Abstract
Background:
An important observation during quantification experiments of Staphylococcus epidermidis biofilm is that there is a great difference in the biofilm biomass of different strains despite the same experimental conditions.Objectives:
This study aimed to study the genotypic background beyond differential rates of Polysaccharide Intercellular Adhesion (PIA) production in S. epidermidis biofilm forming strains.Methods:
A number of 126 strains were isolated from blood cultures (n = 40), catheter cultures (n = 50), and other specimens (n = 36). The strains were obtained from patients hospitalized at the bone marrow transplant center of Tunis. Biofilm micro-plate assay, hemagglutination, and susceptibility to proteinase K methods were used to assess biofilm characteristics in the studied strains. Conventional and real time PCR were used to assess genotypic background of biofilm formation.Results:
Using PCR method, we demonstrated that there is a significant difference in ica genes (P < 0.01) and not in adhesion rsb and sar genes distribution between biofilm forming and non-biofilm forming strains. Almost all strains harbored agr type I. None of studied strains harbored IS256 inside ica operon. Ica-independent biofilm formation was detected in 11 strains that were confirmed to have proteinaceous matrix. Using Kernel density estimation, we established that biofilm biomass was higher in ica-dependent than ica-independent biofilm forming population. Using qRT-PCR, we found a significant correlation between biofilm biomass and RNAIII expression level (r2 = 0.95); but no correlation was found for biofilm biomass neither with icaA nor with ccpA genes.Conclusions:
Data reported here indicated that there is no specific genetic combination beyond the quantity of biofilm biomass in S. epidermidis. Biofilm biomass seemed to be controlled by RNAIII expression level. Further interest should be directed to biofilm dispersal since it seems that the key difference in biofilm biomass ability of S. epidermidis strains relates to factors regulating this stage.Keywords
1. Background
Living in the edge between commensalism and pathogenicity, Staphylococcus epidermidis, which normally colonizes human epithelium and mucous membrane (1), is now among the most frequent bacterial species responsible for nosocomial infections (2). This is true especially for those associated with indwelling medical devices such as prosthesis, prosthetic heart valves, and catheters (3). This fact is mainly associated with interesting strategies that S. epidermidis has developed to conquer hospital environment as a novel ecological niche and to transform into a notorious pathogen (4).
One of those strategies that may be the most important one is the propensity to form an adherent multilayered biofilm on the surface of biomaterials (5). The first step of biofilm formation is initiated with the interaction between the “microbial surface components recognizing adhesive matrix molecules” (MSCRAMM) and host matrix proteins or medical implant polymers (4). Among those bacterial adhesion proteins, the most described ones are the fibrinogen binding protein (fbe), fibronectin binding protein (embp), and autolysin E (atlE) which the latter interacts with fibrinogen and fibronectin, respectively (4). Biofilm accumulation comes directly after a good establishment of stable MSCRAMM-targeted molecule complex. In some cases, biofilm accumulation is mediated by proteins such as accumulation associated protein (aap) (6), biofilm homologous protein (bhp) (7), or the embp protein mentioned above.
In almost all S. epidermidis strains, the accumulation is mediated by the polysaccharide intercellular adhesion (PIA), which has been proved to be the product of ica operon encoded enzymes and the major component of biofilm biomass (5). This polysaccharide not only protects bacterial cells from both antibiotics (4) and immune system (5), but also it is in the origin of persistent bacteria and chronic infection occurrences.
Recent progress in S. epidermidis biofilm genome analysis has given interesting insights into the regulation network of icaADBC genes, which is achieved directly by icaR gene (8) or indirectly by rsb, agr, sar, and luxS (8) genes or most importantly by IS256 insertion sequence (8), a driving force for the flexibility of S. epidermidis genome.
polysaccharide intercellular adhesion production during biofilm formation is yet known as a classical response to several environmental stressors e.g. alcohol, antibiotics, and starvation (9). Genetic elements involved in such responses are mainly rsb and agr genes. RNAIII described as the molecular effector of agr system (10) regulates a large number of proteins all involved in biofilm formation of S. epidermidis which is confirmed to be a multifactorial responding process since it is even depending on proteins involved in central metabolism such as the central catabolism protein A “ccpA” (11).
However, in the absence of any stimulation as for in vitro classical PIA quantification experiments, different strains exhibited different potentials to product PIA and hence, a scale was developed to classify biofilm forming strains in strong, moderate and weak classes (12). Even more, in our lab as in other studies, it was established that there is a tight association between the recorded biofilm biomass and both the type of infection caused by a given strain (13) and the widespread dissemination of multidrug resistant strains (14). This fact suggests that there is an internal determinant allowing each strain to have a precise emplacement in the mentioned scale.
2. Objectives
Based on the described observations, we suggest that the biofilm biomass of a given strain, in the absence of any stimulus, is genetically encoded. Thus, the aim of this work was to verify if there is any correlation between strong, moderate, or weak biofilm forming phenotypes and a specific genetic background.
3. Methods
3.1. Bacterial Strains and Medium
One hundred and twenty six strains were isolated from blood cultures (n = 40), catheter cultures (n = 50), and other specimens (n = 36). The strains were obtained from patients hospitalized at the bone marrow transplant center of Tunis for more than 48 hours in the graft or hematological unit of the specialized center during 2008 - 2009. Initial identification was carried out with the usual method using ApiID32Staph system (Bio Merieux, Marcy l’Etoile, France), and the confirmation was done by specific PCR amplification of 16s RNA encoding gene using appropriate primers (Table 1).
Primers Used in PCR and RT-PCR Amplification of Different Studied Genes
Locus | Sequence 5’ → 3’ | Product Size, pb | Reference |
---|---|---|---|
icaADBC | F : TGC ACT CAA TGA GGG AAT CA | 2725 | (16) |
R : AAT CAC TAC CGG AAA CAG CG | |||
icaR | F : TAA TCC CGA ATT TTT GTG AA | 469 | |
R : AAC GCA ATA ACC TTA TTT TCC | |||
icaA | F : ACA GTC GCT ACG AAA AGA AA | 103 | |
R : GGA AAT GCC ATA ATG ACA AC | |||
icaD | F : ATG GTC AAG CCC AGA CAG AG | 198 | |
R : CGT GTT TTC AAC ATT TAA TGC AA | |||
icaB | F : CTG ATC AAG AAT TTA AAT CAC AAA | 302 | |
R : AAA GTC CCA TAA GCC TGT TT | |||
icaCα | F : TGA AGA AAA ATA AACTTG AAT TAG TG | 127 | |
R : TGC AAT ATG AGT GAA CTA TCA GA | |||
icaCβ | F : TTT ACG TGC GTT TAT TTG TG | 532 | |
R : CAT TGT ATT TTC GCT TAA TGG | |||
icaCγ | F : CTT ATC ACC GCT TCT TCT TTT | 403 | |
R : CGG AAA CAG CGA TAA ATA AA | |||
icaCδ | F : TAA CTT TAG GCG CAT ATG TTT T | 400 | |
R : TTCCAG TTA GGCTGG TAT TG | |||
icaCξ | F : GCT GTT TCC GGT AGT GAT TA | 253 | |
R : TTA AAA GTG AAA TCG CCA AG | |||
is256 | F : TGAAAAGCGAAGAGATTCAAAGC | 1102 | (39) |
R : ATGTAGGTCCATAAGAACGGC | |||
atlE | F : GCTAAGGCACCAGTAAAAAGT | 480 | (40) |
R : GACCTCATCTTGTTTTACCCA | |||
fbe | F : TAAACACCGACGATAATAACCAAA | 495 | (41) |
R : GGTCTAGCCTTATTTTCATATTCA | |||
aap | F : CAACGAAGGCAGAAGAAGGA | 719 | (40) |
R : CATCCCCATCTTTCTTGCTG | |||
embp | F : GCTAAGGCACCAGTAAAAAGT | 455 | (42) |
R : GACCTCATCTTGTTTTACCCA | |||
bhp | F : TGGTATTAGGAAGCTCTCAG | 935 | (43) |
R : ATACCAGCGTGACGCAAATC | |||
agicartypeA | F : GCT GCA ACC AAG AAA CAA CC | 1022 | (44) |
R : CGT GTA TTC ATA ATA TGC TTC GATT | |||
agrtypeB | F : TAT GCA AGC CAA GCA CTT GT | 453 | |
R : GTG CGA AAG CCG ATA ACA AT | |||
agrtypeC | F : CCT TGGC TAG TAC TAC ACC TTC | 615 | |
R : GTG CTT GGC TTG CAT AAA CA | |||
RNAIII | F : GAA AGC ATG CCT AAC TGT TAA AAA | 904 | (45) |
R : GGTGATGATGGTCACACCAA | |||
sigmaB | F : TCA GAC CAA GGT GAA AGT TTT G | 1299 | |
R : CGA TTT ATT TCA ATC AGA GTA CC | |||
rsbU | F : GGA AGT AAG GAG GCG CAT TT | 884 | |
R : TCA CGT GCC TCT GTA ACA CC | |||
rsbV | F : TTG GTG GAG AAT TGG ACG TA | 354 | |
R : GGC AAC CGC ATT TCA ATA TAA | |||
rsbW | F : CAG GCC TCG GTT TAT TCG TA | 1040 | |
R : TGA AAT CGC ACA ACG CTT AG | |||
sarA | F : TGG TCA CTT ATG CTG ACA GAT T | 313 | (46) |
R : TTT GCT TCT GTG ATA CGG TTG | |||
sarR | F : TGC ACG CTT CTC TTT TTA GA | 225 | (*) |
R : GGT TAA TGC GAC ATT TCA AG | |||
sarZ | F : TGT ATG TAG AGA ATA GTT ATT TGA GCA | 410 | |
R : AAA ATT TTG CAA GTC TTC AAC T | |||
16sRNA | F : GGA ATT CAA AGG AAT TGA CGG GGG C | 478 | (46) |
R : CGG GAT CCC AGG CCC GGG AAC GTA TTC AC | |||
gmk | F: GGATAATGAAAAAGGATTGTTAATCG | (47) | |
R: GCTTCTACGCGCTCTCTTTT | |||
ccpa | F : AGC CGA AGA AGC AAC ACA AT | (*) | |
R : CTG AGT TGT CCC ACG GTA TTC |
The reference strain was S. epidermidis RP62A described previously (15) that was purchased from collection de l’Institut Pasteur (Paris, France). Muller Hinton agar (MHA), Tryptocasein Soja Agar (TSA), and Tryptocasein Soja Broth (TSB) supplemented with glucose (Biorad, Marnes-La Coquette, France) were used in different experiments.
3.2. Biofilm Assay
Detection of the produced PIA was carried out using 96-well microplate experiment according to Stepanovic recommendations (12). Briefly, 0.5 Mc suspensions of tested strains were incubated at 37°C for 24 hours and then diluted to 1:100 in TSB containing 0.25% w/v glucose. Each of three wells of the microplate was filled with 200 µL of the diluted suspension of each strain. After 24 hours incubation at 37°C, plates were gently washed with tap water to eliminate planktonic cells, and then air dried at room temperature. Each well was filled with 150 µL of 1% (w/v) crystal violet for 10 minutes incubation at room temperature. Excessive colorant was eliminated with consecutive washes with tap water. Once dried, wells were filled with 150 µL of 33% (v/v) of glacial acetic acid and incubated for 15 minutes at room temperature. Optical density (OD) was then read at 620 nm with ELISA reader. According to their OD values, the strains were classified into weak, moderate, or strong producer as described elsewhere (12). The experiments were conducted in triplicate for each strain with RP62A as positive control and germ-free well as negative control.
3.3. PCR Assay
DNA was extracted from bacterial cells using phenol-chloroform method. Primers and PCR conditions are summarized in Table 1. For some genes, primers design and PCR products size estimation were carried out using Pimer-Blast online tool (http://www.ncbi.nlm.nih.gov/tools/primer-blast/) and Gel-Pro Analyzer (version 3.1) software (Media Cybernetics, Silver Spring, Md.), respectively. For strains which were not harboring the entire ica operon, simplex PCR assays were carried out to locate any insertion of IS256 inside ica operon as described elsewhere (16).
3.4. Biofilm of ica Independent Strains
For strains suspected to have proteinaceous biofilm (ica-independent biofilm), two tests were carried out based on described properties of PIA: hemagglutination activity and susceptibility to proteinase K (pK) treatment.
3.5. Hemagglutination Assay
This assay was carried out as previously described (17). Briefly, 5 mL of human blood collected with heparin were added to 45 mL of sterile phosphate buffer saline (PBS) and then, centrifuged twice at 2500 rpm for 10 minutes. Next, 100 µL of the pallet were mixed with 10 mL PBS to have a 1% erythrocyte solution used in the hemagglutination assays.
For bacterial cells, two colonies of an overnight culture on TSA were grown in fresh TSB supplemented with 0.25% glucose for 18 hours. Next, bacterial suspensions were centrifuged at 5000 rpm for 10 minutes and the cells were then resuspended in 1 mL of PBS. For each strain, serial twofold dilutions were made in a separate row of 96-well (U-shaped) microtiter plates to have a final volume of 100 μL. To each well, 100 µL of the erythrocyte solution were added and the total volume of each well was pipetted in and out to ensure thorough mixing of the bacterial cells. Then, erythrocytes plate was incubated for 2 hours at room temperature, and hemagglutination titers were evaluated macroscopically. The reference strain of RP62A and free-germ sterile PBS were used as positive and negative controls, respectively.
3.6. Biofilm Susceptibility to pK
Biofilm stability assay against proteolytic action of pK was carried out in a 96well (Flat bottom) plate as previously described (18). Briefly, using the same growth conditions described above in the biofilm assay and after 18 hours incubation at 37°C, the old medium was removed and substituted with fresh one supplemented with 100 µg/mL pK incubated for 2 hours at 55°C. The plate then was treated exactly as for biofilm revelation in biofilm assay. Biofilm reduction for each strain was calculated toward the non-treated biofilm of the same strain itself. RP62A strain was considered as negative control which was not affected by pK treatment.
3.7. Kernel Density Estimation
The overall production of PIA in different studied strains populations was displayed using plots of the Epanechnikov kernel density (19). The curves were designed using kernel Microsoft excel add-in downloadable from the official website of the analytical methods committee of the British Royal society of chemistry (www.rsc.org/amc/).
3.8. RT-PCR
This assay was carried out to compare the transcriptional level between the planktonic and the biofilm statutes of icaA, ccpA, RNAIII genes. The Gmk gene was used as internal control gene for normalization of results.
3.9. Biofilm Preparation
Sterile microscope slides were incubated in petri dishes with 0.5McF RP62A suspension already incubated for 24 hours at 37°C then diluted to 1:100 in TSB supplemented with glucose 0.25% (w/v). After 24 hours incubation at 37°C, the slides were washed twice in two baths of sterile PBS for 10 minutes and transferred to 50 mL Falcon tubes containing 10 mg/mL of given antibiotic. After a further incubation for 24 hours at 37°C, the slides were washed as for the previous step and transferred to new 50 mL Falcon tubes and sonificated to collect bacterial cells as described elsewhere (20). Briefly, a cycle of 30 seconds vortexing at 1200 rpm, 1 minute sonification at 40 Hz/s and again 30 seconds vortexing at 1200 rpm were performed in a total volume of 50 mL of sterile PBS. After sonification, the slides were removed and Falcon tubes were subjected to centrifugation for 10 minutes at 5000 rpm; then, supernatants were removed and the cell pallet was resuspended in 1 mL of sterile PBS.
3.10. RNA Extraction and DNAc Synthesis
Recovered biofilms from the previous experiment were subjected to total RNA extraction using FavorPrepTM. Total RNA extraction kit (Favorgene, Vienna, Austria) as described by the manufacturer. Extracted RNA was stored at -80°C if not immediately used. cDNA synthesis was carried out using CycleScript Reverse TranscriptaseTM kit (Bioneer, Alameda, California, USA), primers targeting icaA, ccpA, RNAIII and gmk genes indicated in Table 1 previously extracted RNA as template. Obtained cDNA was stored at -20°C if not immediately used.
3.11. Quantitative Real Time PCR
Real-time PCR was performed in the Exicycler 96TM real time PCR system with SYBR GreenI premix kit (Bioneer, Alameda, California, USA). Individual real-time PCR reactions were carried out using the default thermocycler program furnished with the kit and the same primers for cDNA synthesis described in Table 2.
Frequencies of Different Genes Involved in Biofilm Formation in Studied S. epidermidis Strainsa
Biofilm Forming Strains 57 (45.3) | Non-Biofilm Forming Strains 69 (54.7) | P Valueb | ||
---|---|---|---|---|
ica genes | icaADBC | 46 (80.7) | 5 (7.2) | < 0.001 |
ica R | 45(79) | 68 (98.5) | < 0.001 | |
ica A | 46 (80.7) | 5 (7.2) | < 0.001 | |
ica D | 46 (80.7) | 5 (7.2) | < 0.001 | |
ica B | 46 (80.7) | 5 (7.2) | < 0.001 | |
ica Cα | 46 (80.7) | 5 (7.2) | < 0.001 | |
ica Cβ | 46 (80.7) | 5 (7.2) | < 0.001 | |
ica Cγ | 46 (80.7) | 5 (7.2) | < 0.001 | |
ica Cδ | 46 (80.7) | 5 (7.2) | < 0.001 | |
ica Cε | 46 (80.7) | 5 (7.2) | < 0.001 | |
IS256 | 30 (52.6) | 35 (50.7) | - | |
rsb genes | rsbU | 57 (100) | 69 (100) | - |
rsbV | 57 (100) | 69 (100) | - | |
rsbW | 57 (100) | 69 (100) | - | |
sigmaB | 43 (75.4) | 51 (73.91) | - | |
Quorum sensing genes | agrtypeI | 52 (91.2) | 55 (79.71) | - |
agr type II | 0 | 0 | - | |
agr type III | 0 | 2 (2.8) | - | |
RNAIII | 52 (91.2) | 55 (79.71) | - | |
LuxS | 54 (97.7) | 61 (88.4) | - | |
Sar genes | Sar A | 57 (100) | 67 (97.1) | - |
Sar R | 54 (97.7) | 61 (88.64) | - | |
Sar Z | 53 (93) | 60 (87) | - | |
MSCRAMM | atlE | 49 (86) | 63 (91.3) | - |
fbe | 49 (86) | 63 (91.3) | - | |
Accumulation genes | aap | 30 (52.6) | 35(50.7) | - |
bhp | 16 (28) | 19 (27.5) | - | |
embp | 49 (86) | 63 (91.3) | - |
3.12. 2-ΔΔCT Calculation
The expression levels were based on the Ct value of each sample for icaA, sigmaB, and RNAIII. CT values were normalized to the internal standard gene gmk and differences toward control sample were calculated using 2-ΔΔCT method where ΔΔCt = [(mCt gene1b - Ct gmkb) - (mCt gene1p - Ct gmkp] (21), “b” and “p” in index refer to biofilm and planktonic statute, respectively. The Ct parameter was defined as the cycle number at which the amplification curve passed a fixed threshold line; this parameter is automatically recorded by the Exicycler system. In each assay, mCt was the mean Ct values of triplicate amplification.
3.13. Statistical Analysis
Data analysis of different assays was carried out using one-way analysis of variance (ANOVA), correlation coefficient, and Chi-square tests in the statistical package for the social sciences software (SPSS V19.0, Inc., Chicago). All tests were performed at the confidence level of 95%.
4. Results
4.1. Biofilm Assay
Biofilm formation was detected in 57 strains, classified as strong (26 strains), moderate (22 strains), or weak (9 strains) biofilm forming strains. Among multi-drug resistant strains, 36 were biofilm forming strains.
4.2. PCR Assay
The entire ica operon was detected in 51 strains. Among the detected strains, only 46 were able to form biofilm. Eleven strains were ica-independent biofilm forming. The insertion sequence IS256 was detected in 47% of strains harboring the ica operon and 54.6% of those not harboring the ica opero. In all cases, the insertion was not inside the ica operon.
No significant differences were detected in the distribution of icaR, rsb, sar, RNAIII, ccpA, or adhesion genes in biofilm forming and non-biofilm forming strains and also in different classes of biofilm forming strains. For quorum sensing systems, all strains except two were harboring agr Type I, and the two remaining strains were harboring agr Type III. LuxS system genes were detected in 91.3% of studied strains. Detailed results are given in Table 2.
4.3. Biofilm of ica Negative Strains
All the 11 ica-independent biofilm forming strains were unable to agglutinate horse erythrocytes as well as they were all sensitive to treatment with pK. Further details are provided in Table 3.
Different Characteristics of ica Negative Biofilm Forming Strains
Reference | BiofilmO.D620 | Hemagglutination Assaya | Biofilm Reduction After pk Treatment, %b | Accumulation Genes | ||
---|---|---|---|---|---|---|
aap | bhp | embp | ||||
84 | 0.54 | - | 61.2 | - | + | - |
1656 | 0.58 | - | 45.7 | + | + | - |
429 | 0.56 | - | 54.4 | + | + | - |
1869 | 0.69 | - | 66.1 | + | + | - |
1468 | 0.67 | - | 65.2 | + | + | + |
1100 | 0.70 | - | 62.4 | + | + | + |
1156 | 0.74 | - | 48.5 | + | + | - |
1400 | 0.70 | - | 39.4 | + | + | + |
3304 | 0.74 | - | 47.7 | - | + | - |
4335 | 0.64 | - | 56.5 | + | + | - |
386 | 0.73 | 41.3 | - | - | + | |
RP62A | 2.31 | 1/4 dilution | 26.1 | + | + | + |
4.4. Kernel Density Estimation
Strains harboring ica genes were able to produce greater quantities of PIA compared to ica negative strains (Figure 1A and 1B).
Kernel Epanechnikov Density Estimation of Biofilm Formation in Different Populations
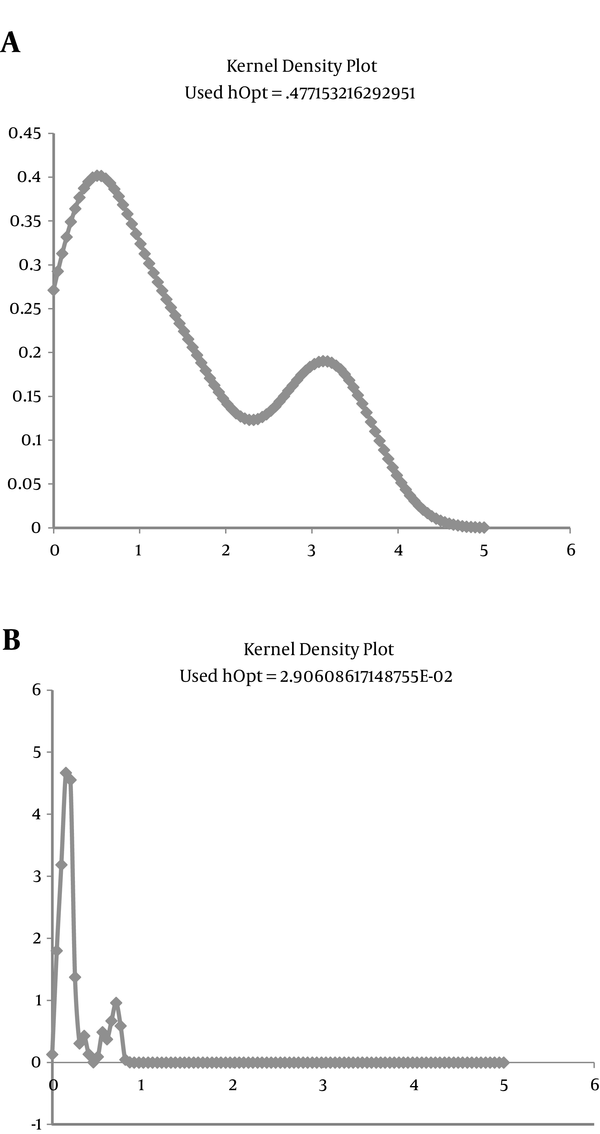
4.5. RT-PCR
IcaA and ccpA transcriptional levels in biofilm growth were weakly correlated with the O.D. recorded in biofilm assay for studied strains (r2 = 0.14 and r2 = 0.18, respectively) (Figure 2A and 2B). RNAIII transcriptional level in biofilm growth was correlated with the O.D. recorded in biofilm assay for studied strains (r2 = 0.95). According to the graph equation (Figure 2C), the increased expression of RNAIII gene was going step by step with decreased O.D.
Correlation Between Optical Density Recorded in Biofilm Quantification and A, icaA; B, ccpA; C, RNAIII Expression
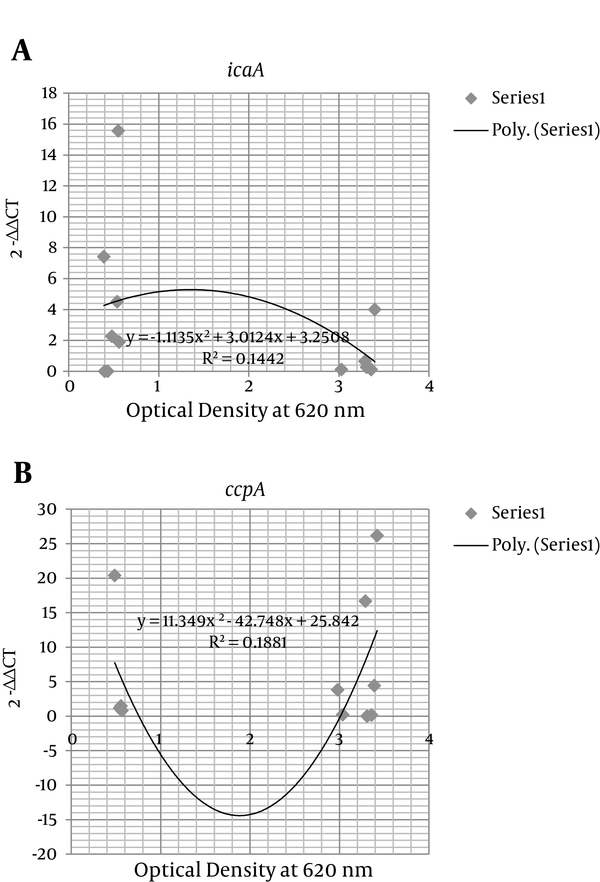
5. Discussion
Biofilm formation is a proved virulence marker of clinical isolates (22, 23). Moreover, the amount of PIA synthesis and hence the ability to form thick biofilm might indicate the pathogenic pathway for a given strain (24). Hereby, we found that biofilm formation was frequent among our strains isolated from catheters and blood cultures. This observation is well associated with advantages provided by biofilm as a life mode to conquer hospital environment (25). Furthermore, important observation to note is that a considerable number of catheter isolates were unable to form biofilm. This fact may be explained by the need of strain to host proteases to trigger biofilm formation through a proteolytic action targeting AAP protein (26). Also, Dice et al. reported that some strains isolated from medical implants were unable to form biofilm in classical 24 hours in vitro tests; indeed, those strains needed six days of consecutive incubation to form their biofilm (27).
The first gene set studied in PCR experiments was those encoding MSCRAMM genes. Here, in our study, those genes were equally distributed on strong, moderate, and weak biofilm forming strains as well as on non-biofilm forming strains. These genes are neglected as molecular virulence determinants since they exist with the same frequencies in healthy people and hospitalized patients (28). They are even described as “not useful to discriminate between invasive and commensal S. epidermidis strains” (29). Nevertheless, lack of some adhesion proteins may not allow any biofilm formation (28). Thus, the existence of those genes is very needful for biofilm formation but it stands far from discriminating commensal from invasive strains as well as they cannot explain the differences in biofilm biomass in the studied strains.
Except icaR, which was more frequent in non-biofilm forming strains, the other ica operon genes were significantly more frequent among biofilm forming strains than non-biofilm forming strains; moreover, only a few strains were harboring ica operon but unable to form biofilm. Since icaR is encoding for a repression regulator, it is obviously clear that this gene could be in the origin of irreversible repression of ica operon expression and hence in non-biofilm forming phenotype (5). The other ica genes are highly frequent in strains isolated from biomaterial associated infections (30), making this molecular marker very suitable to discriminate between invasive and commensal strains (30). In our study, we did not detect the presence of IS256 inside ica operon; therefore, we cannot establish any relation between IS256 presence and the amount of PIA production, at least for studied strains. A phase variation of biofilm phenotype described in S. epidermidis as a result of IS256 insertion in ica operon allows the switch on and off of ica operon and then the dissemination of strain in the patient body (31). Recently, the transposase responsible for IS256 transposition (32) has been identified and transposition sites have been characterized. Using specific primers, Arciola et al. has reported that none of studied strains are harboring IS256 inside ica operon which was physically intact regardless of the amount of PIA produced and hence, the biofilm biomass (16). Two other genes are also described as targets of IS256 including rsbU and sarA (8). They both are crucial for PIA production in S. epidermidis. These genes are ubiquitous and detected in almost all our strains regardless of their ability to produce PIA.
Our strains were all, except two, harboring agr type I allelic form which seems to be specific for clinical strains rather than strains isolated from healthy volunteers. However, this unicity of agr allelic form stands far from being involved in the determination of biofilm biomass in the studied strains. Agr quorum sensing system is often associated with virulence potential of S. epidermidis. Vuong et al. has reported that agr deletion is associated with enhanced in vivo colonization on medical implants since there is upregulation of RNAIII targeted genes involved in the pathogenesis process (33).
LuxS, involved in interspecies communication (33), was the second quorum sensing system investigated in our study. Almost all studied strains, whether they are biofilm producer or not, were LuxS positive. This fact suggests that in our case, LuxS is not clearly correlated with the quantified biofilm biomass. In S. epidermidis, LuxS deletion is associated with over production of PIA, suggesting a biofilm repressive function for this quorum sensing system.
In our study, five strains were not able to produce biofilm despite the presence of intact ica operon, in the meanwhile, 11 of biofilm producer strains did not possess any of ica genes. The first observation confirms the major role of genetic background in PIA production which overcomes ica operon to a major regulatory network (31). The second observation underlies a second recently described mechanism adopted by ica negative strains to form biofilm. Biofilms of those strains are basically proteinaceous, mediated by accumulation factors as aap, embp, or bhp proteins (34). Chemical analysis of such strains has revealed total absence of polysaccharides in biofilm matrix, which is purely composed of proteins (2). Indeed, our 11 ica-independent biofilm forming strains were unable to agglutinate erythrocytes and were abolished after treatment with pK.
Using Kernel density estimation method (19), we noted that biofilm biomass produced by ica positive strains was much higher than the biomass produced by ica negative strains. This reflects the importance of ica operon as principle molecular support of biofilm formation in S. epidermidis; nevertheless, ica independent biofilms are now to be considered as new strategy to conquer hospital environment (2). Furthermore, using the same method, we established that multi drug resistant strains and non-multi drug resistant strains have the same ability to form biofilms. Here, we can clearly estimate that owing to biofilm formation, less resistant strains are in an equal footing with resistant strains and can contribute to nosocomial infections.
During qRT-PCR experiments, we targeted three genes including icaA, ccpA, and RNAIII. Being the first transcript of ica operon genes, icaA transcription level reflects the overall activity of ica genes. Here, we reveled that icaA expression was poorly correlated with quantity of PIA produced for each strain. Dobinsky et al. (35) have noted that icaA transcriptional level is well associated with biofilm growth condition but it is clearly dissociated from the quantity of PIA produced. All data reported here make it impossible to rely on ica genes as molecular explanation for differences in the amount of PIA production.
The second targeted gene that we have assessed is ccpA gene. Based on the fact that this gene influences biofilm formation in S. epidermidis (11) and on the correlation of low metabolic activity with greater biofilm biomass, some studies have found the associating of low expression of ccpA gene with greater amount of PIA production (36, 37). Again as for icaA and ccpA gene, in this study there was no correlation between their transcriptional level and the recorded biofilm biomass. Discordance here may be explained by differences of used methodology between our work and that of Sousa et al. based on phenotypic assay and that of Al Laham based on examining mutant strains (36, 37).
RNAIII gene was the last targeted one during qRT-PCR experiments. As an effector of agr system, RNAIII regulates several genes involved in S. epidermidis biofilm formation and it contributes to cell density regulation via enhancement of bacterial detachment when bacterial population reaches a given threshold (10). In our experiment, we found that transcriptional level of RNAIII gene was inversely correlated with the amount of PIA produced by a given strain, so that, for a mature biofilm of 24 hours, strains with higher biofilm unit were those weaker in RNAIII gene expressing and vice versa. Dai et al. (38) has recently reported that S. epidermidis exhibiting down-regulation of RNAIII in mature biofilm are characterized by higher quantities of extracellular DNA and accelerated cell death arising from both significant rates of cell renewing and bigger biofilm microcolonies.
5.1. Conclusions
In conclusion, biofilm production in our S. epidermidis strains was mainly ica-dependent and biofilm biomass seemed to be downregulated by RNAIII gene.
References
-
1.
Otto M. Staphylococcus colonization of the skin and antimicrobial peptides. Expert Rev Dermatol. 2010;5(2):183-95. [PubMed ID: 20473345]. https://doi.org/10.1586/edm.10.6.
-
2.
Mack D, Davies AP, Harris LG, Jeeves R, Pascoe B, Knobloch JKM, et al. Staphylococcus epidermidis in Biomaterial-Associated Infections. In: Moriarty FT, Zaat AJS, Busscher JH, editors. Biomaterials Associated Infection: Immunological Aspects and Antimicrobial Strategies. New York: Springer; 2013. p. 25-56. https://doi.org/10.1007/978-1-4614-1031-7_2.
-
3.
Wu H, Moser C, Wang HZ, Hoiby N, Song ZJ. Strategies for combating bacterial biofilm infections. Int J Oral Sci. 2015;7(1):1-7. [PubMed ID: 25504208]. https://doi.org/10.1038/ijos.2014.65.
-
4.
Otto M. Staphylococcus epidermidis--the 'accidental' pathogen. Nat Rev Microbiol. 2009;7(8):555-67. [PubMed ID: 19609257]. https://doi.org/10.1038/nrmicro2182.
-
5.
Buttner H, Mack D, Rohde H. Structural basis of Staphylococcus epidermidis biofilm formation: mechanisms and molecular interactions. Front Cell Infect Microbiol. 2015;5:14. [PubMed ID: 25741476]. https://doi.org/10.3389/fcimb.2015.00014.
-
6.
Stevens NT, Greene CM, O'Gara JP, Humphreys H. Biofilm characteristics of Staphylococcus epidermidis isolates associated with device-related meningitis. J Med Microbiol. 2009;58(Pt 7):855-62. [PubMed ID: 19502363]. https://doi.org/10.1099/jmm.0.009209-0.
-
7.
Xue T, Ni J, Shang F, Chen X, Zhang M. Autoinducer-2 increases biofilm formation via an ica- and bhp-dependent manner in Staphylococcus epidermidis RP62A. Microbes Infect. 2015;17(5):345-52. [PubMed ID: 25637952]. https://doi.org/10.1016/j.micinf.2015.01.003.
-
8.
Arciola CR, Campoccia D, Ravaioli S, Montanaro L. Polysaccharide intercellular adhesin in biofilm: structural and regulatory aspects. Front Cell Infect Microbiol. 2015;5:7. [PubMed ID: 25713785]. https://doi.org/10.3389/fcimb.2015.00007.
-
9.
Meireles A, Borges A, Giaouris E, Simoes M. The current knowledge on the application of anti-biofilm enzymes in the food industry. Food Res Int. 2016;86:140-6. https://doi.org/10.1016/j.foodres.2016.06.006.
-
10.
Guldimann C, Boor KJ, Wiedmann M, Guariglia-Oropeza V. Resilience in the Face of Uncertainty: Sigma Factor B Fine-Tunes Gene Expression To Support Homeostasis in Gram-Positive Bacteria. Appl Environ Microbiol. 2016;82(15):4456-69. [PubMed ID: 27208112]. https://doi.org/10.1128/AEM.00714-16.
-
11.
Sadykov MR, Hartmann T, Mattes TA, Hiatt M, Jann NJ, Zhu Y, et al. CcpA coordinates central metabolism and biofilm formation in Staphylococcus epidermidis. Microbiology. 2011;157(Pt 12):3458-68. [PubMed ID: 21964732]. https://doi.org/10.1099/mic.0.051243-0.
-
12.
Stepanovic S, Vukovic D, Hola V, Di Bonaventura G, Djukic S, Cirkovic I, et al. Quantification of biofilm in microtiter plates: overview of testing conditions and practical recommendations for assessment of biofilm production by staphylococci. APMIS. 2007;115(8):891-9. [PubMed ID: 17696944]. https://doi.org/10.1111/j.1600-0463.2007.apm_630.x.
-
13.
Mekni MA, Bouchami O, Achour W, Ben Hassen A. Strong biofilm production but not adhesion virulence factors can discriminate between invasive and commensal Staphylococcus epidermidis strains. APMIS. 2012;120(8):605-11. [PubMed ID: 22779682]. https://doi.org/10.1111/j.1600-0463.2012.02877.x.
-
14.
Saffari F, Widerstrom M, Gurram BK, Edebro H, Hojabri Z, Monsen T. Molecular and Phenotypic Characterization of Multidrug-Resistant Clones of Staphylococcus epidermidis in Iranian Hospitals: Clonal Relatedness to Healthcare-Associated Methicillin-Resistant Isolates in Northern Europe. Microb Drug Resist. 2016;22(7):570-7. [PubMed ID: 26981847]. https://doi.org/10.1089/mdr.2015.0283.
-
15.
Christensen GD, Simpson WA, Bisno AL, Beachey EH. Adherence of slime-producing strains of Staphylococcus epidermidis to smooth surfaces. Infect Immun. 1982;37(1):318-26. [PubMed ID: 6179880].
-
16.
Arciola CR, Campoccia D, Gamberini S, Rizzi S, Donati ME, Baldassarri L, et al. Search for the insertion element IS256 within the ica locus of Staphylococcus epidermidis clinical isolates collected from biomaterial-associated infections. Biomaterials. 2004;25(18):4117-25. [PubMed ID: 15046902]. https://doi.org/10.1016/j.biomaterials.2003.11.027.
-
17.
Mack D, Riedewald J, Rohde H, Magnus T, Feucht HH, Elsner HA, et al. Essential functional role of the polysaccharide intercellular adhesin of Staphylococcus epidermidis in hemagglutination. Infect Immun. 1999;67(2):1004-8. [PubMed ID: 9916125].
-
18.
Qin Z, Yang X, Yang L, Jiang J, Ou Y, Molin S, et al. Formation and properties of in vitro biofilms of ica-negative Staphylococcus epidermidis clinical isolates. J Med Microbiol. 2007;56(Pt 1):83-93. [PubMed ID: 17172522]. https://doi.org/10.1099/jmm.0.46799-0.
-
19.
Ylioinas J, Hadid A, Hong X, Pietikäinen M. Age Estimation Using Local Binary Pattern Kernel Density Estimate. In: Petrosino A, editor. Image Analysis and Processing - ICIAP 2013: 17th International Conference, Naples, Italy. 8156. Berlin, Heidelberg: Springer Berlin Heidelberg; 2013. p. 141-50. https://doi.org/10.1007/978-3-642-41181-6_15.
-
20.
Kobayashi H, Oethinger M, Tuohy MJ, Procop GW, Bauer TW. Improved detection of biofilm-formative bacteria by vortexing and sonication: a pilot study. Clin Orthop Relat Res. 2009;467(5):1360-4. [PubMed ID: 18989729]. https://doi.org/10.1007/s11999-008-0609-5.
-
21.
Rao X, Huang X, Zhou Z, Lin X. An improvement of the 2^(-delta delta CT) method for quantitative real-time polymerase chain reaction data analysis. Biostat Bioinforma Biomath. 2013;3(3):71-85. [PubMed ID: 25558171].
-
22.
Begovic J, Jovcic B, Papic-Obradovic M, Veljovic K, Lukic J, Kojic M, et al. Genotypic diversity and virulent factors of Staphylococcus epidermidis isolated from human breast milk. Microbiol Res. 2013;168(2):77-83. [PubMed ID: 23098640]. https://doi.org/10.1016/j.micres.2012.09.004.
-
23.
Botelho AM, Nunes Z, Asensi MD, Gomes MZ, Fracalanzza SE, Figueiredo AM. Characterization of coagulase-negative staphylococci isolated from hospital indoor air and a comparative analysis between airborne and inpatient isolates of Staphylococcus epidermidis. J Med Microbiol. 2012;61(Pt 8):1136-45. [PubMed ID: 22516129]. https://doi.org/10.1099/jmm.0.035931-0.
-
24.
Mateo M, Maestre JR, Aguilar L, Gimenez MJ, Granizo JJ, Prieto J. Strong slime production is a marker of clinical significance in Staphylococcus epidermidis isolated from intravascular catheters. Eur J Clin Microbiol Infect Dis. 2008;27(4):311-4. [PubMed ID: 18095009]. https://doi.org/10.1007/s10096-007-0433-y.
-
25.
Widerstrom M, Carroll KC. Significance of Staphylococcus epidermidis in Health Care-Associated Infections, from Contaminant to Clinically Relevant Pathogen: This Is a Wake-Up Call!. J Clin Microbiol. 2016;54(7):1679-81. https://doi.org/10.1128/jcm.00743-16.
-
26.
Schaeffer CR, Woods KM, Longo GM, Kiedrowski MR, Paharik AE, Buttner H, et al. Accumulation-associated protein enhances Staphylococcus epidermidis biofilm formation under dynamic conditions and is required for infection in a rat catheter model. Infect Immun. 2015;83(1):214-26. [PubMed ID: 25332125]. https://doi.org/10.1128/IAI.02177-14.
-
27.
Dice B, Stoodley P, Buchinsky F, Metha N, Ehrlich GD, Hu FZ. Biofilm formation by ica-positive and ica-negative strains of Staphylococcus epidermidis in vitro. Biofouling. 2009;25(4):367-75. [PubMed ID: 19267282]. https://doi.org/10.1080/08927010902803297.
-
28.
Harris LG, Murray S, Pascoe B, Bray J, Meric G, Magerios L, et al. Biofilm Morphotypes and Population Structure among Staphylococcus epidermidis from Commensal and Clinical Samples. PLoS One. 2016;11(3):e0151240. [PubMed ID: 26978068]. https://doi.org/10.1371/journal.pone.0151240.
-
29.
Rohde H, Kalitzky M, Kroger N, Scherpe S, Horstkotte MA, Knobloch JK, et al. Detection of virulence-associated genes not useful for discriminating between invasive and commensal Staphylococcus epidermidis strains from a bone marrow transplant unit. J Clin Microbiol. 2004;42(12):5614-9. [PubMed ID: 15583290]. https://doi.org/10.1128/JCM.42.12.5614-5619.2004.
-
30.
Chessa D, Ganau G, Spiga L, Bulla A, Mazzarello V, Campus GV, et al. Staphylococcus aureus and Staphylococcus epidermidis Virulence Strains as Causative Agents of Persistent Infections in Breast Implants. PLoS One. 2016;11(1):e0146668. [PubMed ID: 26811915]. https://doi.org/10.1371/journal.pone.0146668.
-
31.
Arciola CR, Campoccia D, Speziale P, Montanaro L, Costerton JW. Biofilm formation in Staphylococcus implant infections. A review of molecular mechanisms and implications for biofilm-resistant materials. Biomaterials. 2012;33(26):5967-82. [PubMed ID: 22695065]. https://doi.org/10.1016/j.biomaterials.2012.05.031.
-
32.
Hennig S, Ziebuhr W. Characterization of the transposase encoded by IS256, the prototype of a major family of bacterial insertion sequence elements. J Bacteriol. 2010;192(16):4153-63. [PubMed ID: 20543074]. https://doi.org/10.1128/JB.00226-10.
-
33.
Bronesky D, Wu Z, Marzi S, Walter P, Geissmann T, Moreau K, et al. Staphylococcus aureus RNAIII and Its Regulon Link Quorum Sensing, Stress Responses, Metabolic Adaptation, and Regulation of Virulence Gene Expression. Annu Rev Microbiol. 2016;70:299-316. [PubMed ID: 27482744]. https://doi.org/10.1146/annurev-micro-102215-095708.
-
34.
Schommer NN, Christner M, Hentschke M, Ruckdeschel K, Aepfelbacher M, Rohde H. Staphylococcus epidermidis uses distinct mechanisms of biofilm formation to interfere with phagocytosis and activation of mouse macrophage-like cells 774A.1. Infect Immun. 2011;79(6):2267-76. [PubMed ID: 21402760]. https://doi.org/10.1128/IAI.01142-10.
-
35.
Dobinsky S, Kiel K, Rohde H, Bartscht K, Knobloch JK, Horstkotte MA, et al. Glucose-related dissociation between icaADBC transcription and biofilm expression by Staphylococcus epidermidis: evidence for an additional factor required for polysaccharide intercellular adhesin synthesis. J Bacteriol. 2003;185(9):2879-86. [PubMed ID: 12700267].
-
36.
Al Laham N, Rohde H, Sander G, Fischer A, Hussain M, Heilmann C, et al. Augmented expression of polysaccharide intercellular adhesin in a defined Staphylococcus epidermidis mutant with the small-colony-variant phenotype. J Bacteriol. 2007;189(12):4494-501. [PubMed ID: 17449620]. https://doi.org/10.1128/JB.00160-07.
-
37.
Sousa C, Teixeira P, Oliveira R. The role of extracellular polymers on Staphylococcus epidermidis biofilm biomass and metabolic activity. J Basic Microbiol. 2009;49(4):363-70. [PubMed ID: 19219902]. https://doi.org/10.1002/jobm.200800196.
-
38.
Dai L, Yang L, Parsons C, Findlay VJ, Molin S, Qin Z. Staphylococcus epidermidis recovered from indwelling catheters exhibit enhanced biofilm dispersal and "self-renewal" through downregulation of agr. BMC Microbiol. 2012;12:102. [PubMed ID: 22682058]. https://doi.org/10.1186/1471-2180-12-102.
-
39.
Ziebuhr W, Krimmer V, Rachid S, Lossner I, Gotz F, Hacker J. A novel mechanism of phase variation of virulence in Staphylococcus epidermidis: evidence for control of the polysaccharide intercellular adhesin synthesis by alternating insertion and excision of the insertion sequence element IS256. Mol Microbiol. 1999;32(2):345-56. [PubMed ID: 10231490].
-
40.
de Araujo GL, Coelho LR, de Carvalho CB, Maciel RM, Coronado AZ, Rozenbaum R, et al. Commensal isolates of methicillin-resistant Staphylococcus epidermidis are also well equipped to produce biofilm on polystyrene surfaces. J Antimicrob Chemother. 2006;57(5):855-64. [PubMed ID: 16551694]. https://doi.org/10.1093/jac/dkl071.
-
41.
Nilsson M, Frykberg L, Flock JI, Pei L, Lindberg M, Guss B. A fibrinogen-binding protein of Staphylococcus epidermidis. Infect Immun. 1998;66(6):2666-73. [PubMed ID: 9596732].
-
42.
Williams RJ, Henderson B, Sharp LJ, Nair SP. Identification of a fibronectin-binding protein from Staphylococcus epidermidis. Infect Immun. 2002;70(12):6805-10. [PubMed ID: 12438356].
-
43.
Gu J, Li H, Li M, Vuong C, Otto M, Wen Y, et al. Bacterial insertion sequence IS256 as a potential molecular marker to discriminate invasive strains from commensal strains of Staphylococcus epidermidis. J Hosp Infect. 2005;61(4):342-8. [PubMed ID: 16242209]. https://doi.org/10.1016/j.jhin.2005.04.017.
-
44.
Li M, Wang X, Gao Q, Lu Y. Molecular characterization of Staphylococcus epidermidis strains isolated from a teaching hospital in Shanghai, China. J Med Microbiol. 2009;58(Pt 4):456-61. [PubMed ID: 19273641]. https://doi.org/10.1099/jmm.0.007567-0.
-
45.
Pintens V, Massonet C, Merckx R, Vandecasteele S, Peetermans WE, Knobloch JK, et al. The role of sigmaB in persistence of Staphylococcus epidermidis foreign body infection. Microbiology. 2008;154(Pt 9):2827-36. [PubMed ID: 18757816]. https://doi.org/10.1099/mic.0.2007/015768-0.
-
46.
Frebourg NB, Lefebvre S, Baert S, Lemeland JF. PCR-Based assay for discrimination between invasive and contaminating Staphylococcus epidermidis strains. J Clin Microbiol. 2000;38(2):877-80. [PubMed ID: 10655405].
-
47.
Vandecasteele SJ, Peetermans WE, Merckx R, Van Eldere J. Quantification of expression of Staphylococcus epidermidis housekeeping genes with Taqman quantitative PCR during in vitro growth and under different conditions. J Bacteriol. 2001;183(24):7094-101. [PubMed ID: 11717267]. https://doi.org/10.1128/JB.183.24.7094-7101.2001.