Abstract
Background:
Wound infections caused by methicillin-resistant Staphylococcus aureus are a health problem worldwide; therefore, it is necessary to develop new antimicrobial compounds. Considering broad-spectrum antimicrobial activity and low probability of drug resistance to peptides, applications these peptides are being studied extensively.Objectives:
In this study, to control drug release over time, an alginate sulfate-based hydrogel impregnated with the CM11 peptide as the antimicrobial agent was developed, and its healing effects were tested on skin infections caused by methicillin-resistant S. aureus strains in a mouse model.Materials and Methods:
Minimum inhibitory and minimum bactericidal concentrations of the CM11 peptide and alginate hydrogel in combination with the peptide were determined. Forty mice were divided into 4 groups: 1 group as a negative control (without treatment; however, 5 mice received hydrogel dressing without peptide), 1 group as a positive control (2% mupirocin treatment), and 2 groups as test groups. To establish skin infection, 200 μL of bacterial suspension with 3 × 108 CFU/mL concentration was subcutaneously injected in the scapular region of the mice. On the basis of the in vitro minimal bactericidal concentration of the alginate hydrogel containing peptide for 15 clinical isolates, hydrogel containing 128 mg/L of peptide was used for wound dressing over an 8-day period.Results:
The highest and lowest numbers of wounds were observed on day 2 in the negative and positive control groups, respectively. During the 8-day period, the positive control and hydrogel containing peptide treatment groups showed similar levels of wound healing.Conclusions:
This study showed that compared to standard drug treatment, treatment with hydrogel containing peptide had substantial antibacterial effects on S. aureus wound infections in mice.Keywords
Alginate Antibacterial Peptide Hydrogel Wound Infection Staphylococcus aureus
1. Background
Antibiotic resistance is an increasing threat to the general wellbeing of patients. In clinics, 190 million measurements of antitoxins are performed every day. Among nonhospitalized patients, more than 133 million courses of anti-infective agents are recommended by specialists every year. It is assessed that 50% of the recent medicines will be ineffective as they are being recommended for colds, hacking coughs, and other viral diseases (1, 2). Staphylococcus aureus is considered to be the principle etiological agent and most prevalent microorganism involved in clinic-acquired infections (3), and about 1% of the S. aureus infections in patients are caused by methicillin-resistant Staphylococcus aureus (MRSA) (4, 5). Methicillin, a form of penicillin, was introduced to counter the increasing problem of penicillin-resistant S. aureus. In general, MRSA is resistant to an entire class of penicillin-like antibiotics called beta-lactams, including penicillin, amoxicillin, oxacillin, and methicillin (6, 7).
Since MRSA infections are a health problem worldwide, it is necessary to develop new antimicrobial compounds. Considering broad-spectrum antimicrobial activity and low probability of drug resistance to peptides, applications these peptides are being studied extensively. These peptides are produced by macrophages and epithelial cells in humans and have antimicrobial, antifungal, antiviral, and antiprotozoal activity and are important components of the body’s natural defense mechanism against pathogens. In recent years, antimicrobial peptides (AMPs), which are considered to be novel antibiotics with strong bactericidal properties, have been used against antibiotic-resistant bacteria, and some of these are being evaluated in clinical trials (8).
Previous studies led to the identification of the CM11 peptide (WKLFKKILKVL-NH2), a short cecropin-melittin hybrid peptide, with effective antibacterial activity against some Gram-negative and Gram-positive pathogenic bacteria such as MRSA (9, 10). Time-dependent release formulations for drug delivery are useful for slowing down or controlling the release of drugs to maintain the drug levels within the therapeutic window and prevent potentially hazardous peaks in drug concentrations. Different strategies are used to develop materials for time-dependent drug delivery; natural hydrogels (11), which are exceptionally hydrated biomaterials, are typically composed of polysaccharides such as alginate, dextran, and chitosan and have numerous advantages over synthetic hydrogels in that they are biocompatible and biodegradable and have bacteriostatic, wound-healing, and mechanical properties similar to those of natural tissues (12). Therefore, hydrogels are widely used and impregnated with various compounds including antimicrobial agents such as AMPs, silver ions, and polycationic polymers, which are released over time and confer antimicrobial properties to the hydrogels (13).
2. Objectives
On the basis of data reported by previous studies (9, 10), we evaluated the wound healing effects of an alginate sulfate-based hydrogel impregnated with the CM11 peptide on skin infections caused by MRSA strains in a mouse model.
3. Materials and Methods
3.1. Peptide Synthesis
Solid-phase synthesis was performed using Rink p-methyl benzhydrylamine resin (Sigma-Aldrich, USA). The peptide was purified by reversed-phase semi-preparative high-performance liquid chromatography (CECIL, UK) on a C18 column (9).
3.2. Methicillin-Resistant Bacteria Screening
The Kirby-Bauer agar disk diffusion test was used for screening methicillin-resistant bacterial isolates (human skin infection) on Mueller-Hinton agar (Merck, Germany) by using 0.2 mL of bacterial inoculums (1.5 × 105 cells/mL). Specific antibiotic disks (MAST, UK) were selected according to the guidelines of the Clinical and Laboratory Standards Institute (CLSI, 2012; Table 1). After incubation at 37°C for 24 hour, the resistant bacterial strains (30 isolates) were determined using the CLSI guidelines (10).
Antibiotic Resistance Patterns of Staphylococcus aureus Isolates a
Antibiotic Disk | Percentage of Resistance in Isolates |
---|---|
Kanamycin | > 80 |
Ceftazidime | -- |
Penicillin | > 90 |
Rifampin | > 70 |
Gentamicin | > 80 |
Amikacin | -- |
Ciprofloxacin | > 75 |
Imipenem | -- |
Norfloxacin | > 80 |
Cefotaxime | -- |
Chloramphenicol | -- |
Ampicillin | > 90 |
Mupirocin | > 75 |
3.3. Mupirocin-Sensitive Bacteria Screening
Mupirocin (Gilaranco, Iran) was used for the treatment of skin infection in the positive control group, and drug resistance was evaluated (14). For determining mupirocin-sensitive S. aureus isolates, the disk diffusion method using Mueller-Hinton agar (Merck, Germany) and 5-µg mupirocin paper disks (MAST, UK) was performed. The sensitivity patterns of the mupirocin-sensitive strains were analyzed using E-test® mupirocin strips (AB BIODISK, Solna, Sweden) for assessing the minimal inhibitory concentrations (MICs) of the S. aureus isolates to mupirocin. The E-test strips were placed onto the Mueller-Hinton agar plates inoculated with 0.5 McFarland suspensions of the selected isolates. After incubation at 37°C for 24 hours, the E-test MICs were read at the point of intersection between the edge of the zone of bacterial growth and the E-test strip Strains were considered to be powerless if the MIC was ≤ 4 mg/L, and levels of mupirocin safety were characterized as low-level with MIC 8 - 256 mg/L. Multidrug-resistant isolates were defined as MRSA and methicillin-sensitive S. aureus isolates that were resistant to 3 antibiotics including kanamycin, ciprofloxacin, and penicillin (15).
3.4. Polymerase Chain Reaction
Using polymerase chain reaction (PCR), the selected strains were analyzed for the presence of the mecA gene, which is an essential methicillin resistance gene in S. aureus (16). The PCR mixture consisted of 15 µL of 2X Master. Mix Red (Ampliqon III, Denmark) containing 1.5 mM of MgCl2, 1 µL of DNA solution, and 20 pmol of F (5-GTAGAAATGACTGAACGTCCGATAA-3) and R (5-CCAATTCCACATTGTTTCGGTCTAA-3) primers (SinaClon, Iran) (Table 1) to a final volume of 30 µL. The PCR protocol consisted of the following steps: incubation at 95°C for 5 minutes, 30 cycles of denaturation at 94°C for 30 seconds, annealing at 58°C for 30 seconds, and elongation at 72°C for 30 seconds.
3.5. Determination of MICs and Minimum Bactericidal Concentrations of Peptide
Peptide in saline-phosphate buffer (pH 7.2) (1 mg/mL) were diluted (2 to 128 mg/L) to 1.5 × 105 CFU/mL inoculums in Mueller-Hinton broth and incubated at 37°C for 18 hours.
3.6. Alginate Sulfate Hydrogel Synthesis
For synthesis of alginate sulfate, 10 gr of sodium alginate (Merck, Germany) was added to a sulfate reagent containing 80 mL of formamide and 20 mL of chlorosulfonic acid; the mixture was maintained at 60°C for 4 hours until the mixture color turned brown. Then, 200 mL of acetone was added to the solution sediment, and the sediment was dissolved in distilled water. The pH was set to 10 - 11, and the solution was dialyzed and concentrated for 72 hours. For synthesis of the alginate sulfate hydrogel, 0.6 gr of alginate sulfate was added in 40 mL of ion-free water, and this solution was mixed at 45°C for 10 minutes at 600 rpm until the solid compound dissolved. After dissolving, 0.225 gr of liquid ethylene glycol was added to the alginate sulfate solution, and the solution was stirred with a magnetic stirrer for 10 minutes at 200 rpm. Next, for degassing and completely evaporating the solvent, the mixture was placed in a vacuum oven for 24 hours at 40°C. Then, 2% CaCl2 solution was added on an alginate film in a Petri plate, and then, the solution was removed and the film was washed with ion-free water. Hydrogel films were placed in tubes containing 10 mL of phosphate-buffered saline (PBS; pH = 7.4) at 37°C. Finally, the structure of the sulfate hydrogel was characterized by Fourier transform infrared (FTIR) (PerkinElmer, USA) and nuclear magnetic resonance (NMR) (Bruker, Germany) spectroscopy (17).
3.7. Alginate Sulfate Formulation with Peptide
Peptide was incorporated in the hydrogel matrices by in-situ loading. Peptide was at 2 × minimum bactericidal concentration (MBC) and 4x MBC was added to the alginate and alginate sulfate (0.6 g), respectively, dissolved in 40 mL of ion-free water, and the mixture was stirred at 45°C for 10 minutes at 600 rpm for dissolving the solid compound. The other procedures were performed as described in the previous section, and hydrogel network formulation and drug encapsulation were accomplished simultaneously.
3.8. Antimicrobial Assay Using Sulfate Hydrogel Containing Peptide
For the antimicrobial assay, the hydrogel films containing 2x and 4x MBC concentrations of peptide were cut into circles of 1.5 cm diameter, and then, 10 µL of microbial suspension (1.5 × 105 CFU/mL) was spread onto the hydrogel films in the tissue culture plate. The inoculated hydrogel films were incubated at 37°C for 1 hours at a relative humidity of not less than 90%. Next, 1 mL of neutralizing broth was added to recover the microbial isolates. Tenfold dilution series were prepared, and aliquots of the dilution series were plated onto Luria-Bertani agar. The plates were incubated at 37°C for 48 hours, and the colony-forming units were counted. A hydrogel film without peptide was used as a control. The results are expressed as described previously (18).


3.9. Time-Dependent Release Assay
The time-dependent release of Peptide was from the prepared hydrogels (alginate and alginate sulfate) was determined using the diffusion method. Hydrogels containing peptide (2x MBC of peptide) were placed in PBS buffer at predetermined intervals over the 3-week period; the supernatant was removed and fresh buffer was added. The AMP concentration was determined using an enzyme-linked immunosorbent assay reader (Stat Fax, USA) at 450 nm. The amount of peptide in each sample was measured by standard curves plotted using standard serial dilutions and related optical density (OD). Finally, peptide concentrations were obtained by comparing the sample ODs to a standard curve. According to the time release of 50% of peptide concentration in the time period, we formulated hydrogel with peptide in a final concentration.
3.10. Treatment of Wound Infection in Mice by Using Hydrogel Containing Peptide
All animal procedures were performed according to the guidelines of the Care and Use of Laboratory Animals. Forty 8-week-old female ICR mice (25 - 20 gr) (Pasteur Institute of Iran) were used. The mice were divided into 4 groups: 1 group as a negative control (without treatment; however, 5 mice received hydrogel dressing without peptide), 1 group as a positive control (2% mupirocin treatment), and 2 groups as test groups. To establish skin infection, 200 µL of bacterial suspension (selected isolates with the highest resistance to antibiotics) with 3 × 108 CFU/mL concentration was subcutaneously injected in the scapular region of the mice. After skin infection was established, the wound sites in the test groups were dressed using sulfate hydrogel containing peptide over a 2-week period in order to evaluate the antimicrobial activity and time-dependent release of the peptide.
4. Results
4.1. Antibiotic Resistance Assay
The results were analyzed according to the CLSI standards for bacterial isolates. The MRSA strain with the highest antibiotic resistance and mupirocin sensitivity was selected for injection. The results are summarized in Table 1.
4.2. Determination of MIC and MBC
The MIC and MBC of the CM11 peptide were 2 - 32 mg/L and 16 - 64 mg/L, respectively, for the antibiotic-resistant strains of S. aureus. More than 90% of the strains were susceptible to the CM11 peptide at an MIC of 16 mg/L and MBC of 32 mg/L. The results are summarized in Table 2.
MIC and MBC of the CM11 Peptide for S. aureus Isolates a
Peptide | MIC, mg/L | MBC, mg/L | ||||
---|---|---|---|---|---|---|
Range | 50% | 90% | Range | 50% | 90% | |
CM11 | 2 - 32 | 4 | 8 | 16 - 64 | 16 | 32 |
4.3. PCR Amplification
After selection of the candidate strains, the mecA gene in the strains was amplified by PCR. The results show that amplification of mecA (310 bp) was positive in these strains (Figure 1).
Analysis of mecA Gene Amplification in S. aureus By Using 0.8% Agarose Gel
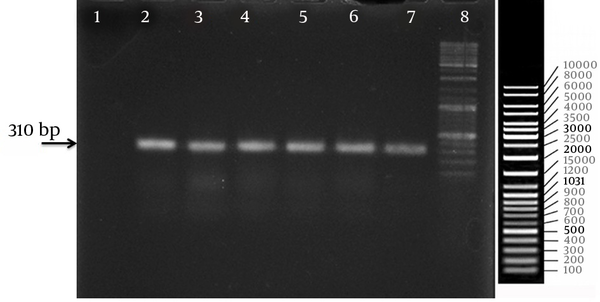
4.4. FTIR Spectra of Sodium Alginate Sulfate
The FTIR spectra of alginate and alginate sulfate with Difference Spectroscopy = 1.4 are shown in Figure 2. The spectra showing two trademark retention groups, one is 600 cm-1 that depict an uneven S=O extending vibration and the other at 520 cm-1 showing a symmetrical C-O-S vibration related to a C-O. In the FTIR spectrum of alginate, these 2 frequencies were absent, which proved that the product was alginate sulfate (19).
FTIR Transmission Spectra of Alginate and Alginate Sulfate
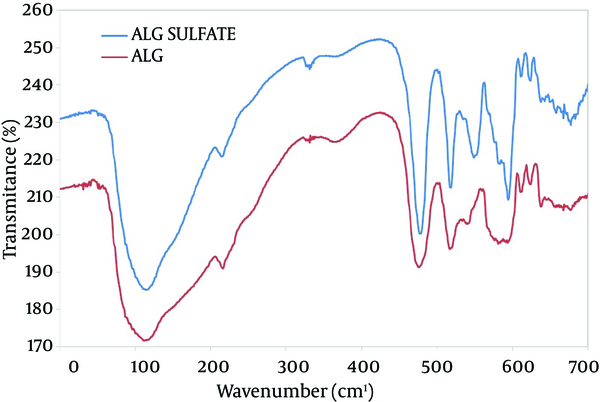
4.5. 13C NMR Spectra of Alginate Sulfate
The NMR spectra presented in Figure 3 confirm the successful synthesis of alginate sulfate hydrogel. The chemical shifts of C-1 and C-6 were 175 and 72 ppm, respectively, while those of C-2 - C-5 were between 65 and 85 ppm. The substance movements of alginate were muddle, however in the wake of being sulfates got to be more entangled on the grounds that the carbons C-2 and C-3 which specifically appending to electronegative sulfate ester gatherings would move to lower field position, while C-4 and C-5 were in a roundabout way connecting to sulfate ester gatherings would move to higher field position (19). Sulfation of the hydroxyl assembles 12 at C-3, C-2 would likewise make the synthetic movement diverse. So the sodium alginate sulfates had various sorts of carbons.
13C NMR Spectra of Alginate and Alginate Sulfate
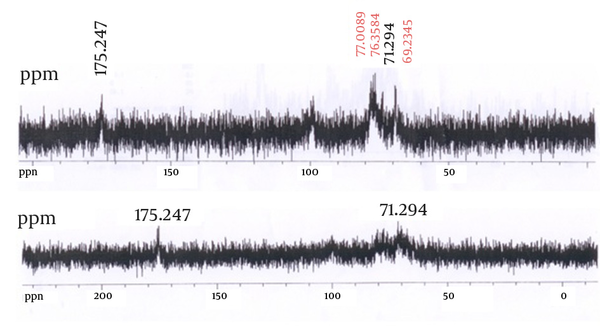
4.6. Antimicrobial Assay
The sulfate hydrogels were incubated with S. aureus strains at a concentration of about 3 × 106 CFU/cm2, and reductions in cell counts were recorded after 1 hour. The results showed the antimicrobial effects of the sulfate hydrogel films containing the peptide. However, increasing the peptide concentration to increase the MBC to 4 × MBC increased the killing efficacy against S. aureus. The 2 hydrogels with 2 × MBC and 4× MBC had excellent log reductions of > 3.0 (> 99% kill), while log reduction in MBC was > 1.0 (Figure 4).
Antimicrobial Assay of Alginate Sulfate Hydrogel Containing Peptide Based on Log Reduction and %Kill
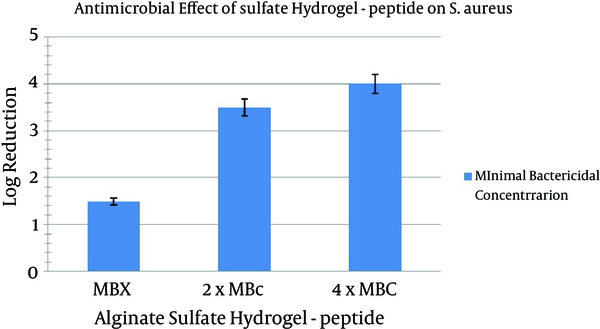
4.7. Time-Dependent Release of Peptide
To assess the controlled delivery of peptide from alginate and alginate sulfate hydrogels and evaluate the effect of the sulfate group on peptide release, the sustained diffusion of peptide in PBS buffer following zero order kinetics releasing the peptide payload was analyzed over a 3-week period. The results show that peptide release from the alginate hydrogel (100%) was faster than that from the alginate sulfate hydrogel (50%) at the end of the first week. However, peptide release from the alginate sulfate hydrogel lasted over 3 weeks (Figure 5).
Time-Dependent Release of Peptide from Alginate (AL) and Alginate Sulfate (AS) Hydrogels over a 3-Week Period
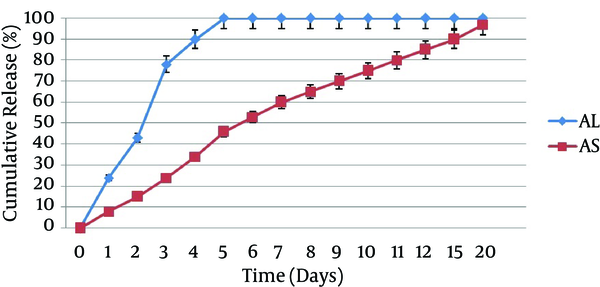
4.8. Peptide Treatment of Wound Infection
The highest and lowest numbers of wounds are shown in Table 3. In the negative control group, no differences in wound healing were observed between mice with and those without hydrogel dressing. During the 2-week period, the positive control group showed improved wound healing, which was similar to that observed in the groups treated with the alginate sulfate hydrogel containing peptide. The degree of wound healing was similar between the groups treated with the hydrogel containing peptide. In the course of the recovery process after opening the wound, the crust was formed on some wounds because after skin lesions Mesoderm blood vessels may be damaged and cause bleeding and Blood leaked from blood vessels, leading to the formation of clots.
Wounds Observed in the Mice after Bacterial Injection over a 2-Week Period
Groups | Mice | Day 2 | Day 8 | Day 15 |
---|---|---|---|---|
Negative control (no treatment) | 9 | 6 | 5 | 5 |
Positive control (2% mupirocin treatment) | 7 | 2 | 0 | 0 |
Treatment with hydrogel containing peptide (2 × MBC) | 9 | 4 | 2 | 0 |
5. Discussion
Staphylococcus aureus and other Staphylococcus are considered to be the most common bacteria causing wound infections. Many minor skin infections (i.e., localized infections) caused by S. aureus can be treated with topical agents such as mupirocin and retapamulin; however, most MRSA strains are resistant to these and other conventional antibiotics. Cationic AMPs and their synthetic derivatives are promising candidates to combat antibiotic resistance (8, 20, 21). We investigated the wound-healing efficacy of CM11, a short hybrid cationic peptide, against S. aureus skin infection. Our previous study showed that this peptide is effective against bacterial infections, but only in the in vivo environment. Therefore, we investigated the in vitro cytotoxic effects of the CM11 peptide on some eukaryotic cells (RAJI, CHO, Hela, SP2/0, and LNCAP) by using the MTT assay (9, 10). At low concentrations, this peptide showed few effects on the cell lines, but increasing the peptide concentration led to an increase in the cytotoxic effects on the eukaryotic cells.
Use of time-dependent release formulations for drug delivery may help prevent potentially hazardous peaks in drug concentrations. Hydrogels are powerful agents that can be formulated in a variety of physical forms with the potential property to control the release of impregnated compounds (22). During the 1970s, the human amniotic membrane was first used as a wound dressing in burn patients, which promoted rapid re-epithelialization and healing of wounds (23). In 1986, Sparkes et al. (24) first described the use of a hydrogel consisting of a blend of gelatin and chitosan, which are biological polymers, for wound healing. Many studies have reported the use of implant coatings or hydrogel dressings for wound and skin lesion healing. Marchesan et al. (25) described a self-assembled antimicrobial hydrogel containing a hydrophobic tripeptide (DLeu-Phe-Phe). The gel showed antibacterial activity against S. aureus, Escherichia coli, and a clinical strain of Klebsiella pneumoniae without significant cytotoxicity against human red blood cells or mouse fibroblast cell cultures. Meng et al. (26) investigated the impact of a gathering toward oneself peptide nanofiber platform (RADA16-I) for the treatment of severely charred skin in rats. They indicated that the use of RADA16-I dressing decreased the level of edema of burn wounds, accelerated the formation and debridement of eschar, and quickened wound constriction.
Sodium alginate and its derivatives are water-soluble anionic polymers that can be used to deliver microspheres, globules, and microcapsules and can be used as dressings to maintain a physiologically moist microenvironment and minimize bacterial infection at wound sites, facilitating wound healing (17, 27). Charge associations between ionic polymers and charged medications improve the quality of the relationships between a gel and a target medication. Therefore, sulfation of alginate can lead to induction of the ionic property of hydrogel and so increase the interaction. Alginate sulfate is structurally similar to heparin and has a high affinity for certain growth factors, leading to the absorption and accumulation of heparin-binding growth factors. Unlike chitosan, alginate is inherently nondegradable in mammals (28). Novel alginate dressings in the dry form absorb wound fluid to re-gel, and the gels can supply water to a dry wound, maintaining a physiologically moist microenvironment and minimizing bacterial disease at the injury site. These properties can promote granulation tissue development, rapid epithelialization, and recuperation (13).
Our results show that 50% of the peptide from the alginate sulfate gel was released during the first week and peptide release continued over a 3-week period, whereas 100% of the peptide from the alginate gel was released before the first week; this demonstrates the suitable effects of gel sulfation on the delay in peptide release. The antimicrobial assay demonstrated the antibacterial effects of the peptide against S. aureus. However, for MBC concentration log reduction was above 1 with more than 90% bacteria killing while this parameter for 2 × MBC and 4 × MBC concentrations were above 3 and 4 respectively which showing > 99% bacteria killing. In this study, we used the hydrogel containing peptide with 4 × MBC to increase the time-dependent release of peptide.
The results show that wound infection completely healed in the positive control and test groups over the 8-day and 12-day periods, respectively; therefore, wounds treated with mupirocin healed faster than those treated with gel containing peptide. Lee et al. (29) determined the MIC of HG1 for an MRSA strain (4 µg/mL) and showed that HG1 administration onto the skin wound showed antimicrobial effects and successfully healed the infection site. Demidova-Rice et al. (30) reported that the use of a combination of 2 peptides, i.e. UN3 and comb1, stimulated wound healing in mouse models. Further advancement of these peptides could prompt another treatment for endless and intense wounds. Finally, with attendance to antibacterial efficacy of peptides with high potential therapeutic it seems that in the futures peptides used as alternative agents for treatment of infection diseases.
Acknowledgements
References
-
1.
Dzidic S, Suskovic J, Kos B. Antibiotic resistance mechanisms in bacteria: biochemical and genetic aspects. Food Tec Bio. 2008;46(1):11-21.
-
2.
Erami M, Soltani B, Taghavi Ardakani A, Moravveji A, Haji Rezaei M, Soltani S, et al. Nasal Carriage and Resistance Pattern of Multidrug Resistant Staphylococcus aureus Among Healthy Children in Kashan, Iran. Iran Red Crescent Med J. 2014;16(9). eee21346. [PubMed ID: 25593734]. https://doi.org/10.5812/ircmj.21346.
-
3.
Bode LG, Kluytmans JA, Wertheim HF, Bogaers D, Vandenbroucke-Grauls CM, Roosendaal R, et al. Preventing surgical-site infections in nasal carriers of Staphylococcus aureus. N Engl J Med. 2010;362(1):9-17. [PubMed ID: 20054045]. https://doi.org/10.1056/NEJMoa0808939.
-
4.
Zolfaghari PS, Packer S, Singer M, Nair SP, Bennett J, Street C, et al. In vivo killing of Staphylococcus aureus using a light-activated antimicrobial agent. BMC Microbiol. 2009;9:27. [PubMed ID: 19193212]. https://doi.org/10.1186/1471-2180-9-27.
-
5.
Boyce JM. Environmental contamination makes an important contribution to hospital infection. J Hosp Infect. 2007;65 Suppl 2:50-4. [PubMed ID: 17540242]. https://doi.org/10.1016/S0195-6701(07)60015-2.
-
6.
Rivera AM, Boucher HW. Current concepts in antimicrobial therapy against select gram-positive organisms: methicillin-resistantstaphylococcus aureus, penicillin-resistant pneumococci, and vancomycin-resistant enterococci. United States: Elsevier; 2011.
-
7.
Stefani S, Chung DR, Lindsay JA, Friedrich AW, Kearns AM, Westh H, et al. Meticillin-resistant Staphylococcus aureus (MRSA): global epidemiology and harmonisation of typing methods. Int J Antimicrob Agents. 2012;39(4):273-82. [PubMed ID: 22230333]. https://doi.org/10.1016/j.ijantimicag.2011.09.030.
-
8.
Moghaddam MM, Aghamollaei H, Kooshki H, Barjini KA, Mirnejad R, Choopani A. The development of antimicrobial peptides as an approach to prevention of antibiotic resistance. Rev Med Microbio. 2015;26(3):98-110. https://doi.org/10.1097/mrm.0000000000000032.
-
9.
Moghaddam MM, Abolhassani F, Babavalian H, Mirnejad R, Azizi Barjini K, Amani J. Comparison of in vitro antibacterial activities of two cationic peptides CM15 and CM11 against five pathogenic bacteria: Pseudomonas aeruginosa, Staphylococcus aureus, Vibrio cholerae, Acinetobacter baumannii, and Escherichia coli. Probiotics Antimicrob Proteins. 2012;4(2):133-9. https://doi.org/10.1007/s12602-012-9098-7.
-
10.
Moghaddam MM, Barjini KA, Ramandi MF, Amani J. Investigation of the antibacterial activity of a short cationic peptide against multidrug-resistant Klebsiella pneumoniae and Salmonella typhimurium strains and its cytotoxicity on eukaryotic cells. World J Microbiol Biotechnol. 2014;30(5):1533-40. [PubMed ID: 24323118]. https://doi.org/10.1007/s11274-013-1575-y.
-
11.
Amani J, Barjini KA, Moghaddam MM, Asadollahi A. In vitro synergistic effect of the CM11 antimicrobial peptide in combination with common antibiotics against clinical isolates of six species of multidrug-resistant pathogenic bacteria. Protein Pept Lett. 2015. [PubMed ID: 26216264].
-
12.
Qiu Y, Park K. Environment-sensitive hydrogels for drug delivery. Adv Drug Delivery Rev. 2012;64:49-60. https://doi.org/10.1016/j.addr.2012.09.024.
-
13.
Salome Veiga A, Schneider JP. Antimicrobial hydrogels for the treatment of infection. Biopolymers. 2013;100(6):637-44. [PubMed ID: 24122459]. https://doi.org/10.1002/bip.22412.
-
14.
Perl TM, Cullen JJ, Wenzel RP, Zimmerman MB, Pfaller MA, Sheppard D, et al. Intranasal mupirocin to prevent postoperative Staphylococcus aureus infections. N Engl J Med. 2002;346(24):1871-7. [PubMed ID: 12063371]. https://doi.org/10.1056/NEJMoa003069.
-
15.
Mohajeri P, Gholamine B, Rezaei M, Khamisabadi Y. Frequency of Mupirocin Resistant Staphylococcus aureus Strains Isolated From Nasal Carriers in Hospital Patients in Kermanshah. Jundishapur J Microbiol. 1970;5(4):560-3. https://doi.org/10.5812/jjm.4199.
-
16.
Zhang K, McClure JA, Elsayed S, Louie T, Conly JM. Novel multiplex PCR assay for characterization and concomitant subtyping of staphylococcal cassette chromosome mec types I to V in methicillin-resistant Staphylococcus aureus. J Clin Microbiol. 2005;43(10):5026-33. [PubMed ID: 16207957]. https://doi.org/10.1128/JCM.43.10.5026-5033.2005.
-
17.
Fan L, Jiang L, Xu Y, Zhou Y, Shen Y, Xie W, et al. Synthesis and anticoagulant activity of sodium alginate sulfates. Carbohydr Polym. 2011;83(4):1797-803. https://doi.org/10.1016/j.carbpol.2010.10.038.
-
18.
Li P, Poon YF, Li W, Zhu HY, Yeap SH, Cao Y, et al. A polycationic antimicrobial and biocompatible hydrogel with microbe membrane suctioning ability. Nat Mater. 2011;10(2):149-56. [PubMed ID: 21151166]. https://doi.org/10.1038/nmat2915.
-
19.
Ronghua H, Yumin D, Jianhong Y. Preparation and in vitro anticoagulant activities of alginate sulfate and its quaterized derivatives. Carbohydr Polym. 2003;52(1):19-24. https://doi.org/10.1016/s0144-8617(02)00258-8.
-
20.
Gordon YJ, Romanowski EG, McDermott AM. A review of antimicrobial peptides and their therapeutic potential as anti-infective drugs. Curr Eye Res. 2005;30(7):505-15. [PubMed ID: 16020284]. https://doi.org/10.1080/02713680590968637.
-
21.
Motevasel M, Okhovat MA, Zomorodian K, Farshad S. A Study of the Effect of Zataria multiflora Extract on Methicillin Resistant Staphylococcus aureus. Jundishapur J Microbiol. 2013;6(5). eee5453. https://doi.org/10.5812/jjm.5453.
-
22.
Hoare TR, Kohane DS. Hydrogels in drug delivery: Progress and challenges. Polymer. 2008;49(8):1993-2007. https://doi.org/10.1016/j.polymer.2008.01.027.
-
23.
Dai T, Huang YY, Sharma SK, Hashmi JT, Kurup DB, Hamblin MR. Topical antimicrobials for burn wound infections. Recent Pat Antiinfect Drug Discov. 2010;5(2):124-51. [PubMed ID: 20429870].
-
24.
Sparkes BG, Murray DG, inventors. Chitosan, gelatin and compatinle plasticizer. Google Patents. 1986.
-
25.
Marchesan S, Easton CD, Styan KE, Waddington LJ, Kushkaki F, Goodall L, et al. Chirality effects at each amino acid position on tripeptide self-assembly into hydrogel biomaterials. Nanoscale. 2014;6(10):5172-80. [PubMed ID: 24700146]. https://doi.org/10.1039/c3nr06752a.
-
26.
Meng H, Chen L, Ye Z, Wang S, Zhao X. The effect of a self-assembling peptide nanofiber scaffold (peptide) when used as a wound dressing for the treatment of deep second degree burns in rats. J Biomed Mater Res B Appl Biomater. 2009;89(2):379-91. [PubMed ID: 18837444]. https://doi.org/10.1002/jbm.b.31226.
-
27.
Lee KY, Mooney DJ. Alginate: properties and biomedical applications. Prog Polym Sci. 2012;37(1):106-26. [PubMed ID: 22125349]. https://doi.org/10.1016/j.progpolymsci.2011.06.003.
-
28.
Sargus-Patino C. Alginate hydrogel as a three-dimensional extracellular matrix for in vitro models of development. United States: University of Nebraska-Lincoln; 2013.
-
29.
Lee YS, Shin YP, Shin SH, Park S, Kim MH, Lee IH. Therapeutic efficacy of halocidin-derived peptide HG1 in a mouse model of surgical wound infection with methicillin-resistant Staphylococcus aureus. Antimicrob Agents Chemother. 2011;55(3):1296-9. [PubMed ID: 21220538]. https://doi.org/10.1128/AAC.00948-10.
-
30.
Demidova-Rice TN, Wolf L, Deckenback J, Hamblin MR, Herman IM. Human platelet-rich plasma- and extracellular matrix-derived peptides promote impaired cutaneous wound healing in vivo. PLoS One. 2012;7(2). ee32146. [PubMed ID: 22384158]. https://doi.org/10.1371/journal.pone.0032146.