Abstract
Background:
Staphylococcus aureus, with reduced vancomycin susceptibility, is probably under the regulation of several genes and various express phenotypes.Objectives:
This study aimed to investigate the phenotypic differences between vancomycin-susceptible S. aureus (VSSA), vancomycin-intermediate S. aureus (VISA), and heterogeneous VISA (hVISA) isolates.Materials and Methods:
A total of 130 methicillin-resistant S. aureus (MRSA) isolates were studied, including 49 VSSA, 28 hVISA, and 5 VISA isolates from blood cultures and 48 isolates (two VSSA, six hVISA, and 40 VISA) derived in vitro (laboratory-induced/sub-passaged). Their phenotypes were examined using a coagulase tube test, colony spreading on soft agar, and urease activity. The SCCmec and agr typing were performed using multiplex PCR.Results:
Most of the MRSA isolates were SCCmec III-agr I (84.5%), followed by SCCmec II-agr II (11.8%). The average plasma coagulation time of vancomycin-non-susceptible isolates was longer than that of the susceptible isolates (12 vs. 2.6 hours). Four hVISA (P = 0.023) and nine VISA (P < 0.001) isolates yielded a negative coagulase test after 24-hour incubation. The percentage of VSSA isolates showing non-spreading colonies (accessory gene regulator (agr) dysfunction) was significantly lower than in the VISA group (P = 0.013), but no significant difference was found between VSSA and hVISA. The VISA group showed higher urease activity than that of the VSSA and hVISA groups (P = 0.002).Conclusions:
There were diverse phenotypic changes among vancomycin-non-susceptible S. aureus isolates. This may be due to the variety of related regulatory systems. The diversity of phenotypic expression may result in its misidentification in routine laboratory checks.Keywords
Coagulase Urease Vancomycin Methicillin-Resistant Staphylococcus aureus
1. Background
Staphylococcus aureus causes various types of infection, and is therefore a serious public health concern. Problem of infections caused by methicillin-resistant S. aureus (MRSA) have been widely reported around the world (1). Vancomycin (a glycopeptide antibiotic) is the recommended therapy for serious infections caused by MRSA. Staphylococcus aureus with reduced susceptibility to vancomycin, including vancomycin-intermediate S. aureus (VISA) and heterogeneous VISA (hVISA), was eventually identified in Japan in 1997 (2, 3). These two strains were related to vancomycin treatment failures (4). The clinical and laboratory standards institute (CLSI; formerly NCCLS) redefined the vancomycin breakpoints, suggesting a susceptible, intermediate, or resistant interpretation when the vancomycin minimum inhibitory concentration (MIC) is ≤ 2 μg/mL, 4 to 8 μg/mL, or ≥ 16 μg/mL, respectively (5). The hVISA isolates cannot be detected using routine laboratory methods (i.e., disk diffusion and MICs determination) (6); rather, the reference procedure for hVISA detection is population analysis profiling with an area under the curve (PAP-AUC) (7). This procedure, however, is time-consuming, labor-intensive, costly, and unsuitable for most laboratories.
The phenotypic features and biochemical alterations of S. aureus with reduced vancomycin susceptibility have been reported. The alterations to the cell wall structure, leading to thickening, included: an increasing level of abnormal muropeptide production (8), an overexpression of penicillin-binding protein (PBP) 2 and PBP2a (9), increasing levels of D-alanyl-D-alanine residues, and reduction of peptidoglycan cross-link (10). The reduction of autolytic activity prevented the diffusion of vancomycin to its active site, increasing the level of resistance. In addition, 58% of hVISA isolates showed a loss of accessory gene regulator (agr) function (11), which is associated with autolytic activity reduction (12). Nelson et al. demonstrated that 8% of VSSA and 71% of VISA strains had reduced acetate catabolism, leading to an alteration of growth characteristics, toleration of antibiotics, cell death changes, and an increase in polysaccharide intercellular adhesion synthesis (13). These characteristic alterations may have an effect on bacterial identification in clinical microbiology laboratories.
2. Objectives
The main objective was to investigate the phenotypic characteristics of VSSA vs. vancomycin-non-susceptible strains, including coagulase activity, urease activity, and colony spreading. The basic genotypes (SCCmec and agr types) were also examined. This information would remind or alert clinical laboratories to identify Staphylococcus, especially the vancomycin-non-susceptible isolates. In addition, we observed the cell wall thicknesses of representative isogenic bacterial pairs.
3. Materials and Methods
3.1. Bacterial Isolates
A total of 130 MRSA isolates, including 82 isolates from Srinagarind hospital, Khon Kaen University, Thailand, and 48 isolates from laboratory induction, were studied. 33 isolates were randomly collected between October 1997 and November 1998, and 49 were collected between November 2010 and December 2011. 82 isolates (49 VSSA, 28 hVISA, and 5 VISA) were recovered from blood cultures, and 48 (2 VSSA, 6 hVISA, and 40 VISA) were derived in vitro (laboratory-induced/sub-passaged). All of the test isolates were identified by routine laboratory methods and confirmed by PCR-based detection of mecA and femA genes that are specific to MRSA (14). The isolates were preserved in skimmed milk, supplemented with 20% glycerol, at -20°C (15). The bacterial isolates of S. aureus were cultured overnight on blood agar (Oxoid, UK) at 37°C; a single colony was then subcultured for use in phenotypic tests. The isogenic set of isolates composed of the parent strain and its in vitro-induced/subpassaged strains with the same genetic background. The S. aureus reference strains were ATCC 29213 (VSSA), ATCC 700699 (VISA strain Mu50), and ATCC 700698 (hVISA strain Mu3). This study was conducted in accordance with the Helsinki declaration and was approved by the Khon Kaen University human research ethics committee (project number HE552272).
3.2. Laboratory Induction of Vancomycin-Non-Susceptible Staphylococcus aureus Isolates
The bacterial isolates were induced for vancomycin resistance by using a method modified from that of Pfeltz et al. (16). The isolates were cultured in tryptic soy broth (TSB) (Oxoid, UK) mixed with 2 μg/mL of vancomycin and shaken at 250 rpm in a 37°C water bath for one to seven days, or until turbid growth was observed. The cultures were then spread on tryptic soy agar (TSA) (Oxoid, UK) containing the same concentration of vancomycin as in the broth cultures. After 24-hour incubation, the mutant colony was re-cultured in TSB that was supplemented with a higher concentration of vancomycin (e.g. three, four, and five μg/mL vancomycin) and incubated under the same conditions for one to seven days. The cycles of broth and agar subcultures were repeated with increased concentrations of vancomycin in the media, until the concentration reached 16 μg/mL.
3.3. Modified Population Analysis Profile With Area Under the Curve (PAP-AUC)
Analyses of the subpopulation that was resistant to vancomycin were carried out using the modified PAP-AUC method (7). Overnight cultures of isolates in brain-heart infusion (BHI) broth (Oxoid, UK) were diluted serially in saline solutions from zero (undiluted) to 10-6. A 100-μL aliquot of each dilution was spread on BHI agar (Oxoid, UK) plates containing 0, 0.5, 1, 2, 3, 4, 5, 6, 7, and 8 μg/mL of vancomycin. The plates were incubated at 37°C for 48 hours before the colonies were counted. The log 10 CFU/mL of the bacterial colony counts were plotted vs. the vancomycin concentrations, and the area under the curve (AUC) was calculated using GraphPad Prism version 5.0.1 (GraphPad Software Inc., USA). The AUC ratio of the test isolate and that of the Mu3 strain were interpreted as follows: < 0.9, 0.9 to 1.3, and > 1.3 for the VSSA, hVISA, and VISA strains respectively. The hVISA strain Mu3 and the VSSA strain ATCC 29213 were tested simultaneously as positive and negative controls, respectively.
3.4. Antimicrobial Susceptibility Testing
The MICs of vancomycin (Sigma Chemical, USA) for all isolates were determined by the agar dilution method, as per the CLSI guideline (5).
3.5. Molecular Typing
The isolates’ DNA was extracted using achromopeptidase enzyme, according to the procedure described by Sasaki et al. (17). The supernatant was used as a DNA template for the PCR reaction. The MRSA isolates were analyzed by accessory gene regulator (agr) grouping according to Lina et al. (18), and the staphylococcal chromosome cassette mec (SCCmec) type was determined using a multiplex PCR as described by Kondo et al. (14).
3.6. Colony Spreading Assay
The presumptive test for function of the agr gene system was conducted in triplicate by colony spreading assay, using a method modified from Kaito et al. (19). Twenty mL of sterile TSB, supplemented with 0.24% agar (Becton and Dickinson, USA) was poured into a sterile petri dish. The plate was dried in a safety cabinet for 20 minutes. A 2-μL aliquot of the test isolate, of 0.5 McFarland turbidity, was spotted at the center of the plate. The plate was then dried in a safety cabinet for another 15 minutes and incubated overnight at 37°C (20). The isolates which showed giant colonies with branched arms were recorded as being positive for colony spreading.
3.7. Coagulase Tube Test
The coagulase tube test was performed in triplicate, as per Moreira et al. (9). Briefly, a loop of growth from fresh colonies on blood agar was suspended in 0.5 mL of plasma and incubated overnight at 37°C. The tubes were inspected for clot formation at 30-minute intervals for four hours, and again at 24 hours.
3.8. Urease Activity
Bacterial urease activity was determined in triplicate using the modified colorimetric assay of Hamilton-Miller and Gargan (21). Briefly, bacteria were cultured overnight at 37°C in a urea broth comprising 0.1 g/L of yeast extract, 9.1 g/L of KH2PO4, 9.5 g/L of Na2HPO4, 20 g/L of urea, and 0.01 g/L of phenol red. The cultures were then centrifuged at 5,000 ×g for three minutes, and the supernatants were transferred into a 96-well plate for measurement of the absorbance at 560 nm using a microplate reader (Tecan Sunrise, USA).
3.9. Transmission Electron Microscopy
Three sets of isogenic VSSA, hVISA, and VISA isolates were randomly selected for investigation of their cell wall thickness by transmission electron microscopy. The bacterial isolates were cultured overnight in TSB at 37°C. Each isolate was fixed overnight in Karnovsky’s fixative reagent, and was then rinsed four times in phosphate-buffered saline (PBS) for 15 minutes. The cells were then post-fixed for two hours in 1% OsO4, followed by washing twice in PBS for 15 minutes. The samples were dehydrated through a graded ethyl alcohol series, followed by infiltration and embedding in Epon 812. Ultrathin sections were cut from selected areas of the embedding samples and double-stained with uranyl acetate and lead citrate. The samples were examined with a transmission electron microscope (Zeiss EM10C, Germany) equipped with a Nikon DS-L2 camera (Nikon Corporation, USA), and digital micrograph software at 60 kV. Cell wall thickness was analyzed using Image J® version 1.49f (National Institute of Health, USA). Triplicate measurements of 30 equatorially cut cells were recorded from each strain and expressed as means ± standard deviations (SDs).
3.10. Statistical Analysis
All statistical analysis was conducted using MedCalc version 10.2.0.0 (MedCalc Software, Belgium). Comparisons of variables were analyzed using Fisher’s exact probability test. The independent and dependent samples t-test was used to compare the means between two unrelated groups, including urease activity and cell wall thicknesses. A P value of < 0.05 was considered as statistically significant.
4. Results
4.1. Bacterial Isolates
Of the 82 MRSA isolates from patients, 49 were VSSA, 28 were hVISA, and five were VISA, with PAP-AUC ratio ranges of 0.12 - 0.88, 0.9 - 1.26, and 1.35 - 1.91 respectively. Their vancomycin MICs ranges were 1 - 2, 1 - 3, and 3 - 4 μg/mL respectively. However, two VSSA isolates with vancomycin MICs of 2 - 3 μg/mL were received from the sub-passage experiments, and from the induction experiments, six hVISA and 40 VISA isolates were found with higher vancomycin MICs (3 - 16 and 3 - 16 μg/mL, respectively). Their PAP-AUC ratios ranged from 0.65 - 0.83, 0.99 - 1.27, and 1.35 - 3.91 respectively. The MIC50/90 values of all isolates are shown in Table 1.
Phenotypic Characteristics of VSSA, hVISA, and VISA Isolates
VSSA (n = 51) | hVISA (n = 34) | VISA (n = 45) | P Valuea | P Valueb | P Valuec | |
---|---|---|---|---|---|---|
PAP-AUC ratio, range (mean ± SD) | 0.12 - 0.88 (0.62 ± 0.16) | 0.90 - 1.27 (1.04 ± 0.12) | 1.35 - 3.91 (1.92 ± 0.66) | < 0.001d | < 0.001d | < 0.001d |
Vancomycin MIC range (MIC50/90), μg/mL | 1 - 2 (1/2) | 1 - 3 (1/2) | 2 - 16 (14/16) | 0.040d | < 0.001d | < 0.001d |
Coagulase negative after 24 hours incubation, No. (%) | 0 | 4 (11.8) | 9 (20) | 0.023e | < 0.001e | 0.375e |
agr-dysfunction by colony spreading assay, No. (%) | 15 (29.4) | 14 (41.2) | 25 (55.6) | 0.351e | 0.013e | 0.258e |
OD560 value of urease activity, Mean ± SD | 0.369 ± 0.25 | 0.345 ± 0.33 | 0.559 ± 0.32 | 0.701d | 0.002d | 0.005d |
4.2. Molecular Typing
Molecular typing was randomly performed in 50 VSSA, 20 hVISA, and 40 VISA isolates. Among the 50 VSSA isolates, 43 were SCCmec III-agr I, five were SCCmec II-agr II, one was SCCmec III-agr II, and one was SCCmec IX-agr II. Similarly, of the 20 hVISA isolates, 15 were SCCmec III-agr I, four were SCCmec II-agr II, and one was SCCmec III-agr II. Among the 40 VISA isolates, 35 were genotype SCCmec III-agr I, four were SCCmec II-agr II, and one was SCCmec II-agr I (Table 2). The isolates which were successful in increasing their vancomycin resistance were mostly of the genotypes SCCmec III-agr I, followed by SCCmec III-agr II and SCCmec II-agr II.
Isolates | agr Group | SCCmec Type | |||
---|---|---|---|---|---|
I | II | II | III | IX | |
VSSA (n = 50) | 43 (86) | 7 (14) | 5 (10) | 44 (88) | 1 (2) |
hVISA (n = 20) | 15 (75) | 5 (25) | 4 (20) | 16 (80) | NA |
VISA (n = 40) | 36 (90) | 4 (10) | 5 (12.5) | 35 (87.5) | NA |
4.3. Colony Spreading Assay
Since the intact agr system is one of the important regulatory systems for colony spreading, the ability to spread on soft agar plates was tested for presumptive evaluation of the presence of intact agr (Figure 1). Colony spreading was most predominant in VSSA isolates (36/51, 70.6%), followed by hVISA (20/34, 58.8%) and VISA (20/45, 44.4%). The prevalence of non-spreading colonies (assumed as agr dysfunction) among the VSSA isolates was significantly lower than that of the VISA isolates (P = 0.013) (Table 1). There was, however, no significant difference observed in colony spreading between the VSSA and hVISA isolates.
Colony Spreading of MRSA Isolates on Soft Agar Plates (Tryptic Soy Broth Plus 0.24% Agar) After Overnight Incubation at 37°C
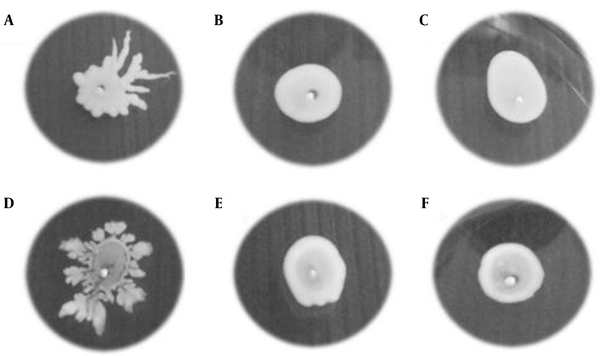
4.4. Coagulase Tube Test
All VSSA isolates yielded a positive coagulase test within one to 24 hours of incubation, whereas 4/34 of the hVISA (11.8%, three isolates being SCCmec III-agr I, one being SCCmec II-agr II) and 9/45 of the VISA isolates (20%, eight isolates being SCCmec III-agr I, one being SCCmec II-agr II) showed no clot formation after 24-hour incubation. These results were significantly different from those of the VSSA isolates (P = 0.023 and < 0.001, respectively) (Table 1). The average coagulation time of the isogenic vancomycin-non-susceptible isolates (n = 47) was significantly longer than that of its parent VSSA isolates (12 vs. 2.6 hours, P < 0.0001). In addition, all of the 13 isolates which showed no clot formation after 24-hour incubation also presented non-spreading colonies.
4.5. Urease Activity
The urease activity of the VSSA and hVISA isolates was quite low compared to that of the VISA isolates (P = 0.002 and 0.005, respectively). The ranges of OD560 of the VSSA, hVISA, and VISA isolates were 0.023 - 0.912, 0.013 - 0.981, and 0.020 - 0.989 respectively (mean OD560 ± SD, 0.369 ± 0.25, 0.345 ± 0.33, and 0.559 ± 0.32 respectively; Figure 2 and Table 1).
Urease Activity of Vancomycin-Susceptible (VSSA) and Vancomycin-Non-Susceptible Isolates (hVISA and VISA)
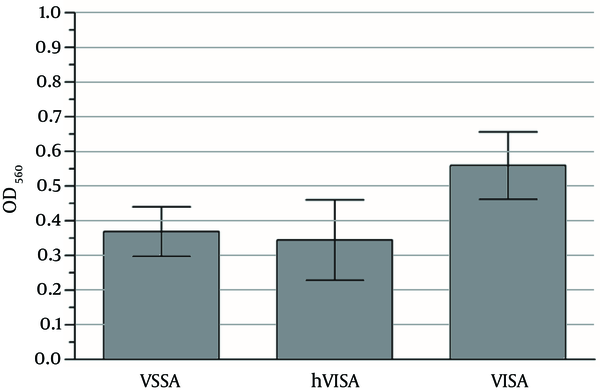
4.6. Transmission Electron Microscopy
A total of nine representative MRSA isolates (including three VSSA, three hVISA, and three VISA) underwent a morphometric study using transmission electron microscopy. The mean ± SDs of cell wall thickness of the 30 cells from each isolate are presented in Figure 3. The student’s t-test demonstrated that the cell wall thicknesses of hVISA and VISA isolates increased to be statistically significant for all samples (P < 0.001).
Transmission Electron Micrographs of Parent Vancomycin-Susceptible Isolates: VS-18, VS-57, VS-79, and Their Induced Heterogeneous Vancomycin-Intermediate (hVISA): hVI-18, hVI-57, hVI-79, and Induced Vancomycin-Intermediate Isolates (VISA): VI-18, VI-57, VI-79
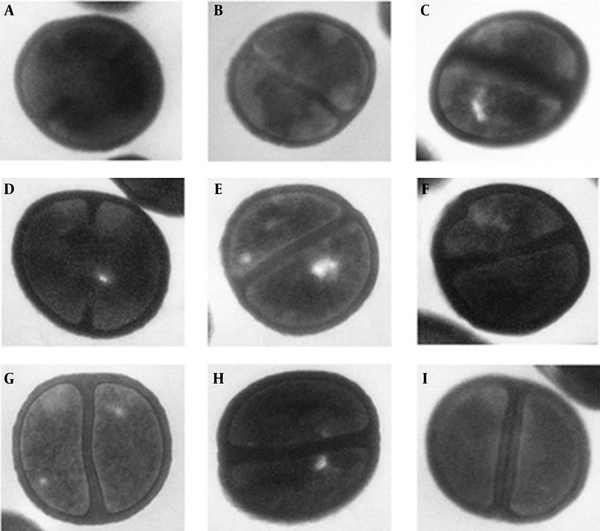
5. Discussion
Staphylococcus aureus with reduced susceptibility to vancomycin, including hVISA and VISA, mostly arises from MRSA. Previous studies have suggested that S. aureus isolates with reduced vancomycin susceptibility were more likely to harbor SCCmec II, which had been associated with increased mortality (22). Most of the MRSA isolates in this study were of the genotype SCCmec III-agr I, which has also been the most dominant nosocomial MRSA isolate in south-east Asia (23). However, diverse genotypes of MRSA isolates were able to be induced to hVISA and VISA. Likewise, the natural hVISA isolates from patients were of several genotypes: SCCmec III-agr I, SCCmec III-agr II, and SCCmec II-agr II. It has been reported that the S. aureus of all agr groups could develop to become VISA. In addition, when hVISA or VISA developed from VSSA, there was a reduction in levels of agr expression in the resistant isolates (24). The agr locus is a main quorum-sensing operon which regulates cell-to-cell signaling and also coordinates the expression of S. aureus virulence genes.
In this study, agr functions were primarily examined by colony-spreading assay which revealed that 55.6% of VISA and 41.2% of hVISA isolates displayed colony non-spreading, compared to 30% of VSSA isolates. In addition, other studies have demonstrated that 58% and 86.8% of hVISA strains had lost their agr function, which were higher percentages than those of the VSSA strains that had done so (11, 25). The colony-spreading of S. aureus is one of the bacterial virulence factors which require an interaction between the cell wall teichoic acids and the water in a soft agar surface (19). It was an agr-dependent expression (20), which might contribute to a synthesis of some of the molecules required for colony-spreading (26). The mechanism responsible for the reduced level of agr expression in these isolates, however, remains unclear; although S. aureus with reduced vancomycin susceptibility tended to lose agr function (10).
Park et al. reported a point mutation in the agr gene of some VISA strains, whereas the RNAIII levels of all VISA strains showed a marked decline when compared with the VSSA strains (27). This implied that there are several pathways that result in agr dysfunction. Coagulase production is another virulence factor whose function may be regulated by the agr operon; it is an important property used for routine identification of S. aureus. Decreases in coagulase activity in S. aureus with reduced glycopeptide susceptibility, however, can lead to misidentification (9). In this study, the hVISA and VISA strains demonstrated that they took longer to form clots than the VSSA strains. In addition, 11.8% of hVISA and 22.2% of VISA isolates showed no clot formation after 24-hour incubation. This may be due to the low expression of the agr gene or decreasing enzyme activity as a result of bacterial cell wall thickness (28). The thicker cell wall is presumed to have acted as a barrier, preventing the diffusion of vancomycin in cytoplasmic membrane through its active site (16). The essential prolonged time for coagulase in vancomycin-non-susceptible strains was consistent with an increase in PBP2 production (9).
At present, there is no simple screening technique or molecular-based testing for the detection of hVISA strains (1). The PAP-AUC method is generally considered the gold standard for confirmation of hVISA strains, albeit impractical for routine use. We therefore tested the potential of urease testing to identify hVISA and VISA strains in routine laboratory checks. S. aureus is particularly capable of using urea as a source of nitrogen for its growth. It produces urease, which hydrolyzes urea into ammonia and carbon dioxide (29). Interestingly, we found that the urease activity of VISA strains was different from that of hVISA and VSSA strains. This, however, is a preliminary study and additional sample sizes should be investigated in order to develop a practical screening method. If the bacteria could release a strongly alkaline agent such as ammonia, this may cause tissue damage or persistent infection (29). Therefore, reducing vancomycin susceptibility in S. aureus isolates may be a cause of poor outcomes, longer antibiotic treatment periods, treatment failure, and prolonged hospitalization (30).
Currently, the mechanism of low-level vancomycin resistance in S. aureus is not fully understood (1). The hypothesis for the resistance mechanism is that cell wall thickening prevents the diffusion of vancomycin (16). Our study supports this concept, as we observed that the hVISA and VISA strains had thicker cell walls than their parent VSSA strains. Cui et al. used mathematical modeling and suggested that cell wall thickening of hVISA and VISA resulted in a clogging phenomenon, which is an important resistance mechanism (31). Interestingly, the thickened cell wall appears to be a predominant feature, besides the increased production of abnormal muropeptides (8), overexpression of PBP2 and PBP2a (9), increase in D-alanyl-D-alanine residues, and reduction of peptidoglycan cross-linking (10). Cell wall biosynthesis pathways are disordered as a result of several mutations, leading to a loss of key enzyme functions (32). These relative characteristics make for interesting topics for further study, in order to elucidate the intrinsic resistant mechanisms.
In conclusion, reduced vancomycin susceptibility in S. aureus demonstrates several different phenotypic and biological changes, which may make identification difficult or result in misidentification of the isolates, leading to an underestimated prevalence. The current study suggests that the phenotypic changes in S. aureus with reduced vancomycin susceptibility are important and should be taken seriously.
Acknowledgements
References
-
1.
Howden BP, Davies JK, Johnson PD, Stinear TP, Grayson ML. Reduced vancomycin susceptibility in Staphylococcus aureus, including vancomycin-intermediate and heterogeneous vancomycin-intermediate strains: resistance mechanisms, laboratory detection, and clinical implications. Clin Microbiol Rev. 2010;23(1):99-139. [PubMed ID: 20065327]. https://doi.org/10.1128/CMR.00042-09.
-
2.
Hiramatsu K, Aritaka N, Hanaki H, Kawasaki S, Hosoda Y, Hori S, et al. Dissemination in Japanese hospitals of strains of Staphylococcus aureus heterogeneously resistant to vancomycin. Lancet. 1997;350(9092):1670-3. [PubMed ID: 9400512]. https://doi.org/10.1016/S0140-6736(97)07324-8.
-
3.
Hiramatsu K, Hanaki H, Ino T, Yabuta K, Oguri T, Tenover FC. Methicillin-resistant Staphylococcus aureus clinical strain with reduced vancomycin susceptibility. J Antimicrob Chemother. 1997;40(1):135-6. [PubMed ID: 9249217].
-
4.
Holmes NE, Johnson PD, Howden BP. Relationship between vancomycin-resistant Staphylococcus aureus, vancomycin-intermediate S. aureus, high vancomycin MIC, and outcome in serious S. aureus infections. J Clin Microbiol. 2012;50(8):2548-52. [PubMed ID: 22593595]. https://doi.org/10.1128/JCM.00775-12.
-
5.
Performance standards for antimicrobial susceptibility testing: Twenty-second informational supplement M100-S16. Wayne, USA: Clinical and Laboratory Standards Institute; 2012.
-
6.
Tenover FC, Biddle JW, Lancaster MV. Increasing resistance to vancomycin and other glycopeptides in Staphylococcus aureus. Emerg Infect Dis. 2001;7(2):327-32. [PubMed ID: 11294734]. https://doi.org/10.3201/eid0702.700327.
-
7.
Wootton M, Howe RA, Hillman R, Walsh TR, Bennett PM, MacGowan AP. A modified population analysis profile (PAP) method to detect hetero-resistance to vancomycin in Staphylococcus aureus in a UK hospital. J Antimicrob Chemother. 2001;47(4):399-403. [PubMed ID: 11266410].
-
8.
Sieradzki K, Tomasz A. Gradual alterations in cell wall structure and metabolism in vancomycin-resistant mutants of Staphylococcus aureus. J Bacteriol. 1999;181(24):7566-70. [PubMed ID: 10601215].
-
9.
Moreira B, Boyle-Vavra S, deJonge BL, Daum RS. Increased production of penicillin-binding protein 2, increased detection of other penicillin-binding proteins, and decreased coagulase activity associated with glycopeptide resistance in Staphylococcus aureus. Antimicrob Agents Chemother. 1997;41(8):1788-93. [PubMed ID: 9257762].
-
10.
Sieradzki K, Tomasz A. Alterations of cell wall structure and metabolism accompany reduced susceptibility to vancomycin in an isogenic series of clinical isolates of Staphylococcus aureus. J Bacteriol. 2003;185(24):7103-10. [PubMed ID: 14645269].
-
11.
Harigaya Y, Ngo D, Lesse AJ, Huang V, Tsuji BT. Characterization of heterogeneous vancomycin-intermediate resistance, MIC and accessory gene regulator (agr) dysfunction among clinical bloodstream isolates of staphyloccocus aureus. BMC Infect Dis. 2011;11:287. [PubMed ID: 22026752]. https://doi.org/10.1186/1471-2334-11-287.
-
12.
Sakoulas G, Eliopoulos GM, Moellering RC, Novick RP, Venkataraman L, Wennersten C, et al. Staphylococcus aureus accessory gene regulator (agr) group II: is there a relationship to the development of intermediate-level glycopeptide resistance? J Infect Dis. 2003;187(6):929-38. [PubMed ID: 12660939]. https://doi.org/10.1086/368128.
-
13.
Nelson JL, Rice KC, Slater SR, Fox PM, Archer GL, Bayles KW, et al. Vancomycin-intermediate Staphylococcus aureus strains have impaired acetate catabolism: implications for polysaccharide intercellular adhesin synthesis and autolysis. Antimicrob Agents Chemother. 2007;51(2):616-22. [PubMed ID: 17130298]. https://doi.org/10.1128/AAC.01057-06.
-
14.
Kondo Y, Ito T, Ma XX, Watanabe S, Kreiswirth BN, Etienne J, et al. Combination of multiplex PCRs for staphylococcal cassette chromosome mec type assignment: rapid identification system for mec, ccr, and major differences in junkyard regions. Antimicrob Agents Chemother. 2007;51(1):264-74. [PubMed ID: 17043114]. https://doi.org/10.1128/AAC.00165-06.
-
15.
Alsunaien T, Pratten J, Ng YL, Gulabivala K, Ready D. Effect of Transport and Storage Conditions on Recovery of Root Canal Isolates. Int Endod J. 2010;43(4):353.
-
16.
Pfeltz RF, Singh VK, Schmidt JL, Batten MA, Baranyk CS, Nadakavukaren MJ, et al. Characterization of passage-selected vancomycin-resistant Staphylococcus aureus strains of diverse parental backgrounds. Antimicrob Agents Chemother. 2000;44(2):294-303. [PubMed ID: 10639353].
-
17.
Sasaki T, Tsubakishita S, Tanaka Y, Sakusabe A, Ohtsuka M, Hirotaki S, et al. Multiplex-PCR method for species identification of coagulase-positive staphylococci. J Clin Microbiol. 2010;48(3):765-9. [PubMed ID: 20053855]. https://doi.org/10.1128/JCM.01232-09.
-
18.
Lina G, Boutite F, Tristan A, Bes M, Etienne J, Vandenesch F. Bacterial competition for human nasal cavity colonization: role of Staphylococcal agr alleles. Appl Environ Microbiol. 2003;69(1):18-23. [PubMed ID: 12513972].
-
19.
Kaito C, Sekimizu K. Colony spreading in Staphylococcus aureus. J Bacteriol. 2007;189(6):2553-7. [PubMed ID: 17194792]. https://doi.org/10.1128/JB.01635-06.
-
20.
Tsompanidou E, Sibbald MJ, Chlebowicz MA, Dreisbach A, Back JW, van Dijl JM, et al. Requirement of the agr locus for colony spreading of Staphylococcus aureus. J Bacteriol. 2011;193(5):1267-72. [PubMed ID: 21169484]. https://doi.org/10.1128/JB.01276-10.
-
21.
Hamilton-Miller JM, Gargan RA. Rapid screening for urease inhibitors. Invest Urol. 1979;16(5):327-8. [PubMed ID: 34578].
-
22.
Han JH, Edelstein PH, Lautenbach E. Reduced vancomycin susceptibility and staphylococcal cassette chromosome mec (SCCmec) type distribution in methicillin-resistant Staphylococcus aureus bacteraemia. J Antimicrob Chemother. 2012;67(10):2346-9. [PubMed ID: 22761330]. https://doi.org/10.1093/jac/dks255.
-
23.
Liu C, Chen ZJ, Sun Z, Feng X, Zou M, Cao W, et al. Molecular characteristics and virulence factors in methicillin-susceptible, resistant, and heterogeneous vancomycin-intermediate Staphylococcus aureus from central-southern China. J Microbiol Immunol Infect. 2015;48(5):490-6. [PubMed ID: 24767415]. https://doi.org/10.1016/j.jmii.2014.03.003.
-
24.
Jang HC, Kang SJ, Choi SM, Park KH, Shin JH, Choy HE, et al. Difference in agr dysfunction and reduced vancomycin susceptibility between MRSA bacteremia involving SCCmec types IV/IVa and I-III. PLoS One. 2012;7(11). eee49136. [PubMed ID: 23152862]. https://doi.org/10.1371/journal.pone.0049136.
-
25.
Hu J, Ma XX, Tian Y, Pang L, Cui LZ, Shang H. Reduced vancomycin susceptibility found in methicillin-resistant and methicillin-sensitive Staphylococcus aureus clinical isolates in Northeast China. PLoS One. 2013;8(9). eee73300. [PubMed ID: 24069184]. https://doi.org/10.1371/journal.pone.0073300.
-
26.
Ueda T, Kaito C, Omae Y, Sekimizu K. Sugar-responsive gene expression and the agr system are required for colony spreading in Staphylococcus aureus. Microb Pathog. 2011;51(3):178-85. [PubMed ID: 21514374]. https://doi.org/10.1016/j.micpath.2011.04.003.
-
27.
Park C, Shin NY, Byun JH, Shin HH, Kwon EY, Choi SM, et al. Downregulation of RNAIII in vancomycin-intermediate Staphylococcus aureus strains regardless of the presence of agr mutation. J Med Microbiol. 2012;61(Pt 3):345-52. [PubMed ID: 22016559]. https://doi.org/10.1099/jmm.0.035204-0.
-
28.
Dai Y, Zhou X, Ma X, Lu H, Li H. Misidentification of vancomycin-resistant Staphylococcus aureus as coagulase-negative Staphylococcus. J Med Microbiol. 2012;61(Pt 10):1454-8. [PubMed ID: 22723253]. https://doi.org/10.1099/jmm.0.045518-0.
-
29.
Derakhshan S, Sattari M, Bigdeli M. Effect of subinhibitory concentrations of cumin (Cuminum cyminum L.) seed essential oil and alcoholic extract on the morphology, capsule expression and urease activity of Klebsiella pneumoniae. Int J Antimicrob Agents. 2008;32(5):432-6. [PubMed ID: 18715764]. https://doi.org/10.1016/j.ijantimicag.2008.05.009.
-
30.
Fong RK, Low J, Koh TH, Kurup A. Clinical features and treatment outcomes of vancomycin-intermediate Staphylococcus aureus (VISA) and heteroresistant vancomycin-intermediate Staphylococcus aureus (hVISA) in a tertiary care institution in Singapore. Eur J Clin Microbiol Infect Dis. 2009;28(8):983-7. [PubMed ID: 19387707]. https://doi.org/10.1007/s10096-009-0741-5.
-
31.
Cui L, Iwamoto A, Lian JQ, Neoh HM, Maruyama T, Horikawa Y, et al. Novel mechanism of antibiotic resistance originating in vancomycin-intermediate Staphylococcus aureus. Antimicrob Agents Chemother. 2006;50(2):428-38. [PubMed ID: 16436693]. https://doi.org/10.1128/AAC.50.2.428-438.2006.
-
32.
Ruef C. Epidemiology and clinical impact of glycopeptide resistance in Staphylococcus aureus. Infection. 2004;32(6):315-27. [PubMed ID: 15597220]. https://doi.org/10.1007/s15010-004-4124-7.