Abstract
Background:
Outer membrane protein D (PD) is a highly conserved and stable protein in the outer membrane of both encapsulated (typeable) and non-capsulated (non-typeable) strains of Haemophilus influenzae. As an immunogen, PD is a potential candidate vaccine against non-typeable H. influenzae (NTHi) strains.Objectives:
The aim of this study was to determine the cytokine pattern and the opsonic antibody response in a BALB/c mouse model versus PD from NTHi as a vaccine candidate.Methods:
Protein D was formulated with Freund’s and outer membrane vesicle (OMV) adjuvants and injected into experimental mice. Sera from all groups were collected. The bioactivity of the anti-PD antibody was determined by opsonophagocytic killing test. To evaluate the cytokine responses, the spleens were assembled, suspension of splenocytes was recalled with antigen, and culture supernatants were analyzed by ELISA for IL-4, IL-10, and IFN-γ cytokines.Results:
Anti-PD antibodies promoted phagocytosis of NTHi in both immunized mice groups (those administered PD + Freund’s and those administered PD + OMV adjuvants, 92.8% and 83.5%, respectively, compared to the control group). In addition, the concentrations of three cytokines were increased markedly in immunized mice.Conclusions:
We conclude that immunization with PD protects mice against NTHi. It is associated with improvements in both cellular and humoral immune responses and opsonic antibody activity.Keywords
Freund’s Adjuvant OMV Adjuvant Protein D Non-Typeable Haemophilus influenzae
1. Background
Haemophilus influenzae is a pleomorphic, Gram-negative bacterium that causes disease exclusively in humans (1). Non-typeable Haemophilus influenzae (NTHi) lacks a poly saccharide capsule (distinguishing it from encapsulated forms, such as type b) and is present as a commensal in the pharynx of up to 75% of healthy adults. It is also a major cause of respiratory tract infections in both developed and non-industrialized countries (2). The success of this organism as a commensal and a pathogen is due to its ability to attach to the mucous membranes and to adapt by varying both its proteins and its enzymes in response to host defense mechanisms (3). In the context of infections, it has the capacity to live intracellularly, particularly in macrophages (4, 5). A different set of challenges to developing a vaccine against NTHi are the antigenic heterogeneity of several of its major surface antigens and the genetic heterogeneity among strains.
Non-typeable Haemophilus influenzae strains express multiple outer membrane proteins (OMPs). Several of the OMPs from NTHi have been isolated, characterized, and eliminated as vaccine candidates because of variable expression, epitope heterogeneity, or other specifications (6). At this time, one OMP of NTHi protein D (PD), an approximate 42-kDa lipoprotein is a highly conserved lipoprotein that is present in both NTHi and type b H. influenzae strains (7). Protein D is not by description an adhesion; however, it indirectly promotes bacterial adhesion and invasion due to glycerophosphodiester phosphodiesterase (GlpQ) activity, and it has also been proven to promote bacterial internalization into human monocytes (8-11).
Due to the properties of PD such as surface localization, antigenic conservation, and pathogenicity it is currently being considered as a vaccine candidate against infections caused by NTHi, and it has demonstrated notable efficacy in preventing infections of H. influenzae (12). So far, the adaptive immune response to PD has not been well defined. The adaptive immune response is mediated by T-helpers, and the relative amounts of IFNγ and IL4 are indicators of a predominantly Th1 and Th2 response, respectively. Adaptive immune responses also result in the production of immunoglobulin G (IgG) (13).
2. Objectives
In the present study, PD adjuvanted with Freund’s and OMV was injected into the experimental mice. Freund’s adjuvant is a solution of antigen emulsified in mineral oil and used as an immunopotentiator (booster), and OMV is an adjuvant of microbial origin, which is produced by Gram-negative organisms during growth (14, 15). To characterize the immune response, we measured the bioactivity of anti-PD antibody in sera and the production of cytokines in the splenocytes of the immunized mice.
3. Methods
3.1. Bacterial Strain
The NTHi standard strain ATCC 49766 was used in this study. This strain was prepared at the Pasteur institute of Iran (Tehran, Iran) and was used for opsonophagocytic assays as a source of antigen. Strain 49766 was cultured on chocolate agar plates and incubated at 37°C and 5% CO2 for 24 hours.
3.2. Protein D
In a previous study, PD from NTHi standard strain ATCC 49766 was cloned, expressed, and purified (Forthcoming).
3.3. Experimental Groups and Immunization Procedures
Six to eight-week-old female BALB/c mice (weighing 18 - 20 g) were obtained from the breeding stock maintained at the Razi vaccine and serum research institute of Karaj, Iran. Mice were housed for one week before the experiments and given free access to food and water. All experiments were in accordance with the animal care and use protocol of the Pasteur institute of Iran. These inbred mice were assigned into three different groups. Each group contained five mice, as described below:
- Group I: injection of 50 μg of the PD emulsified in Freund adjuvant,
- Group II: injection of 25 μg of the PD with 25 μg of OMV,
- Group III: PBS (control group).
The booster injections were administered on the 14th and 28th days following the first injection via subcutaneous route. The immunized mice were bled on days 14, 28, and 42. The immune sera were separated, pooled, and kept at -20°C until further experiments.
3.4. Opsonophagocytic Assay
The test was performed according to the method of Pier et al., with some modifications (16). Briefly, a bacterial suspension (standard NTHi strain) was prepared at an approximate concentration of 2 × 107 CFUs/mL in 1% BSA (Santacruz). Mouse macrophages were used at a final concentration of 2 × 107 cell/ml in complete RPMI-1640 (Gibco). Baby rabbit serum (Pasteur Institute, Tehran, Iran) was used as a complement source. Three different dilutions (1:4, 1:8, and 1:16) of the pooled sera of each group were used. The complement of the experimental sera was inactivated by heating at 56°C for 30 minutes. For the opsonophagocytic assay, at the first, we incubated 100 μL of bacteria (2 × 107 cells per well) with an equal volume of diluted mouse serum at 22°C for 90 minutes, then rinsed twice with PBS + BSA 1% for elimination of excess antibodies.
Following suspension with 200 μL PBS + BSA, 100 μL from the mouse macrophage and the complement were mixed in a sterile 48-well plate and then incubated on a shaker at 37°C for 90 minutes. Soon thereafter (time 0) and after 90 minutes, 25 ml of the sample was removed and diluted in 225 μL of saline. Finally, 10 μL from the samples was plated on chocolate agar medium for bacterial enumeration. The plates were incubated overnight at 37°C. Normal mouse serum (NMS) and normal rabbit serum (NRS) (in a 1:4 dilution) were used as a pre-immune serum (control). The opsonic killing activity of immune sera was statistically compared to pre-immune sera. This experiment was performed in duplicate for each quantity. The percent kill was calculated as follows: Opsonophagocytosis (%) = [1-(CFU of immune serum/CFU of pre-immune serum)] × 100.
3.5. Splenocytes Culture and in Vitro Immunization
Mice were sacrificed at day 42, and their spleens were collected. Splenocytes were isolated for enzyme-linked immunosorbent assay (ELISA), and cytokine production was analyzed. Spleens were harvested in RPMI-1640 medium and minced, and RBCs (red blood cells) were lysed with Tris-buffered ammonium chloride. The remaining cell pellets, representing the total splenic mononuclear cell population, were re-suspended in complete RPMI-1640 medium. These were seeded in duplicate (3 × 105 cells per well) in a 96-well flat-bottomed plate (BD Biosciences) and were stimulated separately with specific antigen (PD; 10 μg/mL) and non-specific mitogen Concanavalin A (Con-A; 5 μg/mL) as a positive control. The plates were incubated at 37°C in 5% CO2 for 72 hours, and then the culture supernatant was harvested and stored at -70°C until use.
3.6. Cytokine Patterns on Splenocyte Culture Supernatant
To examine the level of the Th1-type cytokine (IFN-γ) and Th2-type cytokines (IL-4 and IL-10), commercially available quantitative ELISA assay kits (mouse IFN-γ, IL-4, and IL-10 kits, eBioscience-BMS606-BMS613-BMS614, USA) were used according to the manufacturer’s protocol. Briefly, standards or samples were added to microtiter plates coated with a monoclonal antibody specific to the cytokine of interest, and plates were incubated for two hours at room temperature. After washing, 100 μL of Streptavidine-HRP (horse-radish peroxidase) was added to each well, and the plates were incubated for one hour and washed. A substrate solution (TMB) was then added, and the plates were incubated for 20 minutes. The reaction was terminated by the addition of stop solution, and optical density was read at 450 nm. Cytokine concentration was calculated as pg/ml according to standard curves.
3.7. Statistical Analysis
All experiments were performed in duplicate, and the data was expressed as means ± of each experiment. Statistical analyses were carried out by one-way analysis of variance (ANOVA), which was used to compare the differences between the mean values of experimental groups using Prism Software. P values less than 0.05 were considered statistically significant.
4. Results
4.1. Opsonophagocytic Killing Activity of Antisera to Protein D Against Non-Typeable H. influenzae
In order to determine the functional activity of the anti-PD antibody in vitro, its ability to promote phagocytosis of NTHi was evaluated by incubating NTHi strain ATCC 49766 with different dilutions of antiserum and healthy mouse macrophages in the presence of rabbit complement. Sera obtained from immunized groups of mice with PD were analyzed by the opsonophagocytic killing test and compared to sera from the control group. In the presence of normal mouse serum (control group), the number of viable bacterial cells slightly (9.09%) decreased, and limited opsonophagocytic killing activity was observed. The results of the opsonophagocytic assay for antibodies against NTHi are shown in Figure 1. The level of opsonophagocytic activity against NTHi in the control group was significantly lower than that of immunized groups (P < 0.05). Clear booster effects were seen after the second injection on day 14, and strong booster effects were seen after the third injection on day 28.
The opsonophagocytic Killing Activity of the Best Dilution (1/4) from Sera
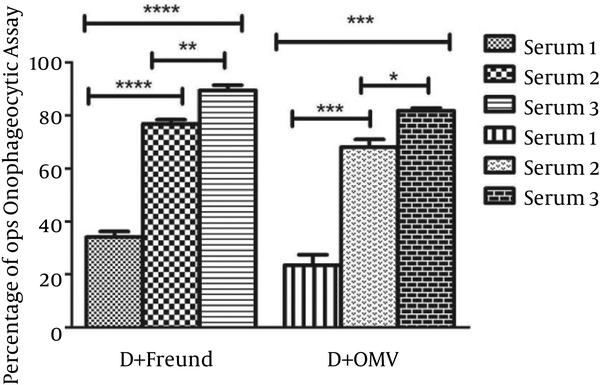
The anti-PD + Freund’s adjuvant antibody (group I) promoted phagocytosis of NTHi, and the number of viable bacterial cells decreased over 80%, whereas this value was over 70% for the anti-PD + OMV adjuvant antibody group (group II) after 90 minutes in serum 3 (P = 0.0262). The opsonophagocytic killing activity of anti-PD + Freund’s adjuvant antibody on day 42 (serum 3) was higher than that of serum 1 and serum 2 (P = 0.0001 and P = 0.0076, respectively). In addition, the bioactivity of opsonin antibody in serum 2 was higher than in serum 1 in the same group (P = 0.0001). The opsonophagocytic activity of the anti-PD + OMV adjuvant antibody in serum 3 was greater than that of serum 1 and serum 2 (P = 0.0001 and P = 0.0114, respectively). In addition, the level of opsonophagocytosis in serum 2 was slightly higher than in serum 1 (P = 0.0008). Nevertheless, there was no statistically significant difference among the groups in terms of serum 1 and serum 2 (P > 0.05). As a result, these data indicate that anti-PD antibody acts as a potent opsonic factor for killing of NTHi and protects the body against infections caused by H. influenzae.
4.2. Cytokine Responses
Quantitative ELISA assays were applied to detect IFN-γ, IL-4, and IL-10 cytokines secreted by splenocytes of the immunized mice upon recall with PD for three days. In comparison with the negative control group, the level of cytokines increased markedly in the experimental groups following stimulation with PD (P = 0.0001; Figure 2). There was a significant difference in the production of IFN-γ in response to the non-specific mitogen Con-A and PD in both immunized groups (P = 0.0001). The production rate of IFN-γ secreted by splenocytes following stimulation with PD in group I (PD + Freund’s adjuvant) was higher than the rate in group II (PD + OMV adjuvant) (P = 0.0023; Figure 2A).
A significant difference was observed in the secretion of IL-4 in response to the non-specific mitogen Con-A and PD in group I and group II (P = 0.0068 and P = 0.0001, respectively). The production of IL-4 from splenocytes stimulated with PD in group I was higher than in group II (P = 0.0035; Figure 2B). A considerable difference was seen in the production of IL-10 in response to the non-specific mitogen Con-A and PD in group I and group II (P = 0.0302 and P = 0.0001, respectively). In addition, the production of IL-10 was higher in group I than in group II (P = 0.0001; Figure 2C).
In Vitro IFN-γ, IL-4, and IL-10 Production by Splenocytes of Immunized Mice with Protein D
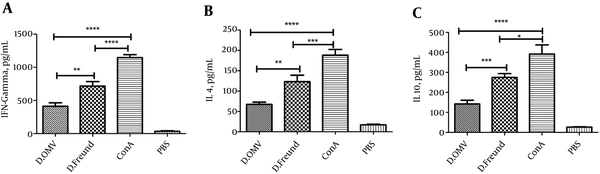
5. Discussion
Non-typeable Haemophilus influenzae is commonly found in the pharyngeal flora of children and adults. In addition to its role as a commensal, NTHi is a major cause of respiratory tract infections in children such as acute otitis media (AOM), acute sinusitis, and pneumonia especially in developing countries (17). In addition, NTHi is implicated in chronic infections of the lower respiratory tract in adults, such as chronic obstructive pulmonary disease (COPD) and chronic bronchitis (18-22). NTHi does not express a capsule; for this reason, research for optional vaccine candidates has been focused on outer membrane proteins (23). Several NTHi outer membrane proteins continue to be investigated as possible vaccine antigens, but most of these antigens have no effect against infections caused by NTHi and other encapsulated serotypes (6, 24, 25). To produce a broad-spectrum vaccine against infections caused by NTHi, we utilized the outer membrane PD from NTHi as protein antigen (26).
Protein D expression is important to NTHi virulence in the respiratory tract, as shown in both in vitro and in vivo experiments. In a rat model of otitis media, a PD-deficient strain demonstrated 100-fold decreased virulence in comparison to the PD-expressing strain (8). Moreover, a PD-expressing strain was shown to cause considerably higher damage to cilia in a human nasopharyngeal ex vivo tissue culture model than a PD-deficient strain (27). In particular, the protective efficacy of PD was demonstrated by employing models of otitis (chinchilla model of otitis media) (28, 29). Protein D was even selected to serve as an active carrier protein for HBsAg against NTHi (30). An 11-valent pneumococcal polysaccharide vaccine with a PD carrier demonstrated protection against both vaccine-type pneumococcal otitis and disease due to NTHi (31).
These clinical studies failed to demonstrate a clear correlation between serum antibody levels and PD immunization in children. A functional assay measuring inhibition of PD phosphodiesterase activity in serum samples of children immunized with the PD conjugate vaccine was described, but an imperfect correlation was noted between serum PD antibody levels and functional antibody activation (7, 32, 33). In this study, we used PD with two adjuvants (OMV and Freund), which was injected into mice at 0, 14, and 28 days according to the protocol. We then analyzed the opsonophagocytic activity of the antibody and levels of IL-4, IL-10, and IFN-γ. Cytokines play a considerable role in immune responses against NTHi. IL-4 and IL-10 modulate the differentiation toward Th2 responses.
Humoral immunity responses are activated by Th2 and produce specific antibodies. These antibodies are increased steadily by the production of these cytokines (34). In this test, we have seen a small amount of opsonophagocytosis activity (30%) in sera of experimental groups at two weeks after the first injection (serum 1). We also demonstrated that booster immunization can increase opsonophagocytic activity from 30% (serum 1) to 90% (serum 3). The growth of IL-4 and IL-10 cytokines in the culture supernatants following stimulation with PD also proved this enhancement in opsonic antibody and opsonophagocytic killing activity percentage. In a previous study, the PD of NTHi has been demonstrated to induce humoral immune responses (Forthcoming). As a result, we can say that humoral immunity increased when PD was administered with adjuvants.
Non-typeable Haemophilus influenzae can reside within macrophages and epithelial cells, and macrophages are also vital to the binding and phagocytosis of NTHi; thus, it is conceivable that protective immunity towards NTHi employs immune strategies similar to those against other intracellular respiratory pathogens (35-37). The antigen-specific T-cell responses are supported for a longer period than the associated antibody titres, emphasizing the importance of cell-mediated responses in protective immunity against intracellular pathogens. Cell-mediated immunity or T-cell immunity is an immune response that activates macrophages, natural killer cells, and T-lymphocytes, enabling them to destroy pathogens and stimulating the cells to secrete a variety of cytokines in response to an antigen (38-40). CD4+ T-cells comprise functionally distinct populations characterized by specific cytokine profiles produced in response to antigens (41-43).
The presence of IFN-γ is highly indicative of Th1 response (44). The IFN-γ cytokine enhances the expression of co-stimulatory molecules to aid recognition by T-cells and regulates the differentiation to Th1 responses (45, 46). This cytokine stimulates production isotypes of antibodies, such as IgG2a, in mice that activate the complement system, macrophages, and subsequent opsonophagocytosis activity (47-49). King PT suggested that CTL and NK cell responses may be important in preventing infection caused by NTHi, and levels of IFN-γ were significantly higher in the control group than in bronchiectasis patients. Thus, this cytokine may play an important role in preventing infection from NTHi (50).
In this study, an increase in IFN-γ cytokine in the culture supernatants following stimulation with PD demonstrated the augmentation of opsonic antibody and opsonophagocytosis activity in sera. In addition, an increase in IFN-γ elevates the Th1 responses and creates the cellular immunity response. In this research, we evaluated the functional activity of the antibodies generated following injection of PD with adjuvants already reported to have protective properties in mouse models, and we demonstrated a fundamental immunological role for PD in protection against NTHi via production of opsonic antibodies. Production of opsonic antibodies is important for both innate and acquired immunity in the infections caused by NTHi, and it plays a vital role in opsonophagocytic activity (51, 52). Our data propose that cytokine responses to NTHi can be incremented to promote protective cell-mediated immune responses and that they facilitate the interaction between humoral and cellular immunity. We also suggest that both Th1 and Th2 immune responses were induced and that opsonophagocytic killing activity increased following inoculation.
The main finding of this study is that PD can enhance NTHi-specific cell mediated immune responses and protective responses through antibody production in mice. These results confirm the possibility of using PD as a protein carrier for other antigens. Future research may recognize other immunogenic proteins of NTHi that function better than PD. Identifying the specific cytokines that provide protection from infections of NTHi and developing effective vaccines against NTHi will be important for the prevention of infections.
Acknowledgements
References
-
1.
Sukupolvi-Petty S, Grass S, St Geme J3. The Haemophilus influenzae Type b hcsA and hcsB gene products facilitate transport of capsular polysaccharide across the outer membrane and are essential for virulence. J Bacteriol. 2006;188(11):3870-7. [PubMed ID: 16707679]. https://doi.org/10.1128/JB.01968-05.
-
2.
Murphy TF, Faden H, Bakaletz LO, Kyd JM, Forsgren A, Campos J, et al. Nontypeable Haemophilus influenzae as a pathogen in children. Pediatr Infect Dis J. 2009;28(1):43-8. [PubMed ID: 19057458]. https://doi.org/10.1097/INF.0b013e318184dba2.
-
3.
St Geme J3. Insights into the mechanism of respiratory tract colonization by nontypable Haemophilus influenzae. Pediatr Infect Dis J. 1997;16(10):931-5. [PubMed ID: 9380466].
-
4.
Avadhanula V, Rodriguez CA, Ulett GC, Bakaletz LO, Adderson EE. Nontypeable Haemophilus influenzae adheres to intercellular adhesion molecule 1 (ICAM-1) on respiratory epithelial cells and upregulates ICAM-1 expression. Infect Immun. 2006;74(2):830-8. [PubMed ID: 16428725]. https://doi.org/10.1128/IAI.74.2.830-838.2006.
-
5.
Langereis JD, Zomer A, Stunnenberg HG, Burghout P, Hermans PW. Nontypeable Haemophilus influenzae carbonic anhydrase is important for environmental and intracellular survival. J Bacteriol. 2013;195(12):2737-46. [PubMed ID: 23564172]. https://doi.org/10.1128/JB.01870-12.
-
6.
Barenkamp SJ, Munson RJ, Granoff DM. Outer membrane protein and biotype analysis of pathogenic nontypable Haemophilus influenzae. Infect Immun. 1982;36(2):535-40. [PubMed ID: 6979511].
-
7.
Forsgren A, Riesbeck K, Janson H. Protein D of Haemophilus influenzae: a protective nontypeable H. influenzae antigen and a carrier for pneumococcal conjugate vaccines. Clin Infect Dis. 2008;46(5):726-31. [PubMed ID: 18230042]. https://doi.org/10.1086/527396.
-
8.
Janson H, Melhus A, Hermansson A, Forsgren A. Protein D, the glycerophosphodiester phosphodiesterase from Haemophilus influenzae with affinity for human immunoglobulin D, influences virulence in a rat otitis model. Infect Immun. 1994;62(11):4848-54. [PubMed ID: 7927765].
-
9.
Janson H, Ruan M, Forsgren A. Limited diversity of the protein D gene (hpd) among encapsulated and nonencapsulated Haemophilus influenzae strains. Infect Immun. 1993;61(11):4546-52. [PubMed ID: 8104899].
-
10.
Ahren IL, Janson H, Forsgren A, Riesbeck K. Protein D expression promotes the adherence and internalization of non-typeable Haemophilus influenzae into human monocytic cells. Microb Pathog. 2001;31(3):151-8. [PubMed ID: 11500100]. https://doi.org/10.1006/mpat.2001.0456.
-
11.
Fan X, Goldfine H, Lysenko E, Weiser JN. The transfer of choline from the host to the bacterial cell surface requires glpQ in Haemophilus influenzae. Mol Microbiol. 2001;41(5):1029-36. [PubMed ID: 11555284].
-
12.
Song XM, Forsgren A, Janson H. The gene encoding protein D (hpd) is highly conserved among Haemophilus influenzae type b and nontypeable strains. Infect Immun. 1995;63(2):696-9. [PubMed ID: 7822043].
-
13.
Constant SL, Bottomly K. Induction of Th1 and Th2 CD4+ T cell responses: the alternative approaches. Annu Rev Immunol. 1997;15:297-322. [PubMed ID: 9143690]. https://doi.org/10.1146/annurev.immunol.15.1.297.
-
14.
Moshiri A, Dashtbani-Roozbehani A, Najar Peerayeh S, Siadat SD. Outer membrane vesicle: a macromolecule with multifunctional activity. Hum Vaccin Immunother. 2012;8(7):953-5. [PubMed ID: 22699443]. https://doi.org/10.4161/hv.20166.
-
15.
Pritsch M, Ben-Khaled N, Chaloupka M, Kobold S, Berens-Riha N, Peter A, et al. Comparison of Intranasal Outer Membrane Vesicles with Cholera Toxin and Injected MF59C.1 as Adjuvants for Malaria Transmission Blocking Antigens AnAPN1 and Pfs48/45. J Immunol Res. 2016;2016:3576028. [PubMed ID: 27239480]. https://doi.org/10.1155/2016/3576028.
-
16.
Ames P, DesJardins D, Pier GB. Opsonophagocytic killing activity of rabbit antibody to Pseudomonas aeruginosa mucoid exopolysaccharide. Infect Immun. 1985;49(2):281-5. [PubMed ID: 3160660].
-
17.
Langereis JD, de Jonge MI. Invasive Disease Caused by Nontypeable Haemophilus influenzae. Emerg Infect Dis. 2015;21(10):1711-8. [PubMed ID: 26407156]. https://doi.org/10.3201/eid2110.150004.
-
18.
Ishiwada N, Kaneko S, Aizawa J, Kuroki H, Kohno Y. [The frequency of non-typeable Haemophilus influenzae systemic disease in children]. Kansenshogaku Zasshi. 2003;77(1):1-4. [PubMed ID: 12638254].
-
19.
Murphy TF. Respiratory infections caused by non-typeable Haemophilus influenzae. Curr Opin Infect Dis. 2003;16(2):129-34. [PubMed ID: 12734445]. https://doi.org/10.1097/01.aco.0000065079.06965.e0.
-
20.
Finney LJ, Ritchie A, Pollard E, Johnston SL, Mallia P. Lower airway colonization and inflammatory response in COPD: a focus on Haemophilus influenzae. Int J Chron Obstruct Pulmon Dis. 2014;9:1119-32. [PubMed ID: 25342897]. https://doi.org/10.2147/COPD.S54477.
-
21.
King PT, Sharma R. The Lung Immune Response to Nontypeable Haemophilus influenzae (Lung Immunity to NTHi). J Immunol Res. 2015;2015:706376. [PubMed ID: 26114124]. https://doi.org/10.1155/2015/706376.
-
22.
Cerquetti M, Giufre M. Why we need a vaccine for non-typeable Haemophilus influenzae. Hum Vaccin Immunother. 2016:1-5. [PubMed ID: 27171854]. https://doi.org/10.1080/21645515.2016.1174354.
-
23.
Murphy TF, Dudas KC, Mylotte JM, Apicella MA. A subtyping system for nontypable Haemophilus influenzae based on outer-membrane proteins. J Infect Dis. 1983;147(5):838-46. [PubMed ID: 6601684].
-
24.
Turk DC. The pathogenicity of Haemophilus influenzae. J Med Microbiol. 1984;18(1):1-16. [PubMed ID: 6146721]. https://doi.org/10.1099/00222615-18-1-1.
-
25.
Murphy TF. Vaccines for Nontypeable Haemophilus influenzae: the Future Is Now. Clin Vaccine Immunol. 2015;22(5):459-66. [PubMed ID: 25787137]. https://doi.org/10.1128/CVI.00089-15.
-
26.
Behrouzi A, Bouzari S, Siadat SD, Jafari A, Irani S. Molecular Cloning, Expression and Purification of Truncated hpd Fragment of Haemophilus influenzae in Escherichia coli. Jundishapur J Microbiol. 2015;8(8). ee23218. [PubMed ID: 26464772]. https://doi.org/10.5812/jjm.23218.
-
27.
Janson H, Carl n B, Cervin A, Forsgren A, Magnusdottir AB, Lindberg S, et al. Effects on the ciliated epithelium of protein D-producing and -nonproducing nontypeable Haemophilus influenzae in nasopharyngeal tissue cultures. J Infect Dis. 1999;180(3):737-46. [PubMed ID: 10438362]. https://doi.org/10.1086/314921.
-
28.
Green BA, Vazquez ME, Zlotnick GW, Quigley-Reape G, Swarts JD, Green I, et al. Evaluation of mixtures of purified Haemophilus influenzae outer membrane proteins in protection against challenge with nontypeable H. influenzae in the chinchilla otitis media model. Infect Immun. 1993;61(5):1950-7. [PubMed ID: 8478084].
-
29.
Hong W, Peng D, Rivera M, Gu XX. Protection against nontypeable Haemophilus influenzae challenges by mucosal vaccination with a detoxified lipooligosaccharide conjugate in two chinchilla models. Microbes Infect. 2010;12(1):11-8. [PubMed ID: 19782149]. https://doi.org/10.1016/j.micinf.2009.09.006.
-
30.
Su Q, Yi Y, Qiu F, Lu X, Ding J, Jia Z, et al. Immune responses to HBsAg conjugated to protein D of non-typeable Haemophilus influenzae in mice. PLoS One. 2015;10(2):e0117736. [PubMed ID: 25689855]. https://doi.org/10.1371/journal.pone.0117736.
-
31.
Nurkka A, Joensuu J, Henckaerts I, Peeters P, Poolman J, Kilpi T, et al. Immunogenicity and safety of the eleven valent pneumococcal polysaccharide-protein D conjugate vaccine in infants. Pediatr Infect Dis J. 2004;23(11):1008-14. [PubMed ID: 15545855].
-
32.
Prymula R, Kriz P, Kaliskova E, Pascal T, Poolman J, Schuerman L. Effect of vaccination with pneumococcal capsular polysaccharides conjugated to Haemophilus influenzae-derived protein D on nasopharyngeal carriage of Streptococcus pneumoniae and H. influenzae in children under 2 years of age. Vaccine. 2009;28(1):71-8. [PubMed ID: 19818722]. https://doi.org/10.1016/j.vaccine.2009.09.113.
-
33.
Prymula R, Peeters P, Chrobok V, Kriz P, Novakova E, Kaliskova E, et al. Pneumococcal capsular polysaccharides conjugated to protein D for prevention of acute otitis media caused by both Streptococcus pneumoniae and non-typable Haemophilus influenzae: a randomised double-blind efficacy study. Lancet. 2006;367(9512):740-8. [PubMed ID: 16517274]. https://doi.org/10.1016/S0140-6736(06)68304-9.
-
34.
Koedel U, Bernatowicz A, Frei K, Fontana A, Pfister HW. Systemically (but not intrathecally) administered IL-10 attenuates pathophysiologic alterations in experimental pneumococcal meningitis. J Immunol. 1996;157(11):5185-91. [PubMed ID: 8943431].
-
35.
Craig JE, Cliffe A, Garnett K, High NJ. Survival of nontypeable Haemophilus influenzae in macrophages. FEMS Microbiol Lett. 2001;203(1):55-61. [PubMed ID: 11557140].
-
36.
Williams AE, Maskell DJ, Moxon ER. Relationship between intracellular survival in macrophages and virulence of Haemophilus influenzae type b. J Infect Dis. 1991;163(6):1366-9. [PubMed ID: 2037802].
-
37.
Clementi CF, Hakansson AP, Murphy TF. Internalization and trafficking of nontypeable Haemophilus influenzae in human respiratory epithelial cells and roles of IgA1 proteases for optimal invasion and persistence. Infect Immun. 2014;82(1):433-44. [PubMed ID: 24218477]. https://doi.org/10.1128/IAI.00864-13.
-
38.
Defrance T, Taillardet M, Genestier L. T cell-independent B cell memory. Curr Opin Immunol. 2011;23(3):330-6. [PubMed ID: 21482090]. https://doi.org/10.1016/j.coi.2011.03.004.
-
39.
Gollob JA, Li J, Kawasaki H, Daley JF, Groves C, Reinherz EL, et al. Molecular interaction between CD58 and CD2 counter-receptors mediates the ability of monocytes to augment T cell activation by IL-12. J Immunol. 1996;157(5):1886-93. [PubMed ID: 8757306].
-
40.
Mond JJ, Vos Q, Lees A, Snapper CM. T cell independent antigens. Curr Opin Immunol. 1995;7(3):349-54. [PubMed ID: 7546399].
-
41.
Fietta P, Delsante G. The effector T helper cell triade. Riv Biol. 2009;102(1):61-74. [PubMed ID: 19718623].
-
42.
McKinstry KK, Strutt TM, Swain SL. The potential of CD4 T-cell memory. Immunology. 2010;130(1):1-9. [PubMed ID: 20331470]. https://doi.org/10.1111/j.1365-2567.2010.03259.x.
-
43.
Sharma SK, Pichichero ME. Cellular immune response in young children accounts for recurrent acute otitis media. Curr Allergy Asthma Rep. 2013;13(5):495-500. [PubMed ID: 24022464]. https://doi.org/10.1007/s11882-013-0370-z.
-
44.
de Bree GJ, Daniels H, Schilfgaarde M, Jansen HM, Out TA, van Lier RA, et al. Characterization of CD4+ memory T cell responses directed against common respiratory pathogens in peripheral blood and lung. J Infect Dis. 2007;195(11):1718-25. [PubMed ID: 17471443]. https://doi.org/10.1086/517612.
-
45.
Iwasaki A, Medzhitov R. Control of adaptive immunity by the innate immune system. Nat Immunol. 2015;16(4):343-53. [PubMed ID: 25789684]. https://doi.org/10.1038/ni.3123.
-
46.
Palm NW, Medzhitov R. Pattern recognition receptors and control of adaptive immunity. Immunol Rev. 2009;227(1):221-33. [PubMed ID: 19120487]. https://doi.org/10.1111/j.1600-065X.2008.00731.x.
-
47.
King PT, Hutchinson PE, Johnson PD, Holmes PW, Freezer NJ, Holdsworth SR. Adaptive immunity to nontypeable Haemophilus influenzae. Am J Respir Crit Care Med. 2003;167(4):587-92. [PubMed ID: 12433671]. https://doi.org/10.1164/rccm.200207-728OC.
-
48.
Klug-Micu GM, Stenger S, Sommer A, Liu PT, Krutzik SR, Modlin RL, et al. CD40 ligand and interferon-gamma induce an antimicrobial response against Mycobacterium tuberculosis in human monocytes. Immunology. 2013;139(1):121-8. [PubMed ID: 23289765]. https://doi.org/10.1111/imm.12062.
-
49.
Staitieh BS, Egea EE, Fan X, Azih N, Neveu W, Guidot DM. Activation of Alveolar Macrophages with Interferon-gamma Promotes Antioxidant Defenses via the Nrf2-ARE Pathway. J Clin Cell Immunol. 2015;6(5). [PubMed ID: 26779387]. https://doi.org/10.4172/2155-9899.1000365.
-
50.
King PT, Ngui J, Farmer MW, Hutchinson P, Holmes PW, Holdsworth SR. Cytotoxic T lymphocyte and natural killer cell responses to non-typeable Haemophilus influenzae. Clin Exp Immunol. 2008;152(3):542-51. [PubMed ID: 18462210]. https://doi.org/10.1111/j.1365-2249.2008.03667.x.
-
51.
Taylor AE, Finney-Hayward TK, Quint JK, Thomas CM, Tudhope SJ, Wedzicha JA, et al. Defective macrophage phagocytosis of bacteria in COPD. Eur Respir J. 2010;35(5):1039-47. [PubMed ID: 19897561]. https://doi.org/10.1183/09031936.00036709.
-
52.
Ween M, Ahern J, Carroll A, Hodge G, Pizzutto S, Jersmann H, et al. A small volume technique to examine and compare alveolar macrophage phagocytosis of apoptotic cells and non typeable Haemophilus influenzae (NTHi). J Immunol Methods. 2016;429:7-14. [PubMed ID: 26678160]. https://doi.org/10.1016/j.jim.2015.12.004.