Abstract
Background:
Fungal infections affect various parts of the body and can be difficult to treat. Aspergillus infection causes a spectrum of diverse diseases particularly in lung according to host immunity. The two major entities are invasive pulmonary aspergillosis and chronic pulmonary aspergillosis. Candida infections can be superficial or invasive. Superficial infections often affect the skin or mucous membranes. However, invasive fungal infections are often life-threatening. Advances in nanotechnology have opened new horizons in nanomedicine, allowing the synthesis of nanoparticles that can be assembled into complex architectures. Novel studies and technologies are devoted to understanding the mechanisms of disease for the design of new drugs.Objectives:
In the present study, the antifungal activity of biogenic selenium nanoparticles (Se NPs) against Aspergillus fumigatus and Candida albicans was investigated.Materials and Methods:
Se-reducing bacteria previously identified as Bacillus sp. MSh-1 were used for the intracellular biosynthesis of elemental Se NPs. The shape, size, and purity of the extracted NPs were determined with various instrumental techniques. The nanoparticles antifungal characterization mainly derives from the following pathways: (i) to generate sustained flux of nano-ions from the compounds that deposited on special substrates or imbedded in colloidal or semisolid matrices. (ii) To transport active those ions to sensitive targets on plasma membrane of fungi.Results:
The results of energy-dispersive X-ray demonstrated that the purified NPs consisted of only Se. In addition, transmission electron micrographs showed that 120- to 140-nm spherical Se NPs were the most common. An antifungal assay was performed with a standard Clinical and Laboratory Standards Institute broth microdilution method. Minimum inhibitory concentration (MIC) measurements of the antifungal activity of the Se NPs against C. albicans (70 μg/mL) and A. fumigatus (100 μg/mL) showed that yeast cells were more sensitive than mold cells.Conclusions:
The MICs against A. fumigatus (100 μg/mL) and C. albicans (70 μg/mL) showed that biogenic Se NPs are useful antifungal agents.Keywords
Selenium Nanoparticles Antifungal Activity Metal Nanoparticle Antifungal Agent Fumigatus Aspergillus fumigates Candida albicans
1. Background
Because pathogenic fungi have become resistant to current antifungal agents, the development of new antibiotics or novel antimicrobial formulations is essential. The antifungal capacities of various organic and inorganic materials against pathogenic fungi have been tested in recent decades and the development of such novel compounds is ongoing (1). Selenium (Se) is a micronutrient metalloid incorporated (in the form of selenomethionine or selenocysteine) in the structure of enzymes such as glutathione peroxidases, iodothyronine deiodinases, and thioredoxin reductase, which are involved in antioxidant defense, detoxification, and metabolism, respectively (2, 3). The low toxicity of Se nanoparticles (NPs) and properties such as antibacterial, antiviral, and antioxidant activities have attracted research attention (4, 5).
Nanotechnology allows the fabrication of novel compounds (i.e., NPs or nanoconjugates) with novel biological properties (6-9). Physicochemical techniques (10, 11), biological methods, and the synthesis of nanostructures with bacterial and fungal strains as well as several plant extracts offer novel, clean, non-toxic, and eco-friendly methods for the production of Se NPs (12, 13).
Although Se compounds such as selenium sulfide (SeS2) have long been used as antifungal agents (14), the antifungal activity of biogenic Se NPs against Aspergillus fumigatus and Candida albicans has not been investigated. In the present study, biogenic Se NPs were purified from the whole-cell lysate of Bacillus sp. MSh-1 and characterized. Then, the antifungal efficacy of these novel NPs against A. fumigatus and C. albicans was studied.
2. Objectives
The aim of this study was to synthesize Se NPs, test them against the yeast cells of C. albicans and mold cells of A. fumigatus, and compare their minimum inhibitory concentrations (MICs) to determine their sensitivities.
3. Materials and Methods
3.1. Chemicals
Selenium dioxide (SeO2), nutrient broth, potato dextrose agar, Sabouraud’s dextrose agar medium, 1-octanol, sodium dodecyl sulfate, and Tris-base were purchased from Merck Chemicals (Darmstadt, Germany). All other chemicals and solvents were of analytical grade.
3.2. Preparation of Biogenic Se NPs
Se NPs were prepared by reducing Se+4 ions with the Bacillus sp. MSh-1, which was previously identified with a 16S ribosomal DNA method (GenBank accession number: GU183144.1) (13). Briefly, sterile nutrient broth was supplemented with SeO2 (1.26 mM) and inoculated with an overnight colony of Bacillus sp. MSh-1 in a shaker incubator (30°C, 150 rpm) for 14 hours. Subsequently, cells containing elemental Se were isolated from the medium via centrifugation (4000 × g, 10 min). Cells were disrupted by using a mortar and pestle after washing with sterile normal saline and freezing with liquid nitrogen. One milliliter of disrupted cells containing Se NPs was dispersed in 2 mL 1-octanol, and the resulting two-phase system was centrifuged (5000 × g, 10 min). The biogenic Se NPs were settled and washed with chloroform, ethyl alcohol, and distilled water (13).
3.3. Characterization of Se NPs
After the Se NPs were sonicated (100 W, 5 minutes), transmission electron microscopy (TEM) images were recorded with a (Zeiss Supra 55 VP, Jena, Germany) equipped with an energy-dispersive X-ray (EDX) microanalyzer at an accelerating voltage of 100 kV. The size distribution pattern of the Se NPs was plotted by manually counting 400 individual particles from various TEM images.
3.4. Antifungal Activity
The antifungal activities of Se NPs against A. fumigatus (ATCC 5662) and C. albicans (ATCC 4862) were determined by using the National Committee for Laboratory Standards (CSLI) for yeasts (CSLI M27-A) and filamentous fungi (CSLI M38-P) approved for both macro- and microdilution methods (15, 16). A. fumigatus and C. albicans were cultured at 25°C in potato dextrose agar and Sabouraud’s dextrose agar medium, respectively. Preparations of 0.5 - 2.5 × 103 and 2 - 5 × 104 conidia/mL (final concentration) were obtained from A. fumigates and C. albicans, respectively, by using a standard neobar slide with the tubes containing 3 mL of sterile saline solution. RPMI 1640 medium containing l-glutamine and phenol red was buffered to pH 7.0 at 25°C with 0.165 mol/L 4-morpholinepropanesulfonic acid and used as the MIC method. Se NPs were suspended in sterile distilled water and subsequently diluted to a final concentration of 10 - 200 µg/mL with the medium according to the CLSI standard. The MICs of Se NPs capable of fungal growth inhibition after 48 hours of incubation at 37°C were determined. RPMI medium containing fungal suspension without Se NPs was used as negative control. Amphotericin B (AmB) (10 μg/mL) and nystatin (15 μg/mL) were used in the same medium as positive controls for A. fumigates and C. albicans, respectively. All antifungal experiments were repeated five times.
4. Results
Se NPs were successfully synthesized with Bacillus sp. MSh-1 as evidenced by a significant change in the cultivation medium from colorless to insoluble orange-red, indicating the presence of elemental Se (Se0), after 14 hours (Figure 1). The TEM images of the Se NPs (Figure 2) showed that these well-dispersed spherical nanostructures had diameters ranging from 80 to 220 nm. The EDX pattern of the biogenic NPs had a strong SeLα peak at 1.37 KeV (Figure 3). Furthermore, SeKα and SeKβ peaks were observed at 1.37 and 11.22 KeV, respectively (see Figure 3). Subsequently, no signals for other elements were observed in the EDX pattern, which showed only the presence of the aforementioned signals for Se atoms (with the weight percent equal to 100).
Cultivation of Bacillus sp. MSh-1 in nutrient broth (a) with and (b) without selenium dioxide
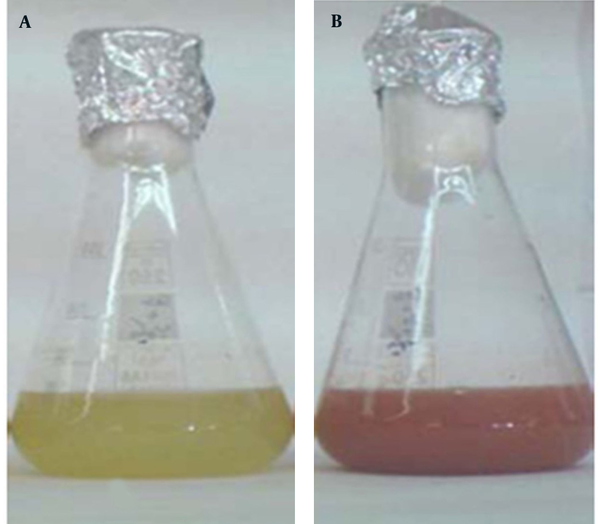
Transmission electron microscopy (TEM) images of selenium nanoparticles (Se NPs) synthesized by using Bacillus sp. MSh-1
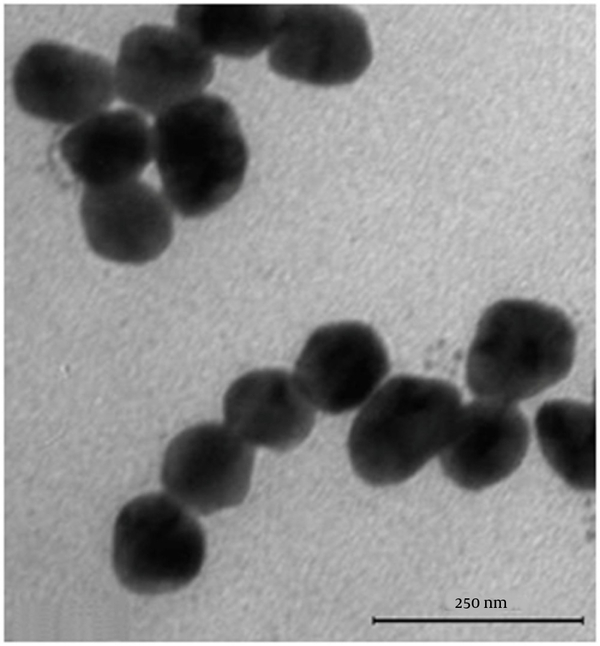
Energy-dispersive X-ray images of purified Se NPs
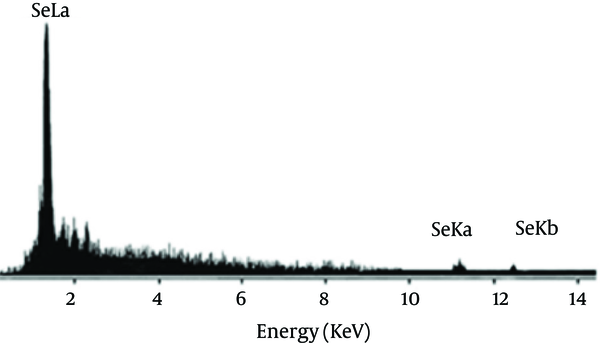
The size distribution pattern revealed that 120- to 140-nm NPs were the most common particles (Figure 4). The antifungal activities of the Se NPs against A. fumigatus and C. albicans are shown in Table 1. The measured MICs for A. fumigatus (100 μg/mL) and C. albicans (70 μg/mL) showed that the biogenic Se NPs had good anti-fungal activity.
Particle size distribution histogram of the biogenic Se NPs obtained by manually counting 400 individual particles from various TEM images
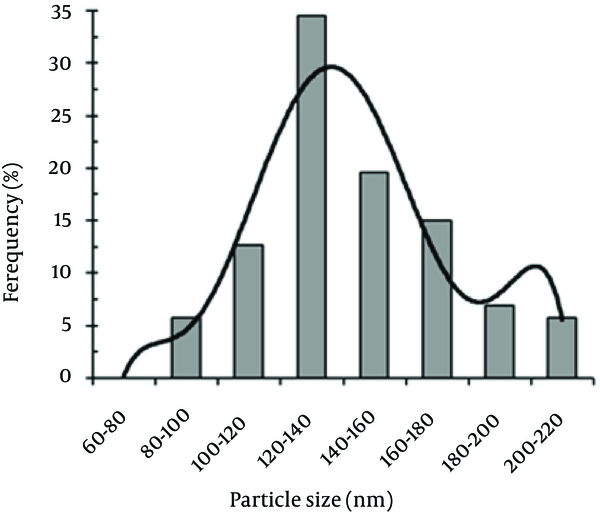
Antifungal Activities of Biogenic Selenium Nanoparticles (Se NPs) against Aspergillus fumigatus and Candida albicans
Fungal Strain | Antifungal Activity of Se NPs MIC a | Active Antifungal Drug MIC |
---|---|---|
Aspergillus fumigatus | 100 | Amphotripcin B 1 |
Candida albicans | 70 | Nystatin 0.05 |
5. Discussion
Because nystatin resistance in Candida sp. has been reported, especially in immunocompromised patients, and amphotericin B is still widely used as a first-line drug for the treatment of invasive aspergillosis despite its low efficacy, the development of new agents that can be used against fungi is critical. Ramamurthy et al. used fenugreek seed extract for the biosynthesis of Se NPs (50 - 150 nm) after 72 hour incubation (17). In another study, supplementation of the culture supernatant of A. terreus with SeO2 (100 μg/mL) produced Se NPs with an average size of 47 nm (18, 19). Bacillusmegaterium (a halophile strain) strongly reduced selenite (up to 0.25 mM) to Se NPs after 40 hours of incubation (17). Our results showed that the Bacillus sp. MSh-1 synthesized Se NPs (80 - 220 nm) after 14 hours of incubation.
The resistances of Candida sp. to nystatin has been reported, especially in immunocompromised patients (20). Amphotericin B is still widely used as first-line drug for treatment of invasive aspergillosis despite its lower efficacy (21, 22). Despite the development of new antifungal drugs, the number and variety of effective treatments remain limited (23). Therefore, use of NPs against pathogenic fungi is yet another novel approach. Se NPs have a variety of biological properties and might be candidates for a range of applications (24-26). Kazempour et al. (27) reported that the MICs of Se NPs (90 - 320 nm) biosynthesized with a two-phase system by using Klebsiella pneumoniae and without purification against A. niger and C. albicans were 250 µg/mL and 2000 µg/mL, respectively. The results of another study of biogenic Se NPs (100 - 600 nm) showed the highest antifungal activity against Malassezia sympodialis (MIC 10 µg/mL) and M. furfur (MIC 50 µg/mL) (28). Furthermore, the measured MICs of nanoselenium against A. niger, A. terreus, A. flavus, A. fumigatus were 260 µg/mL, 60 µg/mL, 220 µg/mL, and 100 µg/mL, respectively, against AmB (28). Although our Se NPs (80 - 220 nm) were smaller than the Se NPs (100 - 600 nm) prepared by using K. pneumoniae, they showed the same levels of antifungal activity against A. fumigatus.
The results of the present study suggest that nanoscale biogenic elemental Se has antifungal activity against A. fumigatus and C. albicans. The mechanism of this antifungal effect is unknown and merits further in vivo and in vitro studies.
Acknowledgements
References
-
1.
Rai M, Yadav A, Gade A. Silver nanoparticles as a new generation of antimicrobials. Biotechnol Adv. 2009;27(1):76-83. [PubMed ID: 18854209]. https://doi.org/10.1016/j.biotechadv.2008.09.002.
-
2.
Forootanfar H, Adeli-Sardou M, Nikkhoo M, Mehrabani M, Amir-Heidari B, Shahverdi AR, et al. Antioxidant and cytotoxic effect of biologically synthesized selenium nanoparticles in comparison to selenium dioxide. J Trace Elem Med Biol. 2014;28(1):75-9. [PubMed ID: 24074651]. https://doi.org/10.1016/j.jtemb.2013.07.005.
-
3.
Messarah M, Klibet F, Boumendjel A, Abdennour C, Bouzerna N, Boulakoud MS, et al. Hepatoprotective role and antioxidant capacity of selenium on arsenic-induced liver injury in rats. Exp Toxicol Pathol. 2012;64(3):167-74. [PubMed ID: 20851583]. https://doi.org/10.1016/j.etp.2010.08.002.
-
4.
Beheshti N, Soflaei S, Shakibaie M, Yazdi MH, Ghaffarifar F, Dalimi A, et al. Efficacy of biogenic selenium nanoparticles against Leishmania major: in vitro and in vivo studies. J Trace Elem Med Biol. 2013;27(3):203-7. [PubMed ID: 23219368]. https://doi.org/10.1016/j.jtemb.2012.11.002.
-
5.
Shakibaie M, Shahverdi AR, Faramarzi MA, Hassanzadeh GR, Rahimi HR, Sabzevari O. Acute and subacute toxicity of novel biogenic selenium nanoparticles in mice. Pharm Biol. 2013;51(1):58-63. [PubMed ID: 23035822]. https://doi.org/10.3109/13880209.2012.710241.
-
6.
El-Sayed MA. Some interesting properties of metals confined in time and nanometer space of different shapes. Acc Chem Res. 2001;34(4):257-64. [PubMed ID: 11308299].
-
7.
McConnell WP, Novak JP, Brousseau LC, Fuierer RR, Tenent RC, Feldheim DL. Electronic and optical properties of chemically modified metal nanoparticles and molecularly bridged nanoparticle arrays. J Phys Chem B. 2000;104(38):8925-30.
-
8.
Binupriya AR, Sathishkumar M, Vijayaraghavan K, Yun SI. Bioreduction of trivalent aurum to nano-crystalline gold particles by active and inactive cells and cell-free extract of Aspergillus oryzae var. viridis. J Hazard Mater. 2010;177(1-3):539-45. [PubMed ID: 20056324]. https://doi.org/10.1016/j.jhazmat.2009.12.066.
-
9.
Moore MN. Do nanoparticles present ecotoxicological risks for the health of the aquatic environment? Environ Int. 2006;32(8):967-76. [PubMed ID: 16859745]. https://doi.org/10.1016/j.envint.2006.06.014.
-
10.
Quintana M, Haro Poniatowski E, Morales J, Batina N. Synthesis of selenium nanoparticles by pulsed laser ablation. Appl Surf Sci. 2002;195(1-4):175-86. https://doi.org/10.1016/s0169-4332(02)00549-4.
-
11.
Langi B, Shah C, Singh K, Chaskar A, Kumar MS, Bajaj PN. Ionic liquid-induced synthesis of selenium nanoparticles. Mater Res Bull. 2010;45(6):668-71. https://doi.org/10.1016/j.materresbull.2010.03.005.
-
12.
Shakibaie M, Khorramizadeh MR, Faramarzi MA, Sabzevari O, Shahverdi AR. Biosynthesis and recovery of selenium nanoparticles and the effects on matrix metalloproteinase-2 expression. Biotechnol Appl Biochem. 2010;56(1):7-15. [PubMed ID: 20408816]. https://doi.org/10.1042/BA20100042.
-
13.
Fesharaki PJ, Nazari P, Shakibaie M, Rezaie S, Banoee M, Abdollahi M, et al. Biosynthesis of selenium nanoparticles using Klebsiella pneumoniae and their recovery by a simple sterilization process. Braz J Microbiol. 2010;41(2):461-6. [PubMed ID: 24031517]. https://doi.org/10.1590/S1517-838220100002000028.
-
14.
Aggarwal K, Jain VK, Sangwan S. Comparative study of ketoconazole versus selenium sulphide shampoo in pityriasis versicolor. Indian J Dermatol Venereol Leprol. 2003;69(2):86-7. [PubMed ID: 17642841].
-
15.
CSLIdocument M27-A. Reference method for broth dilution antifungal susceptibility testing of yeasts. Clin Lab Standards Inst. 1977;17(9):1-29.
-
16.
CSLI document M38-P. Reference method for broth dilution antifungal susceptibility testing of conditionforming filamentous fungi. Clin Lab Standards Inst. 1998;22(16):1-29.
-
17.
Ramamurthy C, Sampath KS, Arunkumar P, Kumar MS, Sujatha V, Premkumar K, et al. Green synthesis and characterization of selenium nanoparticles and its augmented cytotoxicity with doxorubicin on cancer cells. Bioprocess Biosyst Eng. 2013;36(8):1131-9. [PubMed ID: 23446776]. https://doi.org/10.1007/s00449-012-0867-1.
-
18.
Mishra RR, Prajapati S, Das J, Dangar TK, Das N, Thatoi H. Reduction of selenite to red elemental selenium by moderately halotolerant Bacillus megaterium strains isolated from Bhitarkanika mangrove soil and characterization of reduced product. Chemosphere. 2011;84(9):1231-7. [PubMed ID: 21664643]. https://doi.org/10.1016/j.chemosphere.2011.05.025.
-
19.
Zare B, Babaie S, Setayesh N, Shahverdi AR. Isolation and characterization of a fungus for extracellular synthesis of small selenium nanoparticles. Nanomed J. 2013;1(1):13-9.
-
20.
Baddley JW, Stroud TP, Salzman D, Pappas PG. Invasive mold infections in allogeneic bone marrow transplant recipients. Clin Infect Dis. 2001;32(9):1319-24. [PubMed ID: 11303267]. https://doi.org/10.1086/319985.
-
21.
Frampton JE, Scott LJ. Posaconazole. Drugs. 2008;68(7):993-1016. https://doi.org/10.2165/00003495-200868070-00008.
-
22.
Rickerts V, Bohme A, Viertel A, Behrendt G, Jacobi V, Tintelnot K, et al. Cluster of pulmonary infections caused by Cunninghamella bertholletiae in immunocompromised patients. Clin Infect Dis. 2000;31(4):910-3. [PubMed ID: 11049769]. https://doi.org/10.1086/318144.
-
23.
Rosato A, Vitali C, Piarulli M, Mazzotta M, Argentieri MP, Mallamaci R. In vitro synergic efficacy of the combination of Nystatin with the essential oils of Origanum vulgare and Pelargonium graveolens against some Candida species. Phytomedicine. 2009;16(10):972-5. [PubMed ID: 19616925]. https://doi.org/10.1016/j.phymed.2009.02.011.
-
24.
Chen T, Wong YS, Zheng W, Bai Y, Huang L. Selenium nanoparticles fabricated in Undaria pinnatifida polysaccharide solutions induce mitochondria-mediated apoptosis in A375 human melanoma cells. Colloids Surf B Biointerfaces. 2008;67(1):26-31. [PubMed ID: 18805679]. https://doi.org/10.1016/j.colsurfb.2008.07.010.
-
25.
Yazdi MH, Mahdavi M, Varastehmoradi B, Faramarzi MA, Shahverdi AR. The immunostimulatory effect of biogenic selenium nanoparticles on the 4T1 breast cancer model: an in vivo study. Biol Trace Elem Res. 2012;149(1):22-8. [PubMed ID: 22476951]. https://doi.org/10.1007/s12011-012-9402-0.
-
26.
Zhang JS, Gao XY, Zhang LD, Bao YP. Biological effects of a nano red elemental selenium. BioFactors. 2001;15(1):27-38. https://doi.org/10.1002/biof.5520150103.
-
27.
Kazempour ZB, Yazdi MH, Rafii F, Shahverdi AR. Sub-inhibitory concentration of biogenic selenium nanoparticles lacks post antifungal effect for Aspergillus niger and Candida albicans and stimulates the growth of Aspergillus niger. Iran J Microbiol. 2013;5(1):81-5. [PubMed ID: 23466957].
-
28.
Shahverdi AR, Fakhimi A, Mosavat G, Jafari Fesharaki P, Rezaie S, Rezayat SM. Antifungal activity of biogenic selenium nanoparticles. World Appl Sci J. 2010;10(8):918-22.