Abstract
Background:
Listeria monocytogenes is one of the most virulent types of bacteria and causes severe foodborne illness, such as listeriosis. Because this pathogen has become resistant to sanitizers and other disinfectants that are used to clean utensils and surfaces during food processing, it poses a serious threat to the food industry.Objectives:
The study was conducted to determine the anti-listerial potential of essential oils extracted from four edible seaweeds against L. monocytogenes.Materials and Methods:
Essential oil was extracted from four edible seaweeds (Enteromorpha linza, Undaria pinnatifida, Laminaria japonica, and Porphyra tenera) against L. monocytogenes using the microwave hydrodistillation method. The anti-listerial activity of the essential oil was determined using the standard disc diffusion method.Results:
Among the four essential oils, E. linza (ELEO) was most effective against all three strains of L. monocytogenes (11.3 - 16.0 mm). The other three essential oils were only effective against two strains, L. monocytogenes ATCC 19115 (10.0 - 10.5 mm) and L. monocytogenes ATCC 7644 (11.0 - 15.0 mm). The minimum inhibitory concentration and the minimum bactericidal concentration of all four essential oils varied from 12.5 - 25.0 mg/mL. Further, the mode of action of ELEO against L. monocytogenes was investigated by examining its effect on cell viability, the release of 260-nm absorbing materials, the number of K+ ions, the relative electrical conductivity, and the salt tolerance capacity. The results indicated that the essential oils exhibited strong anti-listerial activity against multiple strains of L. monocytogenes. It displayed potential inhibitory effects on the viability of bacterial cells and loss of integrity as indicated by an increase in the relative electrical conductivity, leakage of K+ ions and other 260-nm absorbing materials, and a loss of the salt tolerance capacity.Conclusions:
The results presented herein provided insight into a possible explanation for the modes of action of essential oils on L. monocytogenes. The outcome of the present study may aid the food industry in locating the most promising potential anti-listerial agents from edible seaweed sources to control L. monocytogenes and also in facilitating their application in food processing and preservation techniques in a nontoxic and environmental friendly manner.Keywords
Antibacterial Agents Cell Membrane Permeability Essential Oil Salt-Tolerant
1. Background
Millions of people throughout the world are affected by foodborne diseases each year (1, 2). Although many improvements in maintaining food hygiene and food production techniques have been achieved in recent years, the safety of the food supply is still one of the most important public health issues worldwide (2). The problems of food spoilage and food poisoning due to the harmful effects of various microorganisms introduced during the production and preservation of food are still a concern to both the food industry sector and consumers (3). In addition to the development of a variety of preservation methods, many synthetic chemical additives have been adopted by the food industries (4-6); however, the side effects of some of these synthetic chemicals have negatively impacted their potential usage (3). Thus, the identification of the root causes of food-related illnesses and also the development of a strong effective mechanism for their complete eradication is one of the major concerns for the food processing and food preservation industries.
It is well known that foodborne illnesses primarily result from the consumption of foods contaminated with different types of pathogenic bacteria. Listeria monocytogenes in particular is one of the most virulent Gram-positive bacteria that can produce severe foodborne illness, such as listeriosis (7, 8). It contaminates almost all types of food products because it can easily grow at temperatures ranging from 4 - 50°C and at a pH between 4 - 10 (9, 10). The onset of listeriosis is normally marked by the presence of flulike symptoms, which become serious within few days to three weeks (7).
Listeriosis is especially dangerous for people with suppressed immune systems, such as the elderly, acquired immunodeficiency syndrome (AIDS) patients, and pregnant women (10-12). This disease causes serious inflammation in the brain and spinal cord of premature and newborn babies and even in some people older than 50 years of age (meningitis, encephalitis, and perinatal infections) (7). In pregnant women, listeriosis can lead to spontaneous abortion and intrauterine or cervical infections (7, 11, 13). This disease is typically transmitted through certain foods, including dairy products, processed meat, eggs, poultry, fish, seafood, fruit, vegetables, and salad (13, 14). It is very difficult to control listeria infections because listeria is ubiquitous and widespread in the environment and can survive and multiply even at refrigeration temperatures (7, 15).
Most food processing equipment, utensils, and surfaces are made of stainless steel, polypropylene, polystyrene, or polyvinyl chloride, which are the most common surfaces for L. monocytogenes attachment and biofilm formation. This pathogen has become resistant to sanitizers and various disinfectants that are used to clean utensils and preparation surfaces as well as to the equipment used during food processing (9, 16). Increased consumer demand for food safety has forced the food industry to search for alternatives to synthetic chemicals and preservatives. Therefore, it is essential to identify strong natural antibacterial agents that can act effectively against multiple strains of L. monocytogenes and also serve as environmentally friendly and nontoxic compounds for their potential use by the food industry.
Natural products from the sea, particularly marine algae and seaweeds, have become an important part of the human diet (17). Since ancient times, seaweeds have long been used as food in most parts of the world, especially in eastern and northern Asia, including Korea, Japan, and China (18-23). Many ingredients from these seaweeds are used in drinks, soups and snacks (17). A number of chemical compounds extracted from marine seaweeds, such as alginates, agar, and carrageenan, as well as pigments like beta-carotene, have been widely used by the food and pharmaceutical industries (17). Similarly, plant-derived volatile essential oils (EOs) that possess variable mixtures of essential terpenoids, aliphatic hydrocarbons, acids, alcohols, aldehydes, and phenolic compounds, have also been used as flavoring agents by many food industries (2, 24, 25).
A number of seaweeds and plants possess various biological activities, such as antifungal, antiviral, antioxidant, anticoagulant, and anti-ulcer properties (2, 3, 8, 13, 17, 26-30). Recently, much research has been conducted to identify natural antibacterial bioactive compounds and agents from terrestrial, marine, and aquatic plants and their products (1-3). In this context, EOs from different sources have been tested for their antibacterial properties against a broad range of foodborne pathogenic bacteria (2, 3, 13), although the search for new antibacterial agents is still ongoing.
2. Objectives
In the present study, we extracted EOs from four edible seaweeds (E. linza (L.) J. Ag., U. pinnatifida (Harvey), L. japonica (J.E. Areschoug), and P. tenera (Kjellman)) and evaluated them for their antilisterial potentials against three different strains of L. monocytogenes, as well as for their mechanism of action.
3. Materials and Methods
3.1. Extraction of Essential oil From Seaweeds
Four different edible seaweeds (E. linza (fresh), U. pinnatifida (fresh), L. japonica (dry), and P. tenera (dry)) were purchased from a local market in Gyeongsan, Republic of Korea, and used for the extraction of the EOs. Each sample (500 g) was collected in a specially designed glass container with 5l of water and subjected to hydro-distillation for 4 hours using a microwave-assisted extraction apparatus manufactured by KMD Engineering (KMD Engineering Co., Ltd., Paju, Republic of Korea) for the extraction of the EOs. The operating condition of the machine was maintained by an oven power capacity of 40 W and a frequency of 15 gkH to control the temperature. A conical flask was connected to the collecting nozzle to collect the distillate. Equal volumes of dichloromethane were added to the collected distillate in a separating funnel and then vigorously shaken and kept until the two layers separated. The lower of the two layers was collected from the separating funnel and concentrated using a rotary evaporator (N-1110, Eyela, Tokyo Rikakikai Co., Ltd., Japan) at 40°C. The same procedure was followed for all four seaweed samples, after which the collected, yellow-colored EO samples were dried over anhydrous sodium sulfate and kept in tightly closed vials at 4°C until further use.
3.2. Screening of the Anti-Listerial Potential of the EOs
The anti-listerial activities of EOs from four different edible seaweeds were evaluated against three different strains of L. monocytogenes (ATCC 7644, ATCC 19115, and ATCC 19114). All strains were obtained from the American Type Culture Collection (ATCC, Manassas, VA, USA) and maintained in nutrient broth (NB) (Difco, Becton, Dickinson and Company, Sparks Glencoe, MD, USA) media until their use, at which time all four EOs were diluted twice in 5% dimethyl sulfoxide (DMSO) and filter-sterilized using a 0.22-µm nylon syringe filter. The antibacterial activity was subsequently evaluated using a standard disc diffusion assay (31). Briefly, sterile filter paper discs (Advantec, Toyo Roshi Kaisha Ltd., Japan) 6 mm in diameter were used to prepare different antibacterial discs by adding one of the four EOs (25 mg/disc) or the standard antibiotic kanamycin (40 µg/disc, Sigma-Aldrich Co., St. Louis, MO, USA), respectively. DMSO was the negative control. The assay used a culture of each strain that had been grown overnight at a concentration of 107 colony forming units (CFUs)/ml in NB. The experiment was repeated three times, and the mean of the diameter of the zone of inhibition against each strain was recorded after 24 h of incubation at 37°C.
3.3. Determination of the MIC and MBC
The minimum inhibitory concentration (MIC) and the minimum bactericidal concentration (MBC) of the four different EOs against the three strains of L. monocytogenes were determined with the two-fold dilution method (32). The lowest concentration of each EO that showed no visible growth of the tested strains was selected as the MIC (mg/mL), while the concentrations of each EO that exhibited no signs of any bacterial colony growth on nutrient agar (NA) plates were considered the MBC (mg/mL).
3.4. Anti-Listerial mode of action of E. linza EO (ELEO) on L. monocytogenes
3.4.1. Time kill Assay
The effects of ELEO on the Viability of L. monocytogenes ATCC 19115 were studied using a time kill assay (33). A bacterial culture treated with ELEO at the MIC (12.5 mg/mL) or with 5% DMSO was taken as the treatment sample and the control, respectively. The cultures were incubated at 37°C for 8 hours, with the samples collected at 2 h intervals and appropriately diluted in phosphate buffered saline at a pH of 7.4. The diluted samples were then spread over the NA plates and incubated at 37°C for 24 hours. Bacterial colonies in both the control and the treatment plates were counted as CFUs and expressed in Log10 (CFU/mL).
3.4.2. Cell Membrane Permeability
The membrane permeability of L. monocytogenes ATCC 19115 due to the effects of ELEO was evaluated by the following standard procedure (34). The loss in the permeability of the bacterial membrane was indirectly assessed by the release of ions into the supernatant of the bacterial solution using a conductivity meter (Con 6, LaMotte, MD, USA). The cell membrane permeability was determined by calculating the percentage of the relative electrical conductivity using the following equation:

where L0 was the electrical conductivity of dead L. monocytogenes ATCC 19115 in 5% glucose after treatment in boiling water for 5 minutes; L1 was the electrical conductivity of ELEO at the MIC added to 5% glucose; and L2 was the electrical conductivity of the bacteria treated with ELEO at 2-h intervals during 8 h of incubation.
3.4.3. Release of 260-nm Absorbing Materials
The release of 260 nm absorbing materials from L. monocytogenes ATCC 19115 treated with ELEO was measured using the standard procedure described by Carson et al. (35). Bacterial samples treated with 5% DMSO were taken as the control, while samples treated with the MIC of ELEO were considered to be the treatment. Both the control and treated samples were incubated at 37°C, during which time they were taken out every 30 minutes and centrifuged at 3,500 rpm for 10 minutes, after which the absorbance of the supernatant at 260 nm was measured using a spectrophotometer (ASP 3700, ACTGene Inc., NJ, USA). The results were expressed in terms of their optical density at 260 nm for each interval with respect to time. Corrections for the absorbance of the treated cultures were made by determining the values of ELEO without bacteria in peptone water (0.1% w/v). The untreated bacterial cultures were corrected using peptone water (0.1%).
3.4.4. Leakage of Potassium Ions
The amount of free potassium (K+) ions that leaked from L. monocytogenes ATCC 19115 was determined as per the standard procedure of described by Bajpai et al. (36). The concentration of free K+ ions in the suspension of L. monocytogenes ATCC 19115 was measured after exposing the cells to the MIC of ELEO in peptone water (0.1%) and then incubating them at 37°C for 8 hours. The extracellular potassium concentration was finally measured every 2 hours using a Kalium/Potassium kit (Quantofix, Macherey-Nagel GmbH and Co., Germany). The control consisted of L. monocytogenes ATCC 19115 treated with 5% DMSO.
3.4.5. Loss of Salt Tolerance Capacity
The loss of the salt tolerance capacity of L. monocytogenes ATCC 19115 in response to exposure to ELEO was determined according to the standard procedure described by Miksusanti et al. (37). Bacterial cultures treated with an MIC of ELEO (12.5 mg/mL) were seeded on NA plates supplemented with different concentrations of NaCl (0, 2.5, 5.0, and 10.0%) and then incubated at 37°C for 24 hours. The control plates were seeded with only the bacterial culture treated with 5% DMSO. The number of colonies grown after incubation was counted and expressed in terms of Log10 (CFU/mL).
3.5. Statistical Analysis
All data are expressed as the means ± standard deviation (SD). Significant differences among treatments were identified by one-way analysis of variance followed by Duncan’s multiple range tests at P < 0.05 using Statistical Analysis Software (SAS) version 9.4 (SAS Institute Inc., Cary, NC, USA).
4. Results
The color and percentage yields of the EOs were as follows: E. linza (deep yellow, 0.32%), U. pinnatifida (yellowish, 0.26%), L. japonica (yellowish, 0.85%), and P. tenera (light yellowish, 1.41%). The antilisterial activity of the EOs from the four different edible seaweeds against three different strains of L. monocytogenes were qualitatively and quantitatively determined with the disc diffusion assay (Table 1). Among the four EOs, ELEO was the most active against all three strains of L. monocytogenes, producing zones of inhibition with diameters ranging from 11.3 - 16 mm (Table 1). The EOs from L. japonica, U. pinnatifida, and P. tenera were also active against L. monocytogenes ATCC 19115 and 7644, with zones of inhibition from 10.0 - 15.0 mm, whereas they were inactive against L. monocytogenes ATCC 19114 (Table 1). As the negative control, DMSO did not show any zone of inhibition, while kanamycin, the positive control, generated a zone of inhibition of 16.6 - 21.0 mm (Table 1). The EOs of E. linza, U. pinnatifida, and P. tenera had MIC and MBC values from 12.5 - 25 mg/m, whereas the EO of L. japonica had MIC and MBC values of 25 mg/mL (Table 1).
The ELEO had the most anti-listerial activity and was further investigated for its mechanism of anti-listerial action using L. monocytogenes ATCC 19115. Various assays, such as the time kill assay, cell membrane permeability, release of 260-nm absorbing materials, leakage of potassium ions, and loss of salt tolerance capacity, were undertaken. The effects of ELEO on the viability of L. monocytogenes ATCC 19115 are presented in Figure 1A. Exposure of the bacterial cells to ELEO reduced the number of CFUs with respect to time. The ELEO at the MIC did not show any significant reduction in the viable cell count of the bacteria until after 2 hours of incubation. However, the growth of the viable cells was controlled following 4 hours of incubation.
The permeability of the cell membrane of L. monocytogenes ATCC 19115 under the influence of ELEO was measured based on the relative electrical conductivity (Figure 1B). Exposure of the bacterial cells to the ELEO at the MIC increased the relative electrical conductivities with respect to the incubation time, with a sharp increase occurring after 6 hours of incubation (Figure 1B). However, the control was treated with DMSO and showed no change in its relative electrical conductivity. The culture filtrates exposed to the ELEO at the MIC displayed a continual increase in the concentration of 260-nm absorbing materials over 120 min of incubation (Figure 2).
The untreated control sample showed no marked increase in the concentration of 260-nm absorbing materials. The L. monocytogenes ATCC 19115 treated with ELEO at the MIC displayed an increase in the leakage of K+ ions with respect to time, whereas the untreated control cells showed no sign of K+ ion leakage (Figure 3). The effects of ELEO on the loss of salt tolerance capacity of L. monocytogenes ATCC 19115 is presented in Figure 4. Bacterial cells pretreated with ELEO at the MIC showed a gradual decrease in their number of viable cells with respect to the untreated control sample when grown on NA plates supplemented with different concentrations of NaCl (0, 2.5, 5.0, and 10.0%).
Effects of Enteromorpha linza Essential Oil (ELEO)
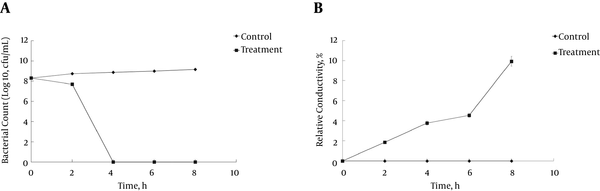
Effects of Enteromorpha linza Essential Oil (ELEO) at the Minimum Inhibitory Concentration on the Release of 260-nm Absorbing Material From L. monocytogenes ATCC 1611519115
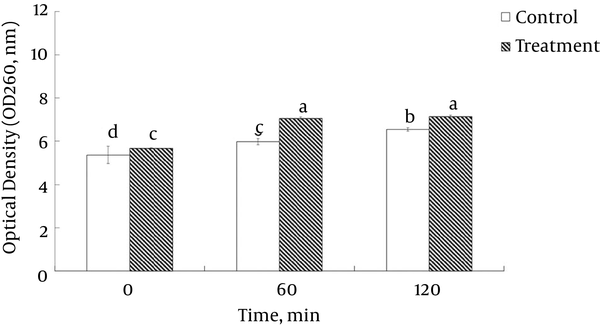
Effects of Enteromorpha linza Essential Oil (ELEO) at the Minimum Inhibitory Concentration on the Leakage of K+ Ions of L. monocytogenes ATCC 19115
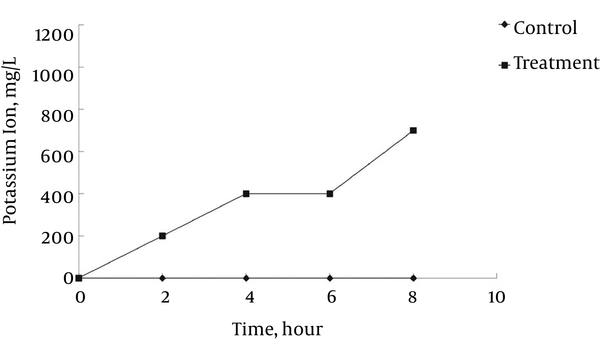
Effects of Enteromorpha linza Essential Oil (ELEO) at the Minimum Inhibitory Concentration on the loss of the Salt Tolerance Capacity of L. monocytogenes ATCC 19115
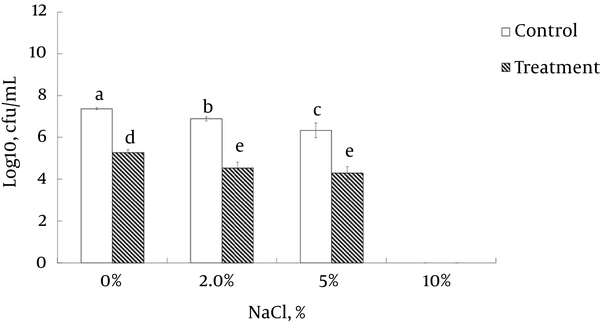
5. Discussion
The yield percentage of EOs in the case of fresh seaweed (E. linza and U. pinnatifida) was comparatively less than that of dry seaweed (L. japonica and P. tenera), which might be due to the decreased moisture content present in the dry seaweed. Similar results were reported previously (38, 39). All four EOs were active against the three strains of L. monocytogenes (Table 1). Several studies have investigated the antimicrobial potential of EOs from seaweed (40-42). Although the antibacterial potentials of E. linza (43), Laminaria, and Undaria species (44, 45) and P. tenera (45) have been reported, our results are the first to indicate that the EOs from E. linza, U. pinnatifida, L. japonica, and P. tenera have a controlling effect on some strains of L. monocytogenes. The results showed that the MIC and MBC values (Table 1) of the EOs were higher than that of kanamycin, the standard antibiotic (Table 1). One possible reason for this finding might be that the EOs are a mixture of a number of bioactive compounds, while only a few are responsible for its antibacterial potential; when these active compounds are purified, their concentrations will be significantly lower. A similar result was reported by Gressler et al. (42), who stated that EOs as a whole did not show any activity, but when their compounds, particularly the sesquiterpenes, were purified and tested individually, they showed potent activity.
Antilisterial Activity of EOs From Four Different Edible Seaweeds Against Three Strains of L. monocytogenesa
Foodborne bacteria | E. linza | U. pinnatifida | L. japonica | P. tenera | Standard antibioticb | ||||||||
---|---|---|---|---|---|---|---|---|---|---|---|---|---|
Inhibitionc | MICd | MBCd | Inhibitionc | MIC | MBC | Inhibitionc | MIC | MBC | Inhibitionc | MIC | MBC | ||
L. monocytogenes ATCC 19115 | 16.0 ± 4.4BC | 12.5 | 25.0 | 10.5±0.6D | 12.5 | 12.5 | 10.0 ± 0D | 25.0 | 25.0 | 10.5 ± 0.6D | 25.0 | 25.0 | 21.0 ± 4.2A |
L. monocytogenes ATCC 19114 | 11.3 ± 0.6D | 25.0 | 25.0 | NA | NA | NA | NA | NA | NA | NA | NA | NA | 16.7 ± 1.5AB |
L. monocytogenes ATCC 7644 | 15.7 ± 1.5BC | 12.5 | 25.0 | 11.0±0.6D | 25.0 | 25.0 | 15.0 ± 1.0BC | 25.0 | 25.0 | 13.0 ± 1.7CD | 12.5 | 25.0 | 17.6 ± 2.1AB |
The anti-listerial mode of action of ELEO against different strains of L. monocytogenes was studied using several assays. The viability of the bacterial cells ceased after 4 hours of incubation in the case of the ELEO-treated bacteria (Figure 1A). A possible reason for this effect might be that the hydrophobic ELEO easily penetrated into the cytosol of the treated bacteria due to the nature of the outer membrane of the Gram-positive L. monocytogenes and resulted in the rupture of the outer cellular membrane, causing cell lysis and death.
A similar hypothesis on the effect of EOs on pathogenic bacteria was previously reported (46). There was also a gradual increase in the relative electrical conductivity and the OD values at 260 nm of L. monocytogenes ATCC 19115 treated with ELEO over time (Figure 1B and 2). One possible reason behind this outcome might be the loss of ions from inside the bacterial cells to the outer environment following the breakdown of the cytoplasmic membrane. The cellular leakage could also result from the effect of penetration of the hydrophobic ELEO. Similar observations regarding the release of ions outside of bacterial cells treated with EOs from rosewood, oregano, lemongrass, and other EOs have also been reported (37, 47-50). The marked leakage of cytosolic materials was considered an indication of gross and irreparable damage to the cytoplasmic membrane (51). Thus, the leakage of ions and other cytosolic materials from the bacteria treated with ELEO could be a strong possible mode of anti-listerial action against the bacteria.
Furthermore, the loss of K+ ions from L. monocytogenes ATCC 19115 treated with ELEO (Figure 3) also confirmed the hypothesis that ELEO acts by disrupting the bacterial cell membrane. As shown by the increase of the relative electrical conductivity after 6 hours of incubation (Figure 1B), a sharp increase indicating significant leakage of K+ ions was also observed after 6 hours of incubation (Figure 3). It is well known that the bacterial cytoplasmic membrane provides a selective barrier to prevent small ions, such as K+, Ca+2, and Na+, from entering and exiting the cell; this control of cell membrane permeability is essential to various cellular activities, such as transport, energy transduction processes, and cell maintenance (36, 52, 53). It is assumed that the hydrophobic ELEO could induce deformities in the cell membrane, which cause cellular damage and lysis and consequently result in the leakage of potassium ions from the bacterial cell. The loss in the ability of the bacteria to sustain a higher salt concentration (Figure 4) also indicated the effect of ELEO on the bacterial cell membrane, producing membrane damage due to the sublethal injury. This lost ability might also affect the capability of the membrane to adequately osmoregulate the cell under high salt concentrations and thus induce cell death. Similar results have been previously reported (37), which confirmed our hypothesis on the anti-listerial mode of action of ELEO.
The present study confirmed the effective anti-listerial potential of the four EOs against multiple strains of the important foodborne pathogen, L. monocytogenes. Additionally, the results presented herein provided insight into a possible explanation for the modes of action of EOs on L. monocytogenes. Seaweed EOs can penetrate the cytoplasmic membrane, which causes a loss of the membrane permeability of the cellular membrane, leakage of the cytoplasm, and decreased ability to osmoregulate during high salt stress. Further, more detailed study on the mechanism of action will be required to depict and confirm the exact effect of ELEO on foodborne pathogens. The outcome of the present study may help food industries identify the most promising potential anti-listerial agent from edible seaweed sources to control L. monocytogenes and facilitate their application in food processing and preservation in a nontoxic and environmentally friendly manner.
References
-
1.
Burt S. Essential oils: their antibacterial properties and potential applications in foods--a review. Int J Food Microbiol. 2004;94(3):223-53. [PubMed ID: 15246235]. https://doi.org/10.1016/j.ijfoodmicro.2004.03.022.
-
2.
Celikel N, Kavas G. Antimicrobial properties of some essential oils against some pathogenic microorganisms. Czech J Food Sci. 2008;26(3):174-81.
-
3.
El Abed N, Kaabi B, Smaali M, Chabbouh M, Habibi K, Mejri M, et al. Chemical composition, antioxidant and antimicrobial activities of Thymus capitata essential oil with its preservative effect against Listeria monocytogenes inoculated in minced beef meat. Evid Based Complement Altern Med. 2014;2014. https://doi.org/10.1155/2014/152487.
-
4.
Alzoreky NS, Nakahara K. Antibacterial activity of extracts from some edible plants commonly consumed in Asia. Int J Food Microbiol. 2003;80(3):223-30. [PubMed ID: 12423924].
-
5.
Shan B, Cai YZ, Brooks JD, Corke H. The in vitro antibacterial activity of dietary spice and medicinal herb extracts. Int J Food Microbiol. 2007;117(1):112-9. [PubMed ID: 17449125]. https://doi.org/10.1016/j.ijfoodmicro.2007.03.003.
-
6.
Viuda-Martos M, Mohamady MA, Fernández-Lopez J, ElRazik K, Omer EA, Pérez-Alvarez JA, et al. In vitro antioxidant and antibacterial activities of essentials oils obtained from Egyptian aromatic plants. Food Control. 2011;22(11):1715-22. https://doi.org/10.1016/j.foodcont.2011.04.003.
-
7.
Mbata TI, Saikia A. Antibacterial activity of essential oil from Ocimum gratissimum on Listeria monocytogenes. Int J Food Safety. 2005;5(7):15-9.
-
8.
van Kuijk S, Noll KS, Chikindas ML. The species-specific mode of action of the antimicrobial peptide subtilosin against Listeria monocytogenes Scott A. Lett Appl Microbiol. 2012;54(1):52-8. [PubMed ID: 22040458]. https://doi.org/10.1111/j.1472-765X.2011.03170.x.
-
9.
Gandhi M, Chikindas ML. Listeria: A foodborne pathogen that knows how to survive. Int J Food Microbiol. 2007;113(1):1-15. [PubMed ID: 17010463]. https://doi.org/10.1016/j.ijfoodmicro.2006.07.008.
-
10.
Warriner K, Namvar A. What is the hysteria with Listeria? Trends Food Sci Technol. 2009;20(6):245-54. https://doi.org/10.1016/j.tifs.2009.03.008.
-
11.
Allerberger F, Wagner M. Listeriosis: a resurgent foodborne infection. Clin Microbiol Infect. 2010;16(1):16-23. [PubMed ID: 20002687]. https://doi.org/10.1111/j.1469-0691.2009.03109.x.
-
12.
Donnelly CW. Listeria monocytogenes: a continuing challenge. Nutr Rev. 2001;59(6):183-94. [PubMed ID: 11444596].
-
13.
Pirbalouti A, Rahimi E, Moosavi S. Antimicrobial activity of essential oils of three herbs against Listeria monocytogenes on chicken frankfurters. Acta agriculturae Slovenica. 2010;95(3):219. https://doi.org/10.2478/v10014-010-0013-1.
-
14.
Garcia MT, Marinez Canamero M, Lucas R, Ben Omar N, Perez Pulido R, Galvez A. Inhibition of Listeria monocytogenes by enterocin EJ97 produced by Enterococcus faecalis EJ97. Int J Food Microbiol. 2004;90(2):161-70. [PubMed ID: 14698097].
-
15.
Chan YC, Wiedmann M. Physiology and genetics of Listeria monocytogenes survival and growth at cold temperatures. Crit Rev Food Sci Nutr. 2009;49(3):237-53. [PubMed ID: 19093268]. https://doi.org/10.1080/10408390701856272.
-
16.
Fouladynezhad N, Afsah Hejri L, Rukayadi Y, Nakaguchi Y, Nishibuchi M, Radu S. Efficiency of four Malaysian commercial disinfectants on removing Listeria monocytogenes biofilm. Int Food Res J. 2013;20(3):1485-90.
-
17.
Faid M. Rai M, Chikindas ML, editors. Seven antimicrobials from marine algae. CAB; 2011.
-
18.
Chapman VJ, Chapman DJ. Seaweeds and their uses. Chapman and Hall, London; 1980.
-
19.
Say PJ, Burrows IG, Whitton BA. Enteromorpha as a monitor of heavy metals in estuarine and coastal intertidal waters: A method for the sampling, treatment and analysis of the seaweed enteramorpha to monitor heavy metals in estuaries and coastal waters. Northern Environmental Consultants; 1986.
-
20.
Lee I. An annotated account of korean economic seaweeds for food, medical and industrial uses. ALGAE. 1990;(y1990m00a):57.
-
21.
Li N, Zhang Q, Song J. Toxicological evaluation of fucoidan extracted from Laminaria japonica in Wistar rats. Food Chem Toxicol. 2005;43(3):421-6. [PubMed ID: 15680677]. https://doi.org/10.1016/j.fct.2004.12.001.
-
22.
Yun S, Jang J, Lee J. Isolation and identification of an antibacterial substance from sea mustard, Undaria pinnatifida, for Streptococcus mutans. J Korean Soc Food Sci Nutri. 2007;36(2):149-54. https://doi.org/10.3746/jkfn.2007.36.2.149.
-
23.
Hwang ES, Thi ND. Effects of Extraction and Processing Methods on Antioxidant Compound Contents and Radical Scavenging Activities of Laver (Porphyra tenera). Prev Nutr Food Sci. 2014;19(1):40-8. [PubMed ID: 24772408]. https://doi.org/10.3746/pnf.2014.19.1.040.
-
24.
Lis-Balchin M, Deans SG. Bioactivity of selected plant essential oils against Listeria monocytogenes. J Appl Microbiol. 1997;82(6):759-62. [PubMed ID: 9202441].
-
25.
Rota C, Carraminana JJ, Burillo J, Herrera A. In vitro antimicrobial activity of essential oils from aromatic plants against selected foodborne pathogens. J Food Prot. 2004;67(6):1252-6. [PubMed ID: 15222560].
-
26.
Salvador N, Gómez Garreta A, Lavelli L, Ribera M. Antimicrobial activity of Iberian macroalgae. Scientia Marina. 2007;71(1):101-14.
-
27.
Souhaili Z, Mohammadi H, Habti N, Faid M. Lethal effect of the aqueous extract of the brown marine algae (Cystoseira tamariscifolia) on the mouse and the myelom. cells Afrique Sci. 2008;4:580-90.
-
28.
Kandhasamy M, Arunachalam KD. Evaluation of in vitro antibacterial property of seaweeds of southeast coast of India. Afr J Biotechnol. 2008;7(12).
-
29.
Rajasulochana P, Dhamotharan R, Krishnamoorthy P, Murugesan S. Antibacterial activity of the extracts of marine red and brown algae. J Am Sci. 2009;5(3):20-5.
-
30.
Plaza M, Santoyo S, Jaime L, Garcia-Blairsy Reina G, Herrero M, Senorans FJ, et al. Screening for bioactive compounds from algae. J Pharm Biomed Anal. 2010;51(2):450-5. [PubMed ID: 19375880]. https://doi.org/10.1016/j.jpba.2009.03.016.
-
31.
Diao WR, Hu QP, Feng SS, Li WQ, Xu JG. Chemical composition and antibacterial activity of the essential oil from green huajiao (Zanthoxylum schinifolium) against selected foodborne pathogens. J Agric Food Chem. 2013;61(25):6044-9. [PubMed ID: 23758080]. https://doi.org/10.1021/jf4007856.
-
32.
Kubo I, Fujita K, Kubo A, Nihei K, Ogura T. Antibacterial activity of coriander volatile compounds against Salmonella choleraesuis. J Agric Food Chem. 2004;52(11):3329-32. [PubMed ID: 15161192]. https://doi.org/10.1021/jf0354186.
-
33.
Bajpai VK, Al-Reza SM, Choi UK, Lee JH, Kang SC. Chemical composition, antibacterial and antioxidant activities of leaf essential oil and extracts of Metasequioa glyptostroboides Miki ex Hu. Food Chem Toxicol. 2009;47(8):1876-83. [PubMed ID: 19426779]. https://doi.org/10.1016/j.fct.2009.04.043.
-
34.
Ye X, Li X, Yuan L, He H. Effect of the surface activity on the antibacterial activity of octadecanoyl acetal sodium sulfite series. Colloid Surface A. 2005;268(1):85-9.
-
35.
Carson CF, Mee BJ, Riley TV. Mechanism of action of Melaleuca alternifolia (tea tree) oil on Staphylococcus aureus determined by time-kill, lysis, leakage, and salt tolerance assays and electron microscopy. Antimicrob Agents Chemother. 2002;46(6):1914-20. [PubMed ID: 12019108].
-
36.
Bajpai VK, Sharma A, Baek K. Antibacterial Mechanism of Action of Taxus cuspidata Stem Essential Oil against Selected Foodborne Pathogens. J Food Safety. 2013;33(3):348-59.
-
37.
Jenie B, Priosoeryanto B, Syarief R, Rekso G. Mode of action Temu kunci (Kaempferia pandurata) essential oil on E. coli K1. 1 cell determined by leakage of material cell and salt tolerance assays. Hayati J Biosci. 2008;15(2):56-60. https://doi.org/10.4308/hjb.15.2.56.
-
38.
Nicolle D, Dunlop PJ, Bignell CM. A study of the variation with time of the compositions of the essential leaf oils of 16 Eucalyptus species. Flavour Fragr J. 1998;13(5):324-8.
-
39.
Subramanian PA, Gebrekidan A, Nigussie K. Yield, Contents and Chemical Composition Variations in the Essential oils of Different Eucalyptus globulus trees from Tigray, Northern Ethiopia. Northern Ethiopia J Pharma Biomed Sci. 2012;17(17).
-
40.
Koz FFY, Yavasoglu N, Demirel Z. Antioxidant and antimicrobial activities of Codium fragile (Suringar) Hariot (Chlorophyta) essential oil and extracts. Asian J Chem. 2009;21(2):1197.
-
41.
Demirel Z, Yilmaz-Koz F, Karabay-Yavasoglu N, Ozdemir G, Sukatar A. Antimicrobial and antioxidant activities of solvent extracts and the essential oil composition of Laurencia obtusa and Laurencia obtusa var. pyramidata. Romanian Biotechnol Lett. 2011;16(1):5927-36.
-
42.
Gressler V, Stein E, Dörr F, Fujii MT, Colepicolo P, Pinto E. Sesquiterpenes from the essential oil of Laurencia dendroidea (Ceramiales, Rhodophyta): isolation, biological activities and distribution among seaweeds. Braz J Pharma. 2011;21(2):248-54. https://doi.org/10.1590/S0102-695X2011005000059.
-
43.
Sukatar A, Karabay-Yavassoglu N, Ozdemir G, Horzum Z. Antimicrobial activity of volatile component and various extracts ofEnteromorpha linza (Linnaeus) J. Agardh from the coast of Izmir, Turkey. Anal Microbiol. 2006;56(3):275-9.
-
44.
Kajiwara T, Matsui K, Akakabe Y, Murakawa T, Arai C. Antimicrobial browning-inhibitory effect of flavor compounds in seaweeds. J Appl Phycol. 2006;18(3-5):413-22. https://doi.org/10.1007/978-1-4020-5670-3_24.
-
45.
Hazzani A, Shehata AI, Moubayed NMS, Houri HJA. Antimicrobial and biochemical properties of selected edible brown and red marine macroalgae. J Pure Appl Microbiol. 2014;8:1275-82.
-
46.
Sikkema J, de Bont JA, Poolman B. Interactions of cyclic hydrocarbons with biological membranes. J Biol Chem. 1994;269(11):8022-8. [PubMed ID: 8132524].
-
47.
Horne D, Holm M, Oberg C, Chao S, Young D. Antimicrobial effects of essential oils on Streptococcus pneumoniae. J Essential Oil Res. 2001;13(5):387-92. https://doi.org/10.1080/10412905.2001.9712241.
-
48.
Galindo J, Hernandez A, Dayan FE, Tellez MR, Macı́as FA, Paul RN, et al. Dehydrozaluzanin C, a natural sesquiterpenolide, causes rapid plasma membrane leakage. Phytochemistry. 1999;52(5):805-13. https://doi.org/10.1016/S0031-9422(99)00303-9.
-
49.
Kong M, Chen XG, Liu CS, Liu CG, Meng XH, Yu le J. Antibacterial mechanism of chitosan microspheres in a solid dispersing system against E. coli. Colloids Surf B Biointerfaces. 2008;65(2):197-202. [PubMed ID: 18508247]. https://doi.org/10.1016/j.colsurfb.2008.04.003.
-
50.
Chovanova R, Mikulasova M, Vaverkova S. In vitro antibacterial and antibiotic resistance modifying effect of bioactive plant extracts on methicillin-resistant Staphylococcus epidermidis. Int J Microbiol. 2013;2013. https://doi.org/10.1155/2013/760969.
-
51.
Zhou K, Zhou W, Li P, Liu G, Zhang J, Dai Y. Mode of action of pentocin 31-1: an antilisteria bacteriocin produced by Lactobacillus pentosus from Chinese traditional ham. Food Control. 2008;19(8):817-22.
-
52.
Cox SD, Gustafson JE, Mann CM, Markham JL, Liew YC, Hartland RP, et al. Tea tree oil causes K+ leakage and inhibits respiration in Escherichia coli. Lett Appl Microbiol. 1998;26(5):355-8. [PubMed ID: 9674165].
-
53.
Moreira MR, Ponce AG, Del Valle CE, Roura SI. Inhibitory parameters of essential oils to reduce a foodborne pathogen. LWT-Food Sci Technol. 2005;38(5):565-70. https://doi.org/10.1016/j.lwt.2004.07.012.