Abstract
Background:
Due to the overuse of antibiotics in livestock as a growth-promoting agent, the emergence of multi-antibiotic resistant bacteria is becoming a concern.Objectives:
In this study, we aimed to detect the presence and discover the molecular determinants of foodborne bacteria in retail sausages resistant towards the antibacterial agent amoxicillin-clavulanate.Methods:
Two grams of sausages were chopped into small pieces and transferred into sterile Luria-Bertani (LB) enrichment broths overnight before they were plated on MacConkey agar petri dishes. The bacteria isolated were then screened for amoxicillin-clavulanate resistance, and an antimicrobial susceptibility test of each isolate was performed by using the disc diffusion method. Double synergy and phenotypic tests were carried out to detect the presence of extended spectrum β-lactamase (ESBL). API 20E kit was used to identify the Enterobacteriaceae. All isolates were further examined by polymerase chain reaction (PCR) for resistant genes blaOXA-1, blaOXA-10, plasmid-mediated AmpC (blaCMY and blaDHA), and the chromosome-mediated AmpC, Sul1, blaTEM, and blaSHV genes.Results:
A total of 18 amoxicillin-clavulanate resistant isolates were obtained from seven different types of retail sausages. Only half of them were identified as Enterobacteriaceae, but none were ESBL-producers. All the 18 isolated strains demonstrated resistance towards amoxicillin-clavulanate, penicillin and oxacillin (100%), cefotaxime (71.4%), cefpodoxime (66.7%), and ampicillin (83.3%). blaTEM was the most frequently detected β-lactamase gene. Both plasmid- and chromosomal-bound blaTEM genes were detected in all of the isolated Enterobacteriaceae. blaSHV and Sul1 accounted for 22.2% and 11.1% of the amoxicillin-clavulanate resistant isolates, respectively, whereas blaAMPC, blaCMY, blaDHA, blaOXA-1, and blaOXA-10 were not found in any of the isolates. The only one ESBL-producing bacteria detected in this study was Chryseobacterium meningosepticum, which harbored the blaTEM gene.Conclusions:
The multidrug resistant bacteria that carry antibiotic resistant genes from retail sausages may increase the risk of transmission to humans via the consumption of contaminated sausages. Stricter measures must be taken to address the use of antibiotics in animal agriculture and to consider their potential impact on human health.Keywords
Beta-Lactamase Amoxicillin-Clavulanate Antibiotic Resistance Multidrug Resistance Foodborne Diseases PCR
1. Background
Amoxicillin-clavulanate (also known as augmentin) is a broad-spectrum antibacterial agent used in the treatment of community-acquired respiratory tract infections. An increasing prevalence of amoxicillin-clavulanate resistance has been reported, notably due to the continued spread of β-lactamases-mediated resistance. These enzymes act by cleaving the lactam ring of susceptible antibiotics via an irreversible hydroxylation of the amide bond. β-lactamase enzymes in bacteria are very diverse, and they mutate continuously in response to the heavy pressure of antibiotic use, leading to the development of extended spectrum β-lactamases (ESBLs). Most of the ESBLs are members of the TEM and SHV β-lactamase families, whereas other groups for example CTX-M, OXA, and PER β-lactamase have been discussed more recently (1). ESBLs are transferable, and they contain a number of mutations that allow them to hydrolyze expanded-spectrum β-lactam antibiotics, such as penicillin; first-, second-, and third generation cephalosporins; and aztreonam (2-4).
β-lactamases resistant to inhibition by clavulanic acid were discovered in the early 1990s; these enzymes are mostly variants of TEM-1 or TEM-2 β-lactamase (4). Inhibitor-resistant TEM β-lactamases have been found mainly in clinical isolates of Escherichia coli and in some strains of Klebsiella pneumoniae, K. oxytoca, Proteus mirabilis, and Citrobacter freundii (4). Amoxicillin-clavulanate resistance seems to be mainly associated with hyperproduction of plasmid-mediated TEM enzymes, production of oxacillinases, or due to co-expression of TEM enzymes with OXA-like and/or SHV β-lactamases (5, 6). A study in France showed that up to 41% of amoxicillin-clavulanate resistant Escherichia coli in a hospital were producers of inhibitor-resistant TEM variants (6). Hyperproduction of plasmid and chromosomal-mediated AmpC may also cause amoxicillin-clavulanate resistance. AmpC-type β-lactamases are clinically important cephalosporinases, and their overexpression confers resistance to broad-spectrum cephalosporins, but they are not inhibited by clavulanic acid (7, 8). AmpC enzymes are encoded by both chromosomal (blaAMPC) and plasmid genes (blaDHA and blaCMY) (7). There was also a significant association between the Sul gene and resistance to amoxicillin-clavulanate (9).
The three food categories most frequently associated with antibiotic resistant pathogens and foodborne outbreaks are dairy products, ground beef, and poultry (10, 11). Retail meat products can serve as important sources for the transfer of multidrug resistant bacteria from food-producing animals to the public. Foodborne bacteria often carry high levels of resistance due to the large amounts of antibiotics often given to food-producing animals in feed and water for growth promotion and other non-therapeutic purposes. Studies have shown that feeding low doses of antibiotics to large numbers of food animals over long periods of time leads to resistance (12). Recently, increasing prevalence of antibiotic resistance genes recovered from food sources like poultry has been reported; this is related to the use of antibiotics as growth promoters in livestock (13). Resistance towards amoxicillin-clavulanate in foodborne pathogens has also been reported in China and Nigeria (14, 15). In our previous study, blaCTXM-2, blaSHV, and blaTEM genes were discovered in bacteria isolated from retail sausages in Malaysia (16).
2. Objectives
The present study aimed to identify the gene determinants responsible for amoxicillin-clavulanate resistance in bacteria isolates obtained from retail sausages. Sausages as poultry products could be a source of multidrug-resistant bacteria, which may spread to and cause the development of antibiotic resistance in humans.
3. Methods
3.1. Bacterial Isolation and Identification
Seven different types of retail sausages were collected in Kampar, Perak between January and November, 2014. The selected sausages were chopped into small pieces and weighed to approximately 2 grams before they were transferred into sterile Luria-Bertani (LB) enrichment broths (Laboratories CONDA, Spain). These broths were incubated at 37°C for 16 to 18 hours with agitation. Afterwards, a loop full of bacteria from LB enrichment broth was streaked onto MacConkey agar petri dishes (Oxoid, England) and incubated at 37°C for 24 hours. MacConkey agar was used to selectively grow the Gram negative bacteria and differentiate the lactose fermentation. The purified isolates were preserved and suspended in 80% (v/v) glycerol and stored at -80°C. Further identification of the isolates was carried out using API strips (bioMerieux®, France).
3.2. Antibiotic Susceptibility Test
The isolated bacteria from MacConkey agar was inoculated into 5 ml of Tryptic soy broth (TSB) (Merck, Germany) and incubated at 37°C. After 24 hours, the inoculum with turbidity equivalent to 0.5 McFarland standards were then inoculated evenly on Mueller-Hinton agar (Oxoid, England) using a sterile cotton swab. The antibiotics used were amoxicillin-clavulanate (AMC-30), aztreonam (ATM-30), cefotaxime (CTX-30), gentamicin (CN-10), ampicillin (AMP-10), tetracycline (TE-30) (all from BD BBLTM, United States), cefpodoxime (CPD-10), ceftazidime (CAZ-30), ceftriazone (CRO-30), imipenem (IMP-10), meropenem (MEM-10), norfloxacin (NOR-10), oxacillin (OX-1), penicillin (P-1), and piperacillin (PRL-75) (all from Oxoid, England). The discs were placed on the Mueller-Hinton agar (Oxoid, England) plate. The plates were then inverted and incubated aerobically at 37°C for 16 to 18 hours. In this study, indication of the bacteria as “susceptible,” “intermediate,” or “resistant” towards the antibiotic discs was based on the Zone Diameter Interpretative Standards of the Clinical Laboratory Standard Institute (CLSI) (17).
3.3. Double Disc Synergy Test
This test is a recommended routine practice to detect ESBL production in Gram negative bacilli. The test was performed by using an amoxicillin-clavulanate (AMC-30) (BD BBLTM, United States) disc. The disc was placed at the center of the Mueller-Hinton agar plate, and ceftazidime (CAZ-30) (Oxoid, England) and cefotaxime (CTX-30) (BD BBLTM, United States) were placed 15 mm away from the disc at the center. The plate was incubated overnight at 37°C and then examined. The presence of the keyhole phenomenon was considered a positive result for ESBL production (17).
3.4. Phenotypic Confirmatory Test
A sterile cotton swab was dipped into the organism suspension. The swab was then swabbed over the dry surface of a Mueller-Hinton agar plate to obtain a lawn culture. Discs containing cefotaxime, cefotaxime-clavulanic acid (BD BBLTM, United States), ceftazidime, or ceftazidime-clavulanic acid (Oxoid, England) were arranged so that the distance between them was approximately twice the radius of the inhibition zone produced by the cephalosporin. Based on CLSI guidelines, the isolates were classified as ESBL producers if there was more than (≥) a 5 mm increase in the zone diameter for either ceftazidime or cefotaxime with clavulanic acid versus its zone when tested alone (17).
3.5. Total and Plasmid DNA Extraction
Bacterial isolates were subjected to total DNA extraction using a fast-boiling method (18). Plasmid DNA was extracted using the DNA-spinTM Plasmid DNA Purification Kit (Intron, South Korea).
3.6. Polymerase Chain Reaction (PCR)
PCR was used to screen for the presence of resistant genes, such as blaOXA-1, blaOXA-10, plasmid-mediated AmpC (blaCMY and blaDHA), chromosomally-mediated blaAMPC, Sul1, blaTEM, and blaSHV. In order to detect these resistant genes, gene-specific forward and reverse primers (IDT, Singapore) were used to carry out the PCR reaction (Table 1). After the amplification process, the PCR products were subjected to 1.5% gel electrophoresis.
Nucleotide Sequences and Uses of Oligonucleotide Primers Used in This Study
Primer | Sequence | Expected Size (bp) | Source |
---|---|---|---|
16S rDNA | F: AGA GTT TGA TCC TGG CTC AG | 1492 | (19) |
R: GGT TAC CTT GTT ACG ACT T | |||
blaCMY | F: ATGATGAAAAAATCGTTATGC | 1200 | (20) |
R: TTGCAGCTTTTCAAGAATGCGC | |||
blaDHA | F: TGATGGCACAGCAGGATATTC | 997 | (21) |
R: GCTTTGACTCTTTCGGTATTCG | |||
Chromosome-mediated AmpC | F: TGCTATTTCAAAGGAACCTTCA | 147 | (22) |
R: TTAATGCGCTCTTCATTTGG | |||
blaOXA-1 | F: GTGCGTCAACGGATATCTCT | 736 | (23) |
R: GTGATCGCATTTTTCTTGGC | |||
blaOXA-10 | F: GTCTTTCGAGTACGGCATTA | 720 | (24) |
R: ATTTTCTTAGCGGCAACTTA | |||
Sul1 | F: ATGGTGACGGTGTTCGGCAT | 840 | (25) |
R: CTAGGCATGATCTAACCCTC | |||
blaTEM | F: ATCAGCAATAAACCAGC | 516 | (26) |
R: CCCCGAAGAACGTTTTC | |||
blaSHV | F: TGGTTATGCGTTATATTCCCC | 868 | (16) |
R: GGTTAGCGTTGCCAGTGCT |
4. Results
In this study, a total of 18 strains of bacteria resistant to amoxicillin-clavulanate were successfully isolated from a variety of retail sausages. Half of the isolates (D1, H3, J2, J6, J7, J8, K1, L1, and L2) were identified as members of Enterobacteriaceae by using an API 20E kit, whereas the remaining (C1, E1, E2, E3, H2, J1, J3, J4, J5) isolates were identified as non-Enterobacteriaceae Gram-negative bacteria. The most prominent Enterobacteriaceae isolated from the retail sausages were Klebsiella spp. (33.33%), followed by Enterobacter spp. (49.01%).
The antibiotic susceptibility and interpretation of bacteria isolates isolated from a variety of sausage brands towards the antibiotic agents are summarized in Table 2. Based on the results, all the isolated strains (100%) were resistant towards amoxicillin-clavulanate, penicillin, and oxacillin. Furthermore, the most common resistances were observed against cefotaxime (71.4%), cefpodoxime (66.7%), and ampicillin (83.3%), whereas the least frequent resistances were against piperacillin, imipenem, and meropenem (5.6%) as well as gentamicin (11.1%). Fifty percent of the isolates showed resistance to aztreonam, whereas 33.3% were resistant towards ceftriazone, and 16.7% were resistant to ceftazidime and tetracycline. None of the isolates showed resistance to norfloxacin. The results for the double disc synergy test and phenotypic confirmatory test are summarized in Table 3. In this study, only one (isolate E3) exhibited the keyhole phenomenon on MH agar after incubation and demonstrated a positive phenotypic result. The only ESBL producer in this study was identified to be Chryseobacterium meningosepticum, a non-Enterobacteriaceae bacteria.
Antibiotics susceptibility profile of the amoxicillin-clavulanate (AMC-30) resistant bacterial isolates: aztreonam (ATM-30), cefotaxime (CTX-30), cefpodoxime (CPD-10), ceftriazone (CRO-30), ceftazidime (CAZ-30), ampicillin (AMP-10), penicillin (P-1), oxacillin (OX-1), piperacillin (PRL-75), imipenem (IPM-10), meropenem (MEM-10), norfloxacin (NOR-10), gentamicin (CN-10), and tetracycline (TE-30). R = resistant, I = intermediate resistant, S = susceptible.
Sample in Assigned Code | Diameter of Zone of Inhibition (mm) | ||||||||||||||
---|---|---|---|---|---|---|---|---|---|---|---|---|---|---|---|
AMC-30 | ATM-30 | CTX-30 | CPD-10 | CRO-30 | CAZ-30 | AMP-10 | P-1 | OX-1 | PRL-75 | IPM-10 | MEM-10 | CN-10 | NOR-10 | TE-30 | |
C1 | R (0) | R (23) | R (19) | R (0) | R (21) | S (25) | R (0) | R (0) | R (0) | S (26) | S (34) | S (36) | S (20) | S (26) | R (11) |
D1 | R (9) | R (26) | R (22) | R (0) | R (22) | S (24) | R (0) | R (0) | R (0) | S (21) | S (26) | S (30) | S (16) | S (23) | S (19) |
E1 | R (0) | R (10) | R (21) | R (0) | R (22) | S (25) | R (9) | R (0) | R (0) | S (28) | S (34) | S (42) | S (25) | S (34) | S (20) |
E2 | R (0) | R (0) | R (0) | R (0) | R (0) | R (0) | R (8) | R (0) | R (0) | R (0) | R (10) | R (14) | R (11) | S (19) | R (10) |
E3 | R (0) | R (0) | R (21) | R (14) | S (26) | R (0) | R (0) | R (0) | R (0) | S (29) | S (32) | S (36) | S (30) | S (20) | S (34) |
H2 | R (0) | R (0) | S (28) | R (17) | S (26) | S (25) | R (0) | R (0) | R (0) | S (25) | S (25) | S (31) | S (16) | S (26) | S (16) |
H3 | R (0) | R (0) | S (27) | R (15) | S (25) | S (25) | R (0) | R (0) | R (0) | S (26) | S (25) | S (30) | S (15) | S (25) | S (15) |
J1 | R (7) | S (30) | I (25) | I (18) | I (22) | S (22) | S (18) | R (0) | R (0) | S (28) | S (30) | S (34) | I (14) | S (32) | S (24) |
J2 | R (6) | S (27) | R (22) | S (23) | S (28) | S (28) | I (14) | R (0) | R (0) | S (24) | S (26) | S (33) | S (20) | S (22) | S (18) |
J3 | R (6) | S (23) | R (20) | R (0) | R (17) | S (23) | R (0) | R (0) | R (0) | S (25) | S (24) | S (32) | S (20) | S (28) | R (8) |
J4 | R (0) | S (24) | R (21) | R (0) | I (20) | S (26) | R (0) | R (0) | R (0) | S (26) | S (27) | S (32) | S (21) | S (28) | I (13) |
J5 | R (0) | R (0) | R (0) | R (0) | R (0) | R (0) | S (17) | R (0) | R (0) | S (24) | S (29) | S (30) | S (18) | S (28) | S (26) |
J6 | R (6) | S (30) | S (26) | S (26) | S (26) | S (28) | R (0) | R (0) | R (0) | S (23) | S (28) | S (28) | S (17) | S (28) | S (26) |
J7 | R (13) | S (32) | S (30) | S (27) | S (29) | S (27) | R (0) | R (0) | R (0) | S (23) | S (25) | S (22) | S (17) | S (24) | S (24) |
J8 | R (0) | S (28) | I (25) | R (17) | S (23) | S (24) | R (0) | R (0) | R (0) | S (26) | S (23) | S (29) | R (12) | S (30) | S (20) |
K1 | R (0) | R (0) | R (22) | R (14) | S (32) | S (34) | R (12) | R (0) | R (0) | S (25) | S (25) | S (29) | S (17) | S (30) | S (20) |
L1 | R (13) | S (27) | S (29) | S (30) | S (26) | S (28) | R (7) | R (0) | R (0) | S (22) | S (28) | S (30) | S (16) | S (25) | S (23) |
L2 | R (11) | S (25) | I (24) | I (19) | S (25) | S (24) | R (7) | R (0) | R (0) | S (24) | S (29) | S (30) | S (17) | S (26) | S (24) |
No of resistant isolates | (18/18), 100% | (9/18), 50% | (10/18), 71.4% | (12/18), 66.7% | (6/18), 33.3% | (3/18), 16.7% | (15/18), 83.3% | (18/18), 100% | (18/18), 100% | (1/18), 5.6% | (1/18), 5.6% | (1/18), 5.6% | (2/18), 11.1% | (18/18), 0% | (3/18), 16.7% |
Results for Double Disc Synergy Test, Phenotypic Confirmatory Tests on Bacterial Isolates, and the Taxa of the Significant Bacteria Identified
Sample in Assigned Code | Double Disc Synergy Test | Phenotypic Confirmatory Test | Significant Taxa and % ID |
---|---|---|---|
C1 | Negative | Negative | Pseudomonas aeruginosa (76.4%) |
D1 | Negative | Negative | Enterobacter cancerogenus (99.9%) |
E1 | Negative | Negative | Pseudomonas luteola (98.8%) |
E2 | Negative | Negative | Pasteurella pneumotropica (99.9%) |
E3 | Positive | Positive | Chryseobacterium meningosepticum (87.5%) |
H2 | Negative | Negative | Pantoea spp. 3 (92.2%) |
H3 | Negative | Negative | Klebsiella oxytoca (97.4%) |
J1 | Negative | Negative | Rahnella aquatilis (63.6%) |
J2 | Negative | Negative | Enterobacter cloacae (95.1%) |
J3 | Negative | Negative | Pseudomonas fluorescens / putida (75.5%) |
J4 | Negative | Negative | Pseudomonas fluorescens / putida (75.5%) |
J5 | Negative | Negative | Pseudomonas luteola (95.4%) |
J6 | Negative | Negative | Klebsiella pneumoniae spp. pneumoniae (97.6%) |
J7 | Negative | Negative | Klebsiella pneumoniae spp. pneumoniae (97.6%) |
J8 | Negative | Negative | Enterobacter cloacae (95.1%) |
K1 | Negative | Negative | Enterobacter cloacae (92%) |
L1 | Negative | Negative | Klebsiella pneumoniae spp. pneumoniae (66.4%) |
L2 | Negative | Negative | Klebsiella pneumoniae spp. pneumoniae (97.6%) |
All the profiles of the 18 isolated strains are summarized in Table 4, and the gel electrophoresis for the PCR amplifications are presented in Figure 1 through 3. In this study, none of the bacterial isolates carried any chromosome-mediated blaAMPC, blaCMY, blaDHA, blaOXA-1, or blaOXA-10 genes. Sul1 genes were detected in Pseudomonas aeruginosa (C1) and Enterobacter cloacae (J2), a member of Enterobacteriaceae. In total, a high prevalence of blaTEM was detected in 15 (83.3%) isolates out of 18 isolates that harbored blaTEM in the total DNA or plasmid DNA. For Enterobacteriaceae, all were screened as positive for the blaTEM gene, 14.3% were detected in chromosomal DNA, and the remaining 85.7% were plasmid-encoded. On the other hand, out of the nine non-Enterobacteriaceae, only P. fluorescens/putida (J4) carried chromosomal-encoded blaTEM, whereas the remaining (88.9%) contained plasmid-encoded blaTEM. The only ESBL-producer (E3) screened positive for the presence of plasmid-encoded blaTEM. Additionally, chromosomal-encoded SHV was detected in the four Klebsiella spp. J6, J7, L1, and L2. Although all of the Enterobacteriaceae harbored at least one β-lactamase gene (either blaSHV or blaTEM) in the total DNA or plasmid DNA, none of these was an ESBL-producer.
Summary of Screening Results for Detection of Resistant Genes Within 18 Isolated Bacteria
Bacterial Strain | Total DNA | Plasmid DNA | ||||||||||
---|---|---|---|---|---|---|---|---|---|---|---|---|
blaCMY | blaDHA | blaAMPC | blaOXA-1 | blaOXA-10 | Sul1 | blaTEM | blaSHV | blaCMY | blaDHA | blaTEM | blaSHV | |
C1 (Pseudomonas aeruginosa) | – | – | – | – | – | + | + | – | – | – | + | – |
D1 (Enterobacter cancerogenus) | – | – | – | – | – | – | – | – | – | – | + | – |
E1 (Pseudomonas luteola) | – | – | – | – | – | – | + | – | – | – | + | – |
E2 (Pasteurella pneumotropica) | – | – | – | – | – | – | + | – | – | – | + | – |
E3 (Chryseobacterium meningosepticum) | – | – | – | – | – | – | + | – | – | – | + | – |
H2 (Pantoea spp.) | – | – | – | – | – | – | + | – | – | – | + | – |
H3 (Klebsiella oxytoca) | – | – | – | – | – | – | + | – | – | – | – | – |
J1 (Rahnella aquatilis) | – | – | – | – | – | – | – | – | – | – | – | – |
J2 (Enterobacter cloacae) | – | – | – | – | – | + | + | – | – | – | + | – |
J3 (Pseudomonas fluorescens / putida) | – | – | – | – | – | – | + | – | – | – | + | – |
J4 (Pseudomonas fluorescens / putida) | – | – | – | – | – | – | + | – | – | – | – | – |
J5 (Pseudomonas luteola) | – | – | – | – | – | – | + | – | – | – | + | – |
J6 (Klebsiella pneumoniae) | – | – | – | – | – | – | + | + | – | – | + | – |
J7 (Klebsiella pneumoniae) | – | – | – | – | – | – | – | + | – | – | + | – |
J8 (Enterobacter cloacae) | – | – | – | – | – | – | + | – | – | – | + | – |
K1 (Enterobacter cloacae) | – | – | – | – | – | – | + | – | – | – | + | – |
L1 (Klebsiella pneumoniae) | – | – | – | – | – | – | + | + | – | – | + | – |
L2 (Klebsiella pneumoniae) | – | – | – | – | – | – | + | + | – | – | + | – |
Gel Image of blaSHV Gene Amplification From Total DNA on 1.5% Agarose Gel
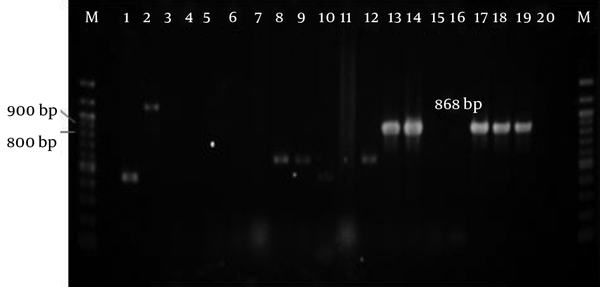
Gel Image of Sul1 Gene Amplification on 1.5% Agarose Gel
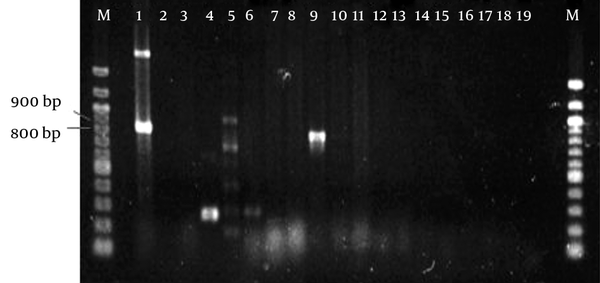
Gel Image of blaTEM Gene Amplification from Plasmid DNA on 1.5% Agarose Gel
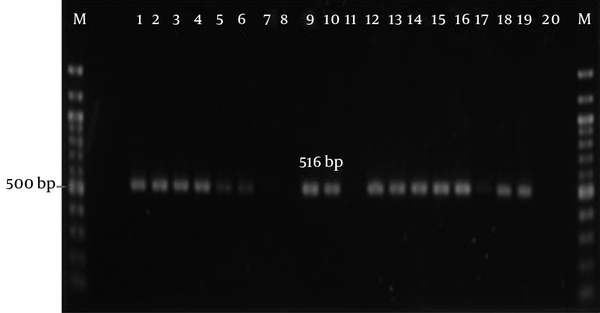
5. Discussion
The present investigation showed multidrug resistance among all the isolates used in this study. In this study, nine (50%) members of Enterobacteriaceae were isolated, but none of these Enterobacteriaceae harbored ESBLs. This is surprising because there have been numerous reports of ESBL-producing Enterobacteriaceae in food sources, especially poultry products (27-29). It is also known that resistant Enterobacteriaceae (i.e., salmonellosis) in food can be transmitted within the community (30, 31). The only one ESBL-producing bacteria detected in this study was Chryseobacterium meningosepticum (E3), a non-Enterobacteriaceae that is known to cause meningitis and pneumonia in patients with compromised immune systems (32, 33). These bacteria are opportunistic human pathogens (33), and they have been isolated from raw and fresh milk (34); however, there have been no reports of their isolation from sausages or poultry products. Here, the isolates exhibited resistance to third generation cephalosporins, including cefotaxime, cefpodoxime, and ceftazidime. This could indicate an increased resistance to β-lactam antibiotics, causing reduction of the efficacy of antibiotic therapy.
As indicated in Table 4, nine of the isolates identified as Enterobacteriaceae did carry either blaSHV or blaTEM genes, but not blaAMPC or blaOXA. blaTEM has been shown to be the most frequently detected amoxicillin-clavulanate resistant Enterobacteriaceae (35). This is further supported by other studies showing that resistance to amoxicillin-clavulanate in E. coli isolates from this area was mainly associated with presumptive overproduction of TEM-1, TEM-2, or SHV-1 β-lactamases or of AmpC cephalosporinase (36-38). However, none of our isolates carried blaAMPC. The carriage of blaSHV was usually found on the chromosomal DNA of K. pneumoniae isolates in our study. This can be supported by studies reporting that genes encoding SHV-1 were naturally found in K. pneumoniae chromosomes (4, 39). Therefore, blaSHV detection in this species is expected and predicted.
Most isolates showed negative results in a phenotypic confirmatory test for ESBL, even though the isolates carried blaTEM and blaSHV genes. This could be due to the hyperproduction of either TEM or SHV β-lactamases in ESBLs, which has been previously reported to cause a false negative phenotypic confirmatory test (40). Moreover, certain ESBL-producers could contain other β-lactamases, including inhibitor-resistant TEMs (IRT), which can mask the production of ESBL in the phenotypic test, leading to a false-negative result (4, 41). Although the inhibitor-resistant TEM variants show clinical resistance to amoxicillin-clavulanate, they remain susceptible to inhibition by tazobactam and subsequently to the combination of piperacillin and tazobactam (4). To confirm whether the isolates are of the ESBL or IRT phenotype, further tests with these antibiotic combinations may be performed in the future.
It is interesting to note that P. aeruginosa (C1) and E. cloacae (J2) co-harbored Sul1 and blaTEM genes in this study. This observation was in agreement with the previous studies, whereby the Sul1 gene was highly prevalent in relation with integron 1 in P. aeruginosa (18, 42). The dissemination of Sul1 may be a result of the horizontal transfer of antibiotic resistance gene cassettes (42). A study in Jordan showed that 53.9% of urinary E. coli isolates were positive for one or more of the Sul genes, and significant resistance was observed towards amoxicillin-clavulanate (9). Interestingly, amoxicillin-clavulanate resistance was also correlated with the presence of Sul in Salmonella enterica isolates in Spain (43). However, all these studies were performed using isolates obtained from humans and not food sources, suggesting that the results obtained in this study are novel.
In conclusion, the fact that amoxicillin-clavulanate resistant isolates were isolated from retail sausages demonstrates the risk of transmission to humans via these sausages if they are not handled or cooked properly prior to consumption. Thus, good practices in hygiene procedures and food preparation are necessary to control and reduce biological risks.
Acknowledgements
References
-
1.
Zurfluh K, Hachler H, Nuesch-Inderbinen M, Stephan R. Characteristics of extended-spectrum beta-lactamase- and carbapenemase-producing Enterobacteriaceae Isolates from rivers and lakes in Switzerland. Appl Environ Microbiol. 2013;79(9):3021-6. [PubMed ID: 23455339]. https://doi.org/10.1128/AEM.00054-13.
-
2.
Fernandes R, Amador P, Oliveira C, Prudencio C. Molecular characterization of ESBL-producing Enterobacteriaceae in northern Portugal. ScientificWorldJournal. 2014;2014:782897. [PubMed ID: 24701189]. https://doi.org/10.1155/2014/782897.
-
3.
Jacoby GA, Medeiros AA. More extended-spectrum beta-lactamases. Antimicrob Agents Chemother. 1991;35(9):1697-704. [PubMed ID: 1952834].
-
4.
Bradford PA. Extended-spectrum beta-lactamases in the 21st century: characterization, epidemiology, and detection of this important resistance threat. Clin Microbiol Rev. 2001;14(4):933-51. [PubMed ID: 11585791]. https://doi.org/10.1128/CMR.14.4.933-951.2001.
-
5.
Nicolas-Chanoine MH. Inhibitor-resistant beta-lactamases. J Antimicrob Chemother. 1997;40(1):1-3. [PubMed ID: 9249197].
-
6.
Leflon-Guibout V, Speldooren V, Heym B, Nicolas-Chanoine M. Epidemiological survey of amoxicillin-clavulanate resistance and corresponding molecular mechanisms in Escherichia coli isolates in France: new genetic features of bla(TEM) genes. Antimicrob Agents Chemother. 2000;44(10):2709-14. [PubMed ID: 10991849].
-
7.
Jacoby GA. AmpC beta-lactamases. Clin Microbiol Rev. 2009;22(1):161-82. [PubMed ID: 19136439]. https://doi.org/10.1128/CMR.00036-08.
-
8.
White AR, Kaye C, Poupard J, Pypstra R, Woodnutt G, Wynne B. Augmentin (amoxicillin/clavulanate) in the treatment of community-acquired respiratory tract infection: a review of the continuing development of an innovative antimicrobial agent. J Antimicrob Chemother. 2004;53 Suppl 1:i3-20. [PubMed ID: 14726431]. https://doi.org/10.1093/jac/dkh050.
-
9.
Nimri L. First Report of Multidrug-Resistant ESBL-Producing Urinary Escherichia coli in Jordan. British Microbiol Res J. 2012;2(2):71-81. https://doi.org/10.9734/bmrj/2012/1360.
-
10.
DeWaal CS, Grooters SV. Antibiotic resistance in foodborne pathogens. Washington, D.C: Center for Science in the Public Interest; 2013.
-
11.
White DG, Zhao S, Simjee S, Wagner DD, McDermott PF. Antimicrobial resistance of foodborne pathogens. Microbes Infect. 2002;4(4):405-12. [PubMed ID: 11932191].
-
12.
Walsh C, Fanning S. Antimicrobial resistance in foodborne pathogens--a cause for concern? Curr Drug Targets. 2008;9(9):808-15. [PubMed ID: 18781926].
-
13.
Stokstad EL, Jukes TH. The multiple nature of the animal protein factor. J Biol Chem. 1949;180(2):647-54. [PubMed ID: 18135798].
-
14.
Adeyanju GT, Ishola O. Salmonella and Escherichia coli contamination of poultry meat from a processing plant and retail markets in Ibadan, Oyo State, Nigeria. Springerplus. 2014;3:139. [PubMed ID: 25674440]. https://doi.org/10.1186/2193-1801-3-139.
-
15.
Wu H, Wang Y, Wu Y, Qiao J, Li H, Zheng S, et al. Emergence of beta-lactamases and extended-spectrum beta-lactamases (ESBLs) producing Salmonella in retail raw chicken in China. Foodborne Pathog Dis. 2015;12(3):228-34. [PubMed ID: 25658910]. https://doi.org/10.1089/fpd.2014.1859.
-
16.
She LY, Tew LS, Chew CH. Occurrence of blaCTXM-2, blaSHV, blaTEM genes in ESBL-producing bacteria from retail sausages in Kampar, Malaysia. Biomedical Res. 2015;26(3):456-60.
-
17.
Performance standards for antimicrobial susceptibility testing; Twenty-third informational supplement M100-S23. Wayne, Pennsylvania, USA: Clinical and Laboratory Standards Institute; 2013.
-
18.
Kor SB, Choo QC, Chew CH. New integron gene arrays from multiresistant clinical isolates of members of the Enterobacteriaceae and Pseudomonas aeruginosa from hospitals in Malaysia. J Med Microbiol. 2013;62(Pt 3):412-20. [PubMed ID: 23180481]. https://doi.org/10.1099/jmm.0.053645-0.
-
19.
Lane DJ. 16S/23S rRNA sequencing. In: Stackebrandt E, Goodfellow M, editors. Nucleic acid techniques in bacterial systematics. United Kingdom: John Wiley and Sons; 1991.
-
20.
Koeck JL, Arlet G, Philippon A, Basmaciogullari S, Thien HV, Buisson Y, et al. A plasmid-mediated CMY-2 beta-lactamase from an Algerian clinical isolate of Salmonella senftenberg. FEMS Microbiol Lett. 1997;152(2):255-60. [PubMed ID: 9231418].
-
21.
Kiiru J, Kariuki S, Goddeeris BM, Butaye P. Analysis of beta-lactamase phenotypes and carriage of selected beta-lactamase genes among Escherichia coli strains obtained from Kenyan patients during an 18-year period. BMC Microbiol. 2012;12:155. [PubMed ID: 22838634]. https://doi.org/10.1186/1471-2180-12-155.
-
22.
Bratu S, Landman D, Martin DA, Georgescu C, Quale J. Correlation of antimicrobial resistance with beta-lactamases, the OmpA-like porin, and efflux pumps in clinical isolates of Acinetobacter baumannii endemic to New York City. Antimicrob Agents Chemother. 2008;52(9):2999-3005. [PubMed ID: 18591275]. https://doi.org/10.1128/AAC.01684-07.
-
23.
Yin Q, Yue D, Peng Y, Liu Y, Xiao L. Occurrence and distribution of antibiotic-resistant bacteria and transfer of resistance genes in Lake Taihu. Microbes Environ. 2013;28(4):479-86. [PubMed ID: 24240317].
-
24.
Aktas Z, Poirel L, Salcioglu M, Ozcan PE, Midilli K, Bal C, et al. PER-1- and OXA-10-like beta-lactamases in ceftazidime-resistant Pseudomonas aeruginosa isolates from intensive care unit patients in Istanbul, Turkey. Clin Microbiol Infect. 2005;11(3):193-8. [PubMed ID: 15715716]. https://doi.org/10.1111/j.1469-0691.2004.01067.x.
-
25.
Galimand M, Courvalin P, Lambert T. Plasmid-mediated high-level resistance to aminoglycosides in Enterobacteriaceae due to 16S rRNA methylation. Antimicrob Agents Chemother. 2003;47(8):2565-71. [PubMed ID: 12878520].
-
26.
Colom K, Perez J, Alonso R, Fernandez-Aranguiz A, Larino E, Cisterna R. Simple and reliable multiplex PCR assay for detection of blaTEM, bla(SHV) and blaOXA-1 genes in Enterobacteriaceae. FEMS Microbiol Lett. 2003;223(2):147-51. [PubMed ID: 12829279].
-
27.
Dolejska M, Matulova M, Kohoutova L, Literak I, Bardon J, Cizek A. Extended-spectrum beta-lactamase-producing Escherichia coli in turkey meat production farms in the Czech Republic: national survey reveals widespread isolates with bla(SHV-12) genes on IncFII plasmids. Lett Appl Microbiol. 2011;53(3):271-7. [PubMed ID: 21671965]. https://doi.org/10.1111/j.1472-765X.2011.03099.x.
-
28.
Pehlivanlar Onen S, Aslantas O, Sebnem Yilmaz E, Kurekci C. Prevalence of beta-Lactamase Producing Escherichia coli from Retail Meat in Turkey. J Food Sci. 2015;80(9):M2023-9. [PubMed ID: 26256548]. https://doi.org/10.1111/1750-3841.12984.
-
29.
Aliyu AB, Saleha AA, Jalila A, Zunita Z. Risk factors and spatial distribution of extended spectrum beta-lactamase-producing- Escherichia coli at retail poultry meat markets in Malaysia: a cross-sectional study. BMC Public Health. 2016;16:699. [PubMed ID: 27484086]. https://doi.org/10.1186/s12889-016-3377-2.
-
30.
Prats G, Mirelis B, Miro E, Navarro F, Llovet T, Johnson JR, et al. Cephalosporin-resistant Escherichia coli among summer camp attendees with salmonellosis. Emerg Infect Dis. 2003;9(10):1273-80. [PubMed ID: 14609463]. https://doi.org/10.3201/eid0910.030179.
-
31.
Chon JW, Jung HI, Kuk M, Kim YJ, Seo KH, Kim SK. High occurrence of extended-spectrum beta-lactamase-producing Salmonella in broiler carcasses from poultry slaughterhouses in South Korea. Foodborne Pathog Dis. 2015;12(3):190-6. [PubMed ID: 25692653]. https://doi.org/10.1089/fpd.2014.1847.
-
32.
Amer MZ, Bandey M, Bukhari A, Nemenqani D. Neonatal meningitis caused by Elizabethkingia meningoseptica in Saudi Arabia. J Infect Dev Ctries. 2011;5(10):745-7. [PubMed ID: 21997946].
-
33.
Hung PP, Lin YH, Lin CF, Liu MF, Shi ZY. Chryseobacterium meningosepticum infection: antibiotic susceptibility and risk factors for mortality. J Microbiol Immunol Infect. 2008;41(2):137-44. [PubMed ID: 18473101].
-
34.
Giannino ML, Marzotto M, Dellaglio F, Feligini M. Study of microbial diversity in raw milk and fresh curd used for Fontina cheese production by culture-independent methods. Int J Food Microbiol. 2009;130(3):188-95. [PubMed ID: 19232767]. https://doi.org/10.1016/j.ijfoodmicro.2009.01.022.
-
35.
Di Conza JA, Badaracco A, Ayala J, Rodriguez C, Famiglietti A, Gutkind GO. beta-lactamases produced by amoxicillin-clavulanate-resistant enterobacteria isolated in Buenos Aires, Argentina: a new blaTEM gene. Rev Argent Microbiol. 2014;46(3):210-7. [PubMed ID: 25444130]. https://doi.org/10.1016/S0325-7541(14)70075-6.
-
36.
Perez-Moreno MO, Perez-Moreno M, Carulla M, Rubio C, Jardi AM, Zaragoza J. Mechanisms of reduced susceptibility to amoxycillin-clavulanic acid in Escherichia coli strains from the health region of Tortosa (Catalonia, Spain). Clin Microbiol Infect. 2004;10(3):234-41. [PubMed ID: 15008945].
-
37.
Rios E, Lopez MC, Rodriguez-Avial I, Pena I, Picazo JJ. Characterization of Inhibitor-Resistant TEM beta-Lactamases and Mechanisms of Fluoroquinolone Resistance in Escherichia coli Isolates. Microb Drug Resist. 2015;21(5):512-5. [PubMed ID: 25945693]. https://doi.org/10.1089/mdr.2015.0039.
-
38.
Sheikh AA, Checkley S, Avery B, Chalmers G, Bohaychuk V, Boerlin P, et al. Antimicrobial resistance and resistance genes in Escherichia coli isolated from retail meat purchased in Alberta, Canada. Foodborne Pathog Dis. 2012;9(7):625-31. [PubMed ID: 22730959]. https://doi.org/10.1089/fpd.2011.1078.
-
39.
Livermore DM. beta-Lactamases in laboratory and clinical resistance. Clin Microbiol Rev. 1995;8(4):557-84. [PubMed ID: 8665470].
-
40.
Rawat D, Nair D. Extended-spectrum beta-lactamases in Gram Negative Bacteria. J Glob Infect Dis. 2010;2(3):263-74. [PubMed ID: 20927289]. https://doi.org/10.4103/0974-777X.68531.
-
41.
Soltan Dallal M, Sabbaghi A, Molla Aghamirzaeie H, Rastegar Lari A, Eshraghian MR, Fallah Mehrabad J, et al. Prevalence of AmpC and SHV β-Lactamases in Clinical Isolates of Escherichia coli From Tehran Hospitals. Jundishapur J Microb. 2013;6(2). https://doi.org/10.5812/jjm.5043.
-
42.
Shin HW, Lim J, Kim S, Kim J, Kwon GC, Koo SH. Characterization of trimethoprim-sulfamethoxazole resistance genes and their relatedness to class 1 integron and insertion sequence common region in gram-negative bacilli. J Microbiol Biotechnol. 2015;25(1):137-42. [PubMed ID: 25348695].