mode to be regulated. Available data from Figure 1 show that PcslAB is a weak promoter responding to ComCDE and its binding with ComE is a negative cooperative reaction. We also know that the affinity of promoters of comC, cslAB, comED, and comX with ComE is decreasing in order and the binding of comC promoter with ComE is a strong positive cooperative reaction (9, 10, 12). Furthermore, PcslAB is incompletely controlled by ComCDE and can work in an independent way (Figures 2 and 3). This evidence indicates that ComE can take more tasks, which must be done by two TCSTSs at least in S. pneumonia.
Moreover, it shows that CslAB offers ComCDE a unique regulation pattern. When ComCDE signal is weak, just like wild type cells were cultivated in the medium without exogenous CSP, PcslAB could also provoke cslAB transcription to ensure CSP secretion (Figure 3). Obviously, PcslAB works in a mode independent of ComCDE (Figures 1 - 3). But when ComCDE signal is strong, as wild type cells were cultivated in the medium with 1.0 μg/mL CSP, PcslAB did not work as well as PnlmAB did, as PcslAB is a weak promoter responding to ComE (Figures 1 - 3). In this way, extracellular CSP gradient is maintained at a certain level, and all of these properties depend on PcslAB having a weak affinity with ComE and being partially controlled by ComCDE.
In S. pneumonia, the genes of competence development and bacteriocin production are organized in two regulons and their regulation starts by two separated primary signals (8, 14, 24, 25), but in S. mutans the same type of genes are packed into one regulon and the genes are differentially expressed relying on the interaction of their primary members (1-4, 9, 16, 26). Clearly, CslAB unique expression pattern helps maintain extracellular CSP gradient, even though its mechanism is not completely obvious. We believe that its mechanism probably involves the following issues. First, the extracellular CSP detection is complex. In the studies of biofilm formation and competence development, a second CSP receptor was suggested because extracellular CSP could not be blocked completely when ComED was abolished (1, 3). Secondly, the intracellular CSP export is also complicated. CslAB is a specific apparatus to secrete CSP, at the same time NlmTE is a specific apparatus to secrete nonlantibiotic mutacin, but in vitro the peptidase domain of NlmT (SMU.286) and ComA-like (SMU.1881c) can cleave ComCs from other Streptococcus spp. (16, 17, 27). Thirdly, ComE binding sites in the promoters of comC (142 bp), comED (321 bp), and cslAB (115 bp) are far from transcription start; it is possible that other unknown components control ComCDE signal (9-12, 18). Fourthly, ComCDE signal and other signal transduction are closely intertwined. The former reports showed that competence development in S. mutans proceeded in multilevel control involved in other two-component signal and acid induction signal transduction (6, 28). Finally, we want to say that maybe there are housekeeping regulation mechanisms to affect the transcription of ComCDE primary members as that PcslAB activity is independent of ComCDE.
Acknowledgements
References
-
1.
Li YH, Lau PC, Lee JH, Ellen RP, Cvitkovitch DG. Natural genetic transformation of Streptococcus mutans growing in biofilms. J Bacteriol. 2001;183(3):897-908. [PubMed ID: 11208787]. https://doi.org/10.1128/JB.183.3.897-908.2001.
-
2.
Li YH, Hanna MN, Svensater G, Ellen RP, Cvitkovitch DG. Cell density modulates acid adaptation in Streptococcus mutans: implications for survival in biofilms. J Bacteriol. 2001;183(23):6875-84. [PubMed ID: 11698377]. https://doi.org/10.1128/JB.183.23.6875-6884.2001.
-
3.
Li YH, Tang N, Aspiras MB, Lau PC, Lee JH, Ellen RP, et al. A quorum-sensing signaling system essential for genetic competence in Streptococcus mutans is involved in biofilm formation. J Bacteriol. 2002;184(10):2699-708. [PubMed ID: 11976299].
-
4.
Li YH, Lau PC, Tang N, Svensater G, Ellen RP, Cvitkovitch DG. Novel two-component regulatory system involved in biofilm formation and acid resistance in Streptococcus mutans. J Bacteriol. 2002;184(22):6333-42. [PubMed ID: 12399503].
-
5.
Aas JA, Paster BJ, Stokes LN, Olsen I, Dewhirst FE. Defining the normal bacterial flora of the oral cavity. J Clin Microbiol. 2005;43(11):5721-32. [PubMed ID: 16272510]. https://doi.org/10.1128/JCM.43.11.5721-5732.2005.
-
6.
Ahn SJ, Wen ZT, Burne RA. Multilevel control of competence development and stress tolerance in Streptococcus mutans UA159. Infect Immun. 2006;74(3):1631-42. [PubMed ID: 16495534]. https://doi.org/10.1128/IAI.74.3.1631-1642.2006.
-
7.
Kreth J, Merritt J, Shi W, Qi F. Co-ordinated bacteriocin production and competence development: a possible mechanism for taking up DNA from neighbouring species. Mol Microbiol. 2005;57(2):392-404. [PubMed ID: 15978073]. https://doi.org/10.1111/j.1365-2958.2005.04695.x.
-
8.
Martin B, Quentin Y, Fichant G, Claverys JP. Independent evolution of competence regulatory cascades in streptococci? Trends Microbiol. 2006;14(8):339-45. [PubMed ID: 16820295]. https://doi.org/10.1016/j.tim.2006.06.007.
-
9.
Hung DC-I. Characterization of Streptococcus mutans response regulator, ComE; Dissertation. Los Angeles, California: University Of Southern California; 2009.
-
10.
Hung DC, Downey JS, Kreth J, Qi F, Shi W, Cvitkovitch DG, et al. Oligomerization of the response regulator ComE from Streptococcus mutans is affected by phosphorylation. J Bacteriol. 2012;194(5):1127-35. [PubMed ID: 22210762]. https://doi.org/10.1128/JB.06565-11.
-
11.
Liu T, Xue S, Cai W, Liu X, Liu X, Zheng R, et al. ComCED signal loop precisely regulates nlmC expression in Streptococcus mutans. Ann Microbiol. 2013;64(1):31-8. https://doi.org/10.1007/s13213-013-0629-6.
-
12.
Hung DC, Downey JS, Ayala EA, Kreth J, Mair R, Senadheera DB, et al. Characterization of DNA binding sites of the ComE response regulator from Streptococcus mutans. J Bacteriol. 2011;193(14):3642-52. [PubMed ID: 21602345]. https://doi.org/10.1128/JB.00155-11.
-
13.
Ween O, Gaustad P, Havarstein LS. Identification of DNA binding sites for ComE, a key regulator of natural competence in Streptococcus pneumoniae. Mol Microbiol. 1999;33(4):817-27. [PubMed ID: 10447890].
-
14.
Claverys JP. Extracellular-peptide control of competence for genetic transformation in Streptococcus Pneumoniae. Frontiers Bioscie. 2002;7(1-3):d1798. https://doi.org/10.2741/claverys.
-
15.
van der Ploeg JR. Regulation of bacteriocin production in Streptococcus mutans by the quorum-sensing system required for development of genetic competence. J Bacteriol. 2005;187(12):3980-9. [PubMed ID: 15937160]. https://doi.org/10.1128/JB.187.12.3980-3989.2005.
-
16.
Petersen FC, Scheie AA. Genetic transformation in Streptococcus mutans requires a peptide secretion-like apparatus. Oral Microbiol Immunol. 2000;15(5):329-34. https://doi.org/10.1034/j.1399-302x.2000.150511.x.
-
17.
Hale JD, Heng NC, Jack RW, Tagg JR. Identification of nlmTE, the locus encoding the ABC transport system required for export of nonlantibiotic mutacins in Streptococcus mutans. J Bacteriol. 2005;187(14):5036-9. [PubMed ID: 15995224]. https://doi.org/10.1128/JB.187.14.5036-5039.2005.
-
18.
Kreth J, Hung DC, Merritt J, Perry J, Zhu L, Goodman SD, et al. The response regulator ComE in Streptococcus mutans functions both as a transcription activator of mutacin production and repressor of CSP biosynthesis. Microbiology. 2007;153(Pt 6):1799-807. [PubMed ID: 17526837]. https://doi.org/10.1099/mic.0.2007/005975-0.
-
19.
Hanahan D. Studies on transformation of Escherichia coli with plasmids. J Mol Biol. 1983;166(4):557-80. https://doi.org/10.1016/s0022-2836(83)80284-8.
-
20.
Ajdic D, McShan WM, McLaughlin RE, Savic G, Chang J, Carson MB, et al. Genome sequence of Streptococcus mutans UA159, a cariogenic dental pathogen. Proc Natl Acad Sci U S A. 2002;99(22):14434-9. [PubMed ID: 12397186]. https://doi.org/10.1073/pnas.172501299.
-
21.
Jing D, Agnew J, Patton WF, Hendrickson J, Beechem JM. A sensitive two-color electrophoretic mobility shift assay for detecting both nucleic acids and protein in gels. Proteomics. 2003;3(7):1172-80. [PubMed ID: 12872218]. https://doi.org/10.1002/pmic.200300438.
-
22.
Schuster M, Urbanowski ML, Greenberg EP. Promoter specificity in Pseudomonas aeruginosa quorum sensing revealed by DNA binding of purified LasR. Proc Natl Acad Sci U S A. 2004;101(45):15833-9. [PubMed ID: 15505212]. https://doi.org/10.1073/pnas.0407229101.
-
23.
Peruzzi F, Piggot PJ, Daneo-Moore L. Development of an integrative, lacZ transcriptional-fusion plasmid vector for Streptococcus mutans and its use to isolate expressed genes. Methods in Cell Science. 1998;20(1/4):153-63. https://doi.org/10.1023/a:1009826001367.
-
24.
Prudhomme M, Attaiech L, Sanchez G, Martin B, Claverys JP. Antibiotic stress induces genetic transformability in the human pathogen Streptococcus pneumoniae. Science. 2006;313(5783):89-92. [PubMed ID: 16825569]. https://doi.org/10.1126/science.1127912.
-
25.
Peterson SN, Sung CK, Cline R, Desai BV, Snesrud EC, Luo P, et al. Identification of competence pheromone responsive genes in Streptococcus pneumoniae by use of DNA microarrays. Mol Microbiol. 2004;51(4):1051-70. [PubMed ID: 14763980].
-
26.
Mandin P, Fsihi H, Dussurget O, Vergassola M, Milohanic E, Toledo-Arana A, et al. VirR, a response regulator critical for Listeria monocytogenes virulence. Mol Microbiol. 2005;57(5):1367-80. [PubMed ID: 16102006]. https://doi.org/10.1111/j.1365-2958.2005.04776.x.
-
27.
Kotake Y, Ishii S, Yano T, Katsuoka Y, Hayashi H. Substrate recognition mechanism of the peptidase domain of the quorum-sensing-signal-producing ABC transporter ComA from Streptococcus. Biochemistry. 2008;47(8):2531-8. [PubMed ID: 18232718]. https://doi.org/10.1021/bi702253n.
-
28.
Gong Y, Tian XL, Sutherland T, Sisson G, Mai J, Ling J, et al. Global transcriptional analysis of acid-inducible genes in Streptococcus mutans: multiple two-component systems involved in acid adaptation. Microbiology. 2009;155(Pt 10):3322-32. [PubMed ID: 19608608]. https://doi.org/10.1099/mic.0.031591-0.