Abstract
Background:
Escherichia coli phytase is an acidic histidine phytase with great specific activity. Pichia pastoris is a powerful system for the heterologous expression of active and soluble proteins which can express recombinant proteins in high cell density fermenter without loss of product yield and efficiently secrete heterologous proteins into the media. Recombinant protein expression is influenced by expression conditions such as temperature, concentration of inducer, and pH. By optimization, the yield of expressed proteins can be increase. Response surface methodology (RSM) has been widely used for the optimization and studying of different parameters in biotechnological processes.Objectives:
In this study, the expression of synthetic appA gene in P. pastoris was greatly improved by adjusting the expression condition.Materials and Methods:
The appA gene with 410 amino acids was synthesized by P. pastoris codon preference and cloned in expression vector pPinkα-HC, under the control of AOX1 promoter, and it was transformed into P. pastoris GS115 by electroporation. Recombinant phytase was expressed in buffered methanol-complex medium (BMMY) and the expression was analyzed by sodium dodecyl sulfate polyacrylamide gel electrophoresis (SDS-PAGE) and enzymatic assay. To achieve the highest level of expression, methanol concentration, pH and temperature were optimized via RSM. Finally, the optimum pH and temperature for recombinant phytase activity was determined.Results:
Escherichia coli phytase was expressed in P. pastoris under different cultivation conditions (post-induction temperature, methanol concentration, and post-induction pH). The optimized conditions by RSM using face centered central composite design were 1% (v/v) methanol, pH = 5.8, and 24.5°C. Under the optimized conditions, appA was successfully expressed in P. pastoris and the maximum phytase activity was 237.2 U/mL after 72 hours of expression.Conclusions:
By optimization of recombinant phytase expression in shake flask culture, we concluded that P. pastoris was a suitable host for high-level expression of phytase and it can possess high potential for industrial applications.Keywords
1. Background
Phytate (myo-inositol hexakisphosphate) is the major storage form of phosphorous in plant seeds (1). It has antinutrient role by complexing with proteins and it can also chelate divalent cations and decrease the bioavailability of phosphorus (2). Animals like poultry and pig are unable to digest phytate phosphorus due to the lack or low level of phytase activity in their intestines; so, inorganic phosphate is used as a feed supplement (3). Phytase (EC 3.1.3.8) is a histidine acid phosphatase capable of hydrolyzing phytate and releasing phosphate groups (4). It is usually used as a feed supplement to effectively improve phosphorous utilization and reduce fecal phosphorous excretion in such animals (1). Different phytase genes from Escherichia coli (1, 5), Bacillus sp. (3, 6), Aspergillus niger (7, 8), and plants (9) have been cloned and expressed. High phytases activity, thermal and pH stability, degradation of different phytate forms and production yields are required for industrial applications (10). Escherichia coli phytase is an acidic histidine phytase which can degrade phytate in acidic pH in the stomach (11). Researchers showed that the phytase gene (appA) of E. coli had the greatest specific activity among the different recombinant expressed phytases (12) and it is more resistant to pepsin activity than fungal phytases (13).
For recombinant proteins production, various hosts such as bacteria, yeast, plant or insect cells are used. Methylotrophic yeast expression system, besides providing high expression level, simplicity of manipulation and stable transformation, can be utilized for increasing the stability of proteins and they have a high growth rate on simple and inexpensive medium (14). Pichia pastoris is a powerful system for the heterologous expression of active and soluble proteins which can express recombinant proteins in high cell density fermenter without loss of product yield and efficiently secrete heterologous proteins to the media (15). AOX1 is a strong promoter induced by methanol in P. pastoris and the recombinant gene under the control of the AOX1 promoter is highly expressed in the presence of methanol as the sole carbon source and is repressed by most other carbon sources (16).
Recombinant protein expression is influenced by multiple parameters including the expression host strain and expression conditions such as temperature, inducer concentration, pH, induction time, and composition of the culture medium. By optimization of these parameters, the yield of expressed proteins can be increase. For optimization of protein expression, conventional one-factor-at-a-time method can be used (17). In this method one factor changes, while all other factors are kept fixed. This method is time consuming and the interactions between the parameters are ignored (18). Response surface methodology (RSM) is a statistical software for designing experiments used for optimization of conditions with a limited number of experiments (19). Response surface methodology has been widely used for studying the interactions of several parameters during bioprocess optimization in different biotechnological processes (20).
2. Objectives
The appA gene is 1233 bp long comprising one open reading frame, encoding a polypeptide of 410 amino acid residues which has been successfully expressed in E. coli (21) and P. pastoris (1, 5). In this study, the appA gene was designed and synthesized by P. pastoris codon preference. The synthetic phytase gene was cloned and expressed in P. pastoris successfully. We demonstrated improvement of recombinant phytase expression in P. pastoris by optimization of the expression conditions. The biochemical properties of the recombinant phytase were also determined.
3. Materials and Methods
3.1. Strains, Plasmids, Culture Conditions and DNA Techniques
Escherichia coli DH5α was used for cloning, transformation, and propagation. The host, P. pastoris strain GS115 and pPinkα-HC plasmid (7.9 kb) were obtained from Invitrogen (USA). Escherichia coli was grown in Luria and Bertani (LB, SIGMA, Germany) medium at appropriate conditions (37°C with 200 rpm shaking). P. pastoris was cultured in YPD , SIGMA, Germany (1% yeast extract (YE), 2% peptone, 2% glucose/dextrose) and buffered glycerol-complex (BMGY) (1% glycerol, 1% YE, 2% peptone, 1.34% yeast nitrogen base w/o amino acids and ammonium sulfate (YNB), 0.0004% biotin in pH = 6.0, and 100 mM potassium phosphate buffer) media and induced in buffered methanol-complex medium (BMMY) (as per BMGY except with 0.5 or 3% v/v methanol instead of the glycerol) from Invitrogen (USA). The growth conditions for P. pastoris was 28 - 30°C and 300 rpm shaking. Unless otherwise stated, standard DNA methodologies were used.
3.2. Design and Synthesis of the Phytase Gene
The codon preferences of E. coli and P. pastoris are significantly different. A gene encoding phosphoanhydride phosphohydrolase (appA) from E. coli (strain K12) was designed according to its protein sequence published in GenBank (accession No. P07102). To improve the phytase expression in P. pastoris, codon optimization was done to the codon bias of P. pastoris genes using NCBI-related database at (http://www.kazusa.or.jp/codon), according to 30 native genes known to be highly expressed in P. pastoris (22). Furthermore, negative secondary mRNA structures, AT-rich and GC-rich regions, and cryptic splice sites were removed and the GC content was adjusted to 44%. The designed sequence was synthesized by Shinegene (China) (GenBank accession No. KP159621) and cloned into pUC57 plasmid named pUC-Phyt.
3.3. Construction of the Phytase Expression Vector and Transformation
The synthetic appA gene was cloned into the XhoI/KpnI cleavage sites of an expression vector pPinkα-HC, under the control of a methanol-inducible (AOX1) promoter and directly downstream of an α-factor secretion signal sequence. Recombinant pPinkα-appA plasmid was linearized by digestion with BspT1 restriction enzyme and it was transformed into P. pastoris GS115 by electroporation (BTX, ECM630 at 1800 V, 200 Ω and 25 µF with 0.2 cm cuvette), following the Invitrogen protocol. After the transformation, the cuvette contents were spread on PAD plates (Pichia Adenine Dropout) and incubated at 30°C for 10 days. As a negative control, the vector of pPinkα-HC was transformed into P. pastoris.
3.4. Polymerase Chain Reaction Analysis of Pichia Integrants
Selected colonies of transgenic yeast were checked by colony polymerase chain reaction (PCR) to directly test P. pastoris clones for insertion of the appA gene. The cells were lysed by five rounds of heat treatment in a microwave oven, followed by overnight freezing. After centrifuging, the genomic DNA was used as a template for PCR, using specific primers for the appA gene (appA forward: 5ˊ-AGATTGGTTGCTGACGGTTTG-3ˊ and appA reverse: 5ˊ-GCAACAAATGAGAGACAAGACTCC-3ˊ). The standard cycling conditions used for PCRs were as follows: five minutes at 95ºC, 30 cycles of 35 seconds at 95ºC, 40 seconds at 60ºC, one minute at 72°C, and one cycle for five minutes at 72°C.
3.5. Phytase Expression in Pichia pastoris
Five milliliters of BMGY medium was inoculated with one colony of the positive transformants and incubated at 30°C for 48 hours with 300 rpm shaking until an OD600 of 2 - 5 was reached. After that, the cells were collected by centrifugation and induced by methanol in BMMY induction medium at 30°C. Methanol was added to the flasks every 24 hours to keep the final methanol concentration at 1%. The culture supernatants were harvested by centrifugation and the expressed recombinant phytase were analyzed by 12% sodium dodecyl sulfate polyacrylamide gel electrophoresis (SDS-PAGE). The amount of the total protein was determined by the Bradford method, using bovine serum albumin (BSA) as a standard (23).
3.6. Experimental Design and Optimization of Phytase Expression Condition by Response Surface Methodology
To enhance the expression level of recombinant phytase, a design with more than two levels should be carried out (24). Therefore, an RSM based on the central composite design (CCD) was used to achieve optimized levels of methanol concentration, pH and temperature. Minitab 16 software (Minitab Inc., USA) was taken to design the experiment. Each factor was tested at three levels: +1 (the higher value of the factors), 0 (central point) and -1 (the lower value of the factors) (Table 1). The design consisted of a central composite design with six replications of the central points (all factor levels at 0). The phytase activity of the secreted recombinant protein was measured in different conditions. The experimental design matrix is given in Table 2. Three replicated experiments were performed for every experiment. Non-recombinant P. pastoris host cells and Pichia transformed with native vector (without insert) were used as negative controls.
Experimental Range of the Three Variables Studied Using Central Composite Design in Terms of Actual and Coded Factors
Variable Levels | Component | Level | ||
---|---|---|---|---|
-1 | 0 | +1 | ||
A | pH | 4 | 6 | 8 |
B | Methanol concentration, % | 1 | 2 | 3 |
C | Temperature. °C | 20 | 25 | 30 |
Central Composite Design of Factors in Coded Levels With Phytase Activity as Response
Run | Factor A, pH | Factor B, Methanol, % | Factor C, Temperature, °C | Phytase Activity, U/mL | |
---|---|---|---|---|---|
Experimental | Predicted | ||||
1 | -1 | -1 | -1 | 127.7 | 132.553 |
2 | 1 | -1 | -1 | 113.1 | 116.923 |
3 | -1 | 1 | -1 | 65.3 | 60.013 |
4 | 1 | 1 | -1 | 57.9 | 50.833 |
5 | -1 | -1 | 1 | 98.3 | 102.293 |
6 | 1 | -1 | 1 | 87.1 | 89.313 |
7 | -1 | 1 | 1 | 50.2 | 43.303 |
8 | 1 | 1 | 1 | 44.7 | 36.773 |
9 | -1 | 0 | 0 | 146.4 | 149.736 |
10 | 1 | 0 | 0 | 129.7 | 138.656 |
11 | 0 | -1 | 0 | 235.1 | 220.216 |
12 | 0 | 1 | 0 | 130.5 | 157.676 |
13 | 0 | 0 | -1 | 117.3 | 120.976 |
14 | 0 | 0 | 1 | 90.2 | 98.816 |
5 | 0 | 0 | 0 | 195.5 | 182.019 |
16 | 0 | 0 | 0 | 191.4 | 182.019 |
17 | 0 | 0 | 0 | 184.9 | 182.019 |
18 | 0 | 0 | 0 | 170.6 | 182.019 |
19 | 0 | 0 | 0 | 193.1 | 182.019 |
20 | 0 | 0 | 0 | 181.2 | 182.019 |
3.7. Enzymatic Assay
The phytase assay was performed according to the method of Bae (25). Briefly, 75 μL of enzyme solution was added to 300 μL substrate solution (1.5 mM sodium phytate in 0.1 M sodium acetate buffer, pH = 5.0) and incubated at 37°C for 20 minutes. The reaction was stopped by adding 375 μL of 5% (w/v) trichloroacetic acid. The liberated inorganic phosphate was analyzed by adding 375 μL of a coloring reagent (mixing four volumes of 1.5% (w/v) ammonium molybdate in a 5.5% (v/v) sulfuric acid solution and one volume of a 2.7% (w/v) ferrous sulfate solution) and the solution absorbance was determined at 700 nm. One unit (U) of enzyme activity was the amount of the enzyme releasing 1 mmol of either p-nitrophenol or inorganic phosphate per minute at 37°C.
3.8. Genetic Stability of Phytase Expression
To investigate the stable inheritance and stable expression levels of the appA genes in progenies, five clones of the transgenic Pichia strain were grown on YPD for 10 cycles (after the culture reached the stationary phase on YPD, 10 mL of the final culture was inoculated into new 100 mL YPD for the next cycle of growth. This was similarly carried out for 10 cycles). Phytase enzyme activities and appA gene expression levels were determined in the strain after 10 generations of culture.
3.9. Characterization of Recombinant Enzyme
The optimum pH and temperature for recombinant enzyme activity was determined using the standard assay at pH range of 1 - 10 and temperature ranging 30 - 80°C, respectively. For phytase optimum pH determination, different buffers were used glycine-HCl (pH 1 - 3.5), sodium acetate-acetic acid (pH 3.5 - 6), Tris-acetic acid (pH 6-7), Tris-HCl (pH 7 - 9), and glycine-NaOH (pH 9 - 10). All the buffers were used at 100 mM for phytase activity.
4. Results
4.1. Codon Optimization
The appA gene from E. coli is 1233 bp long and encodes a polypeptide of 410 amino acid residues. The coding sequence of appA was synthesized with codon optimization of P. pastoris with codon adaptation index (CAI) value of 0.92. During the optimization process, GC-rich sequence, RNA instability motifs and other negative cis-acting sequences were avoided. Comparison between the optimized sequence (GenBank KP159621) and the wild type (GenBank JF274478) showed 71.1% identity (Figure 1). With optimization of the appA gene, 356 nucleotides were changed, which led to the optimization of 295 amino acid codons (71.8%) and a reduction in the G + C content from 54.6% to 44% (Figure 1). According to the difference between the codon frequencies in the Kazusa database and native high expressed genes for Pichia, it seems that the codon frequencies in highly expressed genes is the more reliable choice for the optimization of genes for high level expression.
Comparison of Nucleotide Sequences Between the Optimized appA Gene (Op-appA) (GenBank Accession No. KP159621) and the Wild Type appA Gene (Wd-appA) (GenBank Accession No. JF274478)
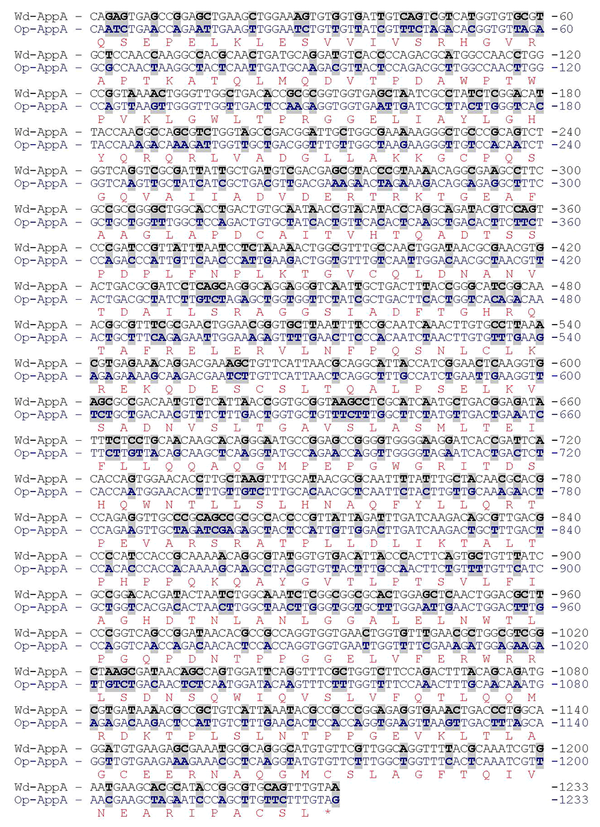
4.2. Cloning and Expression of the appA Gene in Pichia pastoris
For P. pastoris transformation, the synthetic appA gene was ligated into pPinkα-HC between XhoI and KpnI sites, resulting in a construct of pPinkα-appA transformed into E. coli DH5α. Several positive clones were checked by colony PCR with appA forward and appA reverse primers to confirm the presence of the appA gene. Nucleotide sequencing of pPinkα-appA confirmed that the appA gene was inserted in frame into the plasmid. For efficient integration into the Pichia genome at the TRP2 region, pPinkα-appA was lineared by BspT1 restriction enzyme before the transformation.
After the transformation of Pichia, screening of the transformants was carried out on Pichia adenine dropout (PAD) agar plates. Subsequently, to determine the presence of the appA gene in transformants, the extracted DNA from several colonies was used as template for PCR screening with appA-specific primers. PCR amplification results for P. pastoris transformants showed that only one band appeared at 900 bp using the genomic DNA from positive recombinant colonies as the template (Figure 2A).
PCR and SDS-PAGE Analysis of Recombinant Phytase
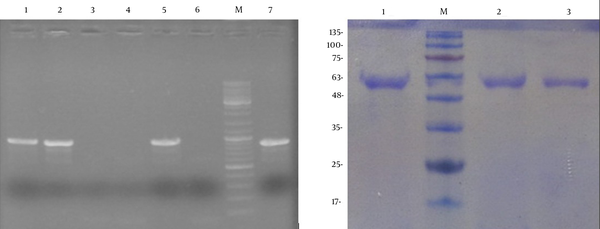
Recombinant phytase in the culture medium was expressed under the control of the AOX1 promoter, after induction by 1% methanol in shaking flasks in BMGY medium for 48 hours. Recombinant phytase (AppA) has 410 amino acids and its molecular weight is about 45 kDa without glycosylation. As we know, when expressing in P. pastoris, the recombinant proteins are usually glycosylated. Accordingly, SDS-PAGE analysis of the recombinant phytase expressed in P. pastoris demonstrated a sharp band with larger molecular mass (about 59 kDa) after glycosylation (Figure 2B). These results indicated that the recombinant phytase was a glycosylated protein and it was successfully expressed in P. pastoris.
4.3. Genetic Stability of Phytase Expression
The PCR experiment on 65 colonies confirmed that 58 of the transformants contained integrated appA sequence in their genomic DNA. One of the advantages of protein expression in yeast is the ability of stable transformation in them. The stability of gene integration in recombinant P. pastoris was confirmed by PCR verification of the appA gene. After 10 successive generations of culture, the strain that expressed the recombinant phytase maintained 97% of the original phytase activity (data not shown). These results demonstrated that the re-engineered strain with the appA gene was stably inherited.
4.4. Experimental Design and Statistical Analysis for Optimization of appA Expression by Pichia pastoris in Shaking Flask
Recombinant P. pastoris strain containing the appA gene was inoculated in BMGY medium, until an OD600 value of 2 - 5 was reached. BMMY medium was used as the optimal medium for the expression of recombinant phytase. For continuous expression, every 24 hours, methanol was added to the flasks and the phytase activity in supernatants was measured after 72 hours. P. pastoris transformed by pPinkα-HC plasmid as a negative control had no endoglucanase expression.
The use of statistically based experimental design is a vital tool in optimization of expression conditions which may increase phytase production. RSM has many advantages such as the factor effects and determination of the optimum values with interaction of different factors in less experimental requirements (20, 26). The levels of factors (pH, methanol concentration and temperature) and the effect of their interactions on phytase activity were determined by central composite design of RSM. Twenty experiments were carried out at different combinations of the factors, which are shown in Table 2. The predicted and experimental responses are shown in Table 2 the results were analyzed. The second-order regression equation provided the levels of phytase activity as the function of pH, methanol concentration and temperature, which can be presented in terms of coded factors as in the following equation:

Where the response (Y) is the phytase activity, while A, B and C are pH, methanol concentration and temperature, respectively. This equation can be used to predict the phytase activity within experiments. Figure 3 shows the agreement of actual and predicted response values.
Predicted Response Versus Actual Value
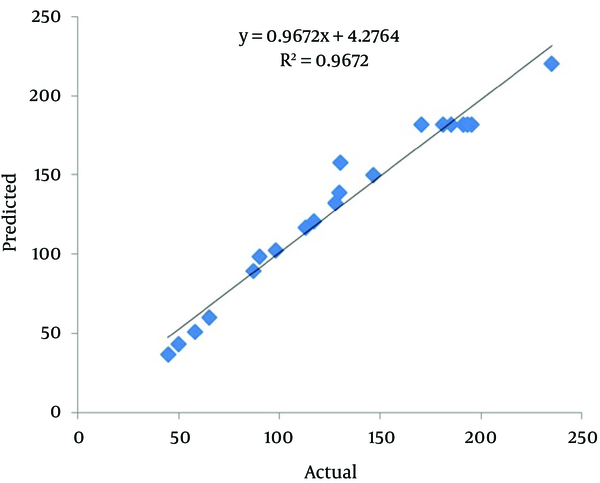
The response surface plots and their corresponding contour plots described by the second-order polynomial equation are illustrated in Figure 4. It demonstrates that the peak of the surface in the model contained the entire optimum region for phytase activity (24). With the prediction of the maximum phytase activity, an optimal design was carried out with the aid of Minitab 16. A maximum phytase activity (221.28 U/mL) was predicted at the following condition: pH = 5.8, 1% of methanol concentration and 24.5°C. In our experiments, appA was successfully expressed at optimum conditions using the P. pastoris yeast for 72 hours. The maximum phytase activity was 237.2 U/mL (mean result of three repetitions), suggesting that experimental and predicted values were in good agreement, validating the model.
Response Surface Plot and Its Contour Plot Described by the Y Model
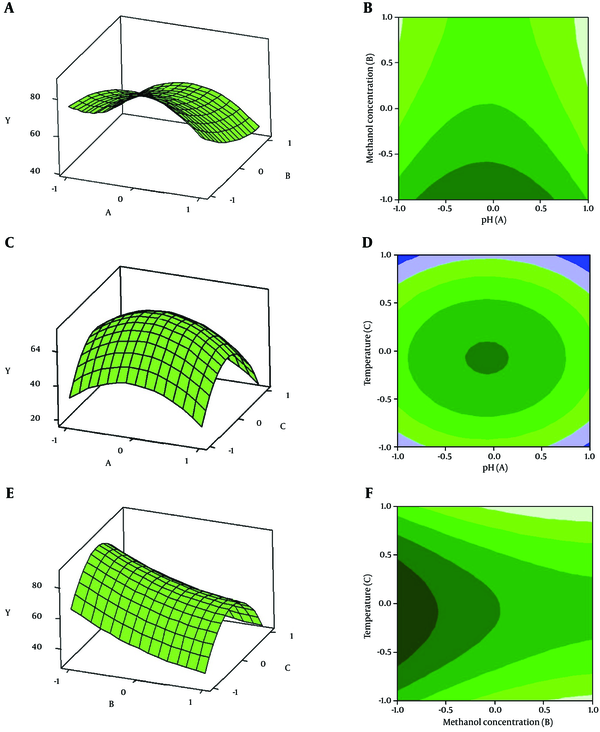
4.5. Effect of Different pH and Temperature Values on Phytase Activity
We studied the pH effects on enzymatic activity of AppA protein (Figure 5A). It exhibited a single pH optimum of 4.5 for phytase activity while it kept more than 80% of the maximal activity in the pH range of 3.5 - 5. We then measured the activity of the recombinant enzyme in different temperatures (Figure 5B). It exhibited maximum phytase activity at 60°C and the recombinant enzyme kept 70% of its activity in the range of 45% - 70°C (Figure 5B).
Characterization of the Recombinant Phytase From Pichia pastoris
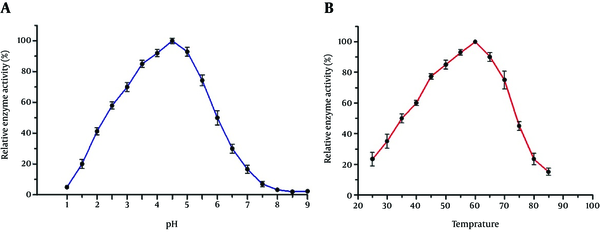
5. Discussion
Recently, P. pastoris has become a commercial expression system for recombinant expression of different proteins. P. pastoris has a lot of advantages as an expression host including a strongly inducible promoter, stable transformation and high cell density fermentation (8). For increasing the recombinant protein expression in P. pastoris, codon optimization technique has been widely used (27, 28). Moreover, Sinclair and Choy demonstrated that rare codons decrease mRNA stability and its translation rate, while high G+C contents could cause reduced translational yields or even failed expression (29). We optimized the appA gene with P. pastoris codon preference and reduced the G+C content from 54.6% to 44%, closer to the average G + C content of other highly expressed genes in P. pastoris (30). Bae et al. previously expressed native appA gene in P. pastoris without codon optimization (25). The lower expression level (100 U/mL) compared to our results (237.2 U/mL) might be because of codon preference differences between E. coli and P. pastoris. A previous study showed that codon optimization can increase the recombinant protein expression by 2 - 3 folds (22).
Here we tried to reach the maximum phytase activity by P. pastoris expression system; so, a scientific and effective method was required to improve the yield of recombinant phytase production. Previous researches demonstrated that the culture condition and inducer concentration were effective factors in recombinant protein expression (31, 32). Therefore, three different factors including the expression pH, expression temperature and methanol concentration were selected to investigate here and the corresponding levels were determined according to our precious work in laboratory (27).
Single factor optimization studies on expression condition are highly time-consuming and we cannot investigate the interaction between different variables in an effective way. Furthermore, with RSM, we can verify the interactions between the variables (33). Therefore, RSM was used to obtain the optimal conditions for desirable responses and also reduce the number of experiments. CCD was thus carried out to improve the phytase expression yield. CCD predicted that a maximum recombinant phytase activity was obtained at the condition of pH = 5.8, 1% of methanol concentration (commonly used conditions for protein expression in P. pastoris (16)), and 24.5°C expression temperature. For protein expression in P. pastoris, 30°C is usual (16). However, Hong et al. (34) demonstrated that the amount of recombinant protein dramatically increased at lower temperature because of the increased stability of the recombinant protein. Our experiment results showed that the maximum recombinant phytase activity was at 24.5°C. It seems that lower temperature could cause higher enzyme activity by increasing protein concentration and decreasing protein misfolding.
Biochemical properties investigation of recombinant phytase showed that the optimal pH and temperature for recombinant appA were 4.5 and 60°C, respectively, the same as native appA (21, 35). It demonstrated that recombinant phytase expressed in yeast maintained its activity and biochemical properties. Following the critical variables selection, the expression optimization by RSM allowed reaching a maximum phytase activity in the crude intracellular medium (237.2 U/mL) by optimization of the coding sequence and expression conditions in shake flask. Our findings showed that P. pastoris was an excellent host for phytase expression, with a substantial value for industrial applications. Our results can be used for industrial expression of phytase in high cell density fermentation.
Acknowledgements
References
-
1.
Chen CC, Wu PH, Huang CT, Cheng KJ. A Pichia pastoris fermentation strategy for enhancing the heterologous expression of an Escherichia coli phytase. Enzyme Microb Technol. 2004;35(4):315-20. https://doi.org/10.1016/j.enzmictec.2004.05.007.
-
2.
Xiong AS, Yao QH, Peng RH, Han PL, Cheng ZM, Li Y. High level expression of a recombinant acid phytase gene in Pichia pastoris. J Appl Microbiol. 2005;98(2):418-28. [PubMed ID: 15659196]. https://doi.org/10.1111/j.1365-2672.2004.02476.x.
-
3.
Rao DE, Rao KV, Reddy VD. Cloning and expression of Bacillus phytase gene (phy) in Escherichia coli and recovery of active enzyme from the inclusion bodies. J Appl Microbiol. 2008;105(4):1128-37. [PubMed ID: 18479345]. https://doi.org/10.1111/j.1365-2672.2008.03833.x.
-
4.
Zinin NV, Serkina AV, Gelfand MS, Shevelev AB, Sineoky SP. Gene cloning, expression and characterization of novel phytase from Obesumbacterium proteus. FEMS Microbiol Lett. 2004;236(2):283-90. [PubMed ID: 15251209]. https://doi.org/10.1016/j.femsle.2004.05.051.
-
5.
Stahl CH, Wilson DB, Lei XG. Comparison of extracellular Escherichia coli AppA phytases expressed in Streptomyces lividans and Pichia pastoris. Biotechnol Lett. 2003;25(10):827-31. [PubMed ID: 12882015].
-
6.
Guerrero-Olazaran M, Rodriguez-Blanco L, Carreon-Trevino JG, Gallegos-Lopez JA, Viader-Salvado JM. Expression of a Bacillus phytase C gene in Pichia pastoris and properties of the recombinant enzyme. Appl Environ Microbiol. 2010;76(16):5601-8. [PubMed ID: 20601512]. https://doi.org/10.1128/AEM.00762-10.
-
7.
Ushasree MV, Vidya J, Pandey A. Gene cloning and soluble expression of Aspergillus niger phytase in E. coli cytosol via chaperone co-expression. Biotechnol Lett. 2014;36(1):85-91. [PubMed ID: 24078121]. https://doi.org/10.1007/s10529-013-1322-3.
-
8.
Xuan NT, Hang MT, Thanh VN. Cloning and over Expression of an Aspergillus niger XP Phytase Gene (phyA) in Pichia pastoris. World Acad Sci Engin Technol. 2009;56:750-3.
-
9.
Johnson SC, Yang M, Murthy PP. Heterologous expression and functional characterization of a plant alkaline phytase in Pichia pastoris. Protein Expr Purif. 2010;74(2):196-203. [PubMed ID: 20655385]. https://doi.org/10.1016/j.pep.2010.07.003.
-
10.
Tai HM, Yin LJ, Chen WC, Jiang ST. Overexpression of Escherichia coli phytase in Pichia pastoris and its biochemical properties. J Agric Food Chem. 2013;61(25):6007-15. [PubMed ID: 23738921]. https://doi.org/10.1021/jf401853b.
-
11.
Lassen SF, Breinholt J, Ostergaard PR, Brugger R, Bischoff A, Wyss M, et al. Expression, gene cloning, and characterization of five novel phytases from four basidiomycete fungi: Peniophora lycii, Agrocybe pediades, a Ceriporia sp., and Trametes pubescens. Appl Environ Microbiol. 2001;67(10):4701-7. [PubMed ID: 11571175].
-
12.
Wyss M, Brugger R, Kronenberger A, Remy R, Fimbel R, Oesterhelt G, et al. Biochemical characterization of fungal phytases (myo-inositol hexakisphosphate phosphohydrolases): catalytic properties. Appl Environ Microbiol. 1999;65(2):367-73. [PubMed ID: 9925555].
-
13.
Rodriguez E, Wood ZA, Karplus PA, Lei XG. Site-directed mutagenesis improves catalytic efficiency and thermostability of Escherichia coli pH 2.5 acid phosphatase/phytase expressed in Pichia pastoris. Arch Biochem Biophys. 2000;382(1):105-12. [PubMed ID: 11051103]. https://doi.org/10.1006/abbi.2000.2021.
-
14.
Akbarzadeh A, Ranaei Siadat SO, Zamani MR, Motallebi M, Barshan Tashnizi M. Comparison of biochemical properties of recombinant endoglucanase II of Trichoderma reesei in methylotrophic yeasts, Pichia pastoris and Hansenula polymorpha. Progress Biol Sci. 2013;3(1):108-17.
-
15.
Gellissen G, Kunze G, Gaillardin C, Cregg JM, Berardi E, Veenhuis M, et al. New yeast expression platforms based on methylotrophic Hansenula polymorpha and Pichia pastoris and on dimorphic Arxula adeninivorans and Yarrowia lipolytica - a comparison. FEMS Yeast Res. 2005;5(11):1079-96. [PubMed ID: 16144775]. https://doi.org/10.1016/j.femsyr.2005.06.004.
-
16.
Cregg JM, Cereghino JL, Shi J, Higgins DR. Recombinant protein expression in Pichia pastoris. Mol Biotechnol. 2000;16(1):23-52.
-
17.
Francis DM, Page R. Strategies to optimize protein expression in E. coli. Curr Protoc Protein Sci. 2010;Chapter 5:Unit 5 24 1-29. [PubMed ID: 20814932]. https://doi.org/10.1002/0471140864.ps0524s61.
-
18.
Chambers SP, Swalley SE. Designing experiments for high-throughput protein expression. Methods Mol Biol. 2009;498:19-29. [PubMed ID: 18988016]. https://doi.org/10.1007/978-1-59745-196-3_2.
-
19.
Maharjan S, Singh B, Bok JD, Kim JI, Jiang T, Cho CS, et al. Exploring codon optimization and response surface methodology to express biologically active transmembrane RANKL in E. coli. PLoS One. 2014;9(5). eee96259. [PubMed ID: 24809485]. https://doi.org/10.1371/journal.pone.0096259.
-
20.
Muntari B, Amid A, Mel M, Jami MS, Salleh HM. Recombinant bromelain production in Escherichia coli: process optimization in shake flask culture by response surface methodology. AMB Express. 2012;2:12. [PubMed ID: 22336426]. https://doi.org/10.1186/2191-0855-2-12.
-
21.
Golovan S, Wang G, Zhang J, Forsberg CW. Characterization and overproduction of the Escherichia coli appA encoded bifunctional enzyme that exhibits both phytase and acid phosphatase activities. Can J Microbiol. 2000;46(1):59-71. [PubMed ID: 10696472].
-
22.
Bai J, Swartz DJ, Protasevich ,I, Brouillette CG, Harrell PM, Hildebrandt E, et al. A gene optimization strategy that enhances production of fully functional P-glycoprotein in Pichia pastoris. PLoS One. 2011;6(8). eee22577. [PubMed ID: 21826197]. https://doi.org/10.1371/journal.pone.0022577.
-
23.
Bradford MM. A rapid and sensitive method for the quantitation of microgram quantities of protein utilizing the principle of protein-dye binding. Anal Biochem. 1976;72:248-54. [PubMed ID: 942051].
-
24.
Li X, Xu T, Ma X, Guo K, Kai L, Zhao Y, et al. Optimization of culture conditions for production of cis-epoxysuccinic acid hydrolase using response surface methodology. Bioresour Technol. 2008;99(13):5391-6. [PubMed ID: 18083551]. https://doi.org/10.1016/j.biortech.2007.11.017.
-
25.
Bae HD, Yanke LJ, Cheng KJ, Selinger LB. A novel staining method for detecting phytase activity. J Microbiol Methods. 1999;39(1):17-22. [PubMed ID: 10579503].
-
26.
Salihu A, Alam MZ, AbdulKarim MI, Salleh HM. Optimization of lipase production by Candida cylindracea in palm oil mill effluent based medium using statistical experimental design. J Mol Catalysis Enzym. 2011;69(1):66-73.
-
27.
Akbarzadeh A, Ranaei Siadat SO, Motallebi M, Zamani MR, Barshan Tashnizi M, Moshtaghi S. Characterization and high level expression of acidic endoglucanase in Pichia pastoris. Appl Biochem Biotechnol. 2014;172(4):2253-65. [PubMed ID: 24347161]. https://doi.org/10.1007/s12010-013-0672-6.
-
28.
Wang H, Wang Q, Zhang F, Huang Y, Ji Y, Hou Y. Protein expression and purification of human Zbtb7A in Pichia pastoris via gene codon optimization and synthesis. Protein Expr Purif. 2008;60(2):97-102. [PubMed ID: 18482847]. https://doi.org/10.1016/j.pep.2008.03.017.
-
29.
Sinclair G, Choy FY. Synonymous codon usage bias and the expression of human glucocerebrosidase in the methylotrophic yeast, Pichia pastoris. Protein Expr Purif. 2002;26(1):96-105. [PubMed ID: 12356476].
-
30.
Huang H, Yang P, Luo H, Tang H, Shao N, Yuan T, et al. High-level expression of a truncated 1,3-1,4-beta-D-glucanase from Fibrobacter succinogenes in Pichia pastoris by optimization of codons and fermentation. Appl Microbiol Biotechnol. 2008;78(1):95-103. [PubMed ID: 18080120]. https://doi.org/10.1007/s00253-007-1290-4.
-
31.
Batra J, Beri D, Mishra S. Response surface methodology based optimization of beta-glucosidase production from Pichia pastoris. Appl Biochem Biotechnol. 2014;172(1):380-93. [PubMed ID: 24081708]. https://doi.org/10.1007/s12010-013-0519-1.
-
32.
Yu Y, Zhou X, Wu S, Wei T, Yu L. High-yield production of the human lysozyme by Pichia pastoris SMD1168 using response surface methodology and high-cell-density fermentation. Electron J Biotechnol. 2014;17(6):311-6. https://doi.org/10.1016/j.ejbt.2014.09.006.
-
33.
Cao W, Li H, Zhang J, Li D, Acheampong DO, Chen Z, et al. Periplasmic expression optimization of VEGFR2 D3 adopting response surface methodology: antiangiogenic activity study. Protein Expr Purif. 2013;90(2):55-66. [PubMed ID: 23680780]. https://doi.org/10.1016/j.pep.2013.04.010.
-
34.
Hong F, Meinander NQ, Jonsson LJ. Fermentation strategies for improved heterologous expression of laccase in Pichia pastoris. Biotechnol Bioeng. 2002;79(4):438-49. [PubMed ID: 12115407]. https://doi.org/10.1002/bit.10297.
-
35.
Greiner R, Konietzny U, Jany KD. Purification and characterization of two phytases from Escherichia coli. Arch Biochem Biophys. 1993;303(1):107-13. [PubMed ID: 8387749]. https://doi.org/10.1006/abbi.1993.1261.