Abstract
Background:
Due to long-term treatment of dermatophytic lesions, such as tinea unguium and using antifungal drugs, which cause side effects, it is essential to investigate new antifungal drugs with greater absorption and fewer side effects, such as nano-drugs. Trichophyton rubrum and Microsporum canis are important dermatophyte species. To investigate new strategy treatment, researchers have tried to find a new drug with extensive therapeutic effects and short-duration treatment. In this context, nano-drugs have gained great interest in improving the efficacy of antifungal properties compared to commercial drugs.Objectives:
The aim of this study was to compare the effects of free terbinafine and nano-liposomal terbinafine on growth of clinical isolates of T. rubrum and M. canis.Methods:
In this study, 120 isolates of T. rubrum and M. canis were separated from dermatophytosis lesions. Diagnosis of the strains was based on the morphological structure and molecular identification. Nano-liposomal terbinafine was prepared and analyzed. Antifungal susceptibility testing was performed by broth micro dilution CLSI M 38-A method to compare inhibitory growth effects of nano-liposomal characteristics of terbinafine and free terbinafine.Results:
The results showed that the Minimum Inhibitory Concentration (MIC) values of terbinafine against T. rubrum and M. canis strains were 0.0625 to 1 μ/mL and 0.0313 to 0.5 μ/mL, respectively. Also, the MIC values of nano-liposomal terbinafine against T. rubrum and M. canis were 0.0156 to 0.25 μ/mL and 0.0078 to 0.125 μ/mL, respectively.Conclusions:
Comparison of the antifungal effects of nano-liposomal terbinafine and free terbinafine against T. rubrum and M. canis isolates showed that the nanopartilces of terbinafine had a greater antifungal activity against the studied isolates and can be used as an alternative agent for dermatophytosis treatments.Keywords
Antifungal Activity Nanoparticles Terbinafine Microsporum canis Trichophyton rubrum
1. Background
Dermatophytosis is one of the most common fungal infections of keratinized human and animal tissues, which is caused by dermatophytes as a group of filamentous fungi (1). An inflammatory scalp infection was the first report of dermatophytosis by Auluscornelius Celsusin 30 A.D. (2). Dermatophyte infections are limited to the stratum corneum and the infections range from acute to chronic infections. Among these fungal pathogens, Trichophyton rubrum was the most common etiological agent of tinea unguium and the second causative agent of tinea corporis and tinea cruris from clinical isolation during 2010 to 2014 in Tehran, Iran (3), in addition to the most common isolated dermatophytes in other European countries (4-6). Trichophyton rubrum infections are often intractable and associated with relapses after discontinuation of antifungal therapy (7). Another dermatophyte agent is Microsporum canis, which was the earnest epidemiologic problem in Europe and Australia, with increased incidence observed recently (8). According to Fallahi et al. (2017), M. canis was one of the etiological agents of tinea capitis in Guilan, Iran (9).
Terbinafine is a well-known allylamine agent used for the treatment of superficial fungal infections (10), yet some infections cannot be successfully treated with terbinafine. Recently, Mukherjee et al. (11) reported an increased trend of minimum inhibitory concentrations (MICs) of T. rubrum strains against terbinafine. A long duration of treatment with terbinafine in some infections and its side effects are the main reasons for the need for a new antifungal drug.
In the recent years, studies on nanoparticles loaded with drugs have been performed. Mendes et al. (12) applied nanostructured lipid carriers loaded with miconazole for increasing the antifungal activity of miconazole. Also, Gupta et al. (13) pointed out that solid lipid nanoparticles loaded with fluconazole are other carriers, which were used as a drug delivery system. Using liposome-loaded amphotericin B in addition to reduction of nephrotoxicity by forming amphotericin B lipid complex, leads to an increase of plasma drug concentration and reduction of clearance; therefore, extended half-life elimination of the drug was observed (14).
The lipid nanoparticles are safe carries and can improve the bioactivity of drugs by control release of drugs, which leads to increasing bioavailability. The small size of these lipid nanoparticles increases the effect delivery in target cells and reduces adverse effect on other cells (15). Therefore, nanoliposome carries loaded with drugs can be considered as alternative treatments for fungal infections.
2. Objectives
The aim of the present study was to examine the antifungal activities of nano-liposomel terbinafine versus free terbinafine against T. rubrum and M. canis species isolated from human and animal specimens.
3. Methods
3.1. Ethics Statement
The study was approved by the ethics committee of Tehran University of Medical Science (IR.TUMS.SPH.Rec1395.1339).
3.2. Dermatophyte Isolates
In this experimental study, 60 isolates of T. rubrum were collected from nail, hair, and skin of patients (Table 1) with symptoms of dermatophytosis referred to the mycology lab of Tehran University of Medical Sciences and another private laboratory (Medical mycology laboratory of Dr S J. Hashemi). Also, 60 isolates of M. canis were taken from infected hair and skin of cats (Table 2) with symptoms of dermatophytosis. These samples were collected from the veterinary clinic of the science and research branch of Tehran Islamic Azad University (Saadat Abad, Tehran, Iran). Trichophyton interdigitale ATCC MYA-4439 was used as a quality control for antifungal susceptibility testing.
Number of Isolates and Lesion Site of T. rubrum
Lesion Site | No. of T. rubrum Isolates |
---|---|
Foot | 18 |
Nail | 15 |
Groin | 8 |
Hand | 6 |
Body | 8 |
Head | 5 |
Total | 60 |
Number of Isolates and Lesion Site of M. canis
Lesion Site | No. of M. canis Isolates |
---|---|
Muzzle | 28 |
Body | 26 |
Claw | 6 |
Total | 60 |
All clinical isolates were cultured on Sabouraud dextrose agar + cyclohexamide + chloramphenicol (QUELAB, Canada) and incubated at 28°C for 2 weeks. Identification of isolates was done using colony appearance for macroscopic characteristics and the slide culture technique for microscopic examination of micro- and macroconidia. Differential tests like urease test and hair perforation were also used. To induce conidia production, all isolates were subcultured in potato dextrose agar (PDA, Merck, Germany) for 7 days at 28°C. Tests were performed using NCCLS (CLSI) broth micro dilution method (M38-A) (16). To prepare stocks, colonies of the isolates were transferred to distilled water in sterile Eppendorfs with 20% glycerol and stored at -20°C in the freezer.
For molecular identification, 85 isolates have already been identified by Passargad Laboratory, and Genomic DNA of the rest of samples (13 T. rubrum and 22 M. canis) were extracted by the “Gene JETTM Plant Genomic DNA Purification Mini Kit” (Fermentas, Germany), according to the manufacturer’s instructions. Two primers, ITS1 5ˊ-TCC GTA GGT GAA CCT GCG G-3ˊ and ITS4 5ˊ TCC TCC GCT TAT TGA TAT GC -3ˊ, were used for PCR analysis (17). The reaction mixture consisted of 10 μL of 2X master mix (Ampliqon III, Denmark), 5 μL DNA template, 2 μL primers and 3 μL double distilled sterile water to 20 μL final volume. The reactions were performed with a thermocycler (BioRad) under the following conditions: samples were heated to 95°C for 3 minutes and then subjected to 35 cycles of 30 seconds at 95°C (denaturation), 30 seconds at 55°C (annealing), and 40 seconds at 72°C (extension). The final extension was set at 72°C for 5 minutes. Trichophyton rubrum PTCC 5143 and M. canis PTTC 5069 were used as controls. The PCR products were electrophoresed on 1.5% agarose gel, stained with ethidium bromide, and illuminated and documented using the BioRad UV trans-illuminator. The obtained PCR fragments were sent to Takapouzist Company (Tehran, Iran) for sequencing and then the results were evaluated for homology analysis by using the Gen Bank (NCBI).
3.3. Preparation of Nano-Liposomal Terbinafine and Nanostructure Analysis
Liposomal formation of terbinafine was prepared at Nanozino Company (18). Overall, 12.8 g of terbinafine (Sigma, Germany) was dissolved in 1 mL Dimethyl Sulfoxide (DMSO) then dissolved in 6 mL of solvent of chloroform-methanol (1:1), and lichitin (Lipoid, Canada) and cholesterol (Sigma, Germany) were added to this solvent. Evaporation of this solvent was done at 50°C. Sucrose contributed to hydration. Homogenization was performed by a homogenizer at 20,000 rpm and at a temperature above the liposomes phase transition (70) for 10 minutes. Sonication of liposomal sample was carried out in an ice bath. Removed amount of drugs that was not loaded was centrifuged at 4000 × g for 4 minutes then was passed for sterilization twice using a 0.22 micron filter needle.
Scanning electron microscope (SEM) was used to determine the size and structure of the prepared nanoliposomal terbinafine. Also, a sputter coater (KYKY SBC-12, Beijing, China) was used to prepare SEM photographs, and then analyzed with SEM (KYKY-EM3200) at 15 KV acceleration voltages. Measurement of zeta potential was also done in this study. To determine the status of superficial electrical dispersions, an appropriate dilution was prepared with saline phosphate and kept at 25°C.
3.4. Antifungal Susceptibility Testing
3.4.1. Inoculum Preparation
Mature colonies grown on PDA were covered by 5 mL of sterile saline (0.85%) with one drop of tween 20 and then the surface of colonies was scarped with a sterile swab. The mixture solution of conidia and hyphal fragment was transferred to a sterile tube and kept for 5 to 10 minutes at room temperature to sediment heavy particles. Afterwards, the upper suspension, which contained conidia, was counted with a hemacytometer and the concentrations were adjusted with RPMI 1640 (this medium was prepared with glutamine and buffer with pH 7.0 and without sodium bicarbonate; 10.43 g of RPMI powder and 34.53 g of MOPS buffer (N-Morpholino Propanesulfoni Acid) were dissolved in 1 L of distilled water with gentle shaking on the flame to standardize the inoculum (1 × 103 to 3 × 103 CFU/mL)).
3.4.2. Antifungal Agents
In this study, the crude powder of terbinafine was obtained from Sigma-Aldrich (Chemical Company, GmbH, Riedstr). Terbinafine was diluted with DMSO (sigma) and RPMI 1640 was used for dilution of the nano-liposomal terbinafine. The antifungal agent’s ranges were from 0.001 to 2 μL/mL.
3.4.3. Minimum Inhibitory Concentration Test Procedure
The antifungal susceptibility method was based on the standard method of Broth Micro dilution CLSI M 38. To perform the test, 96-flat-bottomed well microplates (Orange Scientific, E.U) were used. Then, 100 μL of each drug concentration was inoculated to the wells then 100 μL of inoculum suspension was added to each drug well. Growth control and sterilized control wells were also used for each isolate. Blank nano-liposome was also used to monitor no antifungal activity of thissubstance. Plates were incubated at 28°C for 4 days. Results were considered visually and recorded as MICs; the lowest concentration showed 100% growth inhibition as compared to growth in the control wells. All tests were performed at least twice.
3.5. Data Analysis
The Kolmogorov-Smirnov test and Student’s t-test were used to compare and analyze the data. P values of ≤ 0.05 were considered significant.
4. Results
4.1. PCR Assay
Fungal genomic DNA was extracted from T. rubrum, and M. canis isolates were amplified using internal transcribed spacer 1 (ITS1) and ITS4 primers; the results showed an expected size in the range between 600 and 700 bp (Figure 1). The DNA sequence of the nuclear ribosomal ITS region of the fungus was T. rubrum and M. canis with homology of 99% by using the Blast 2 Sequences Tool.
PCR Products of Some Clinical Isolates of T. rubrum and M. canis Using ITS1 and ITS4 Primes, Lane L: 1500 bp DNA Ladder, Lane 1: T. rubrum Standard Strain ATCC 18759, Lane 2 - 4: T. rubrum Clinical Isolates, Lane 5: M. canis Standard Strain ATCC 36299, Lane 6: M. canis Clinical Isolate.
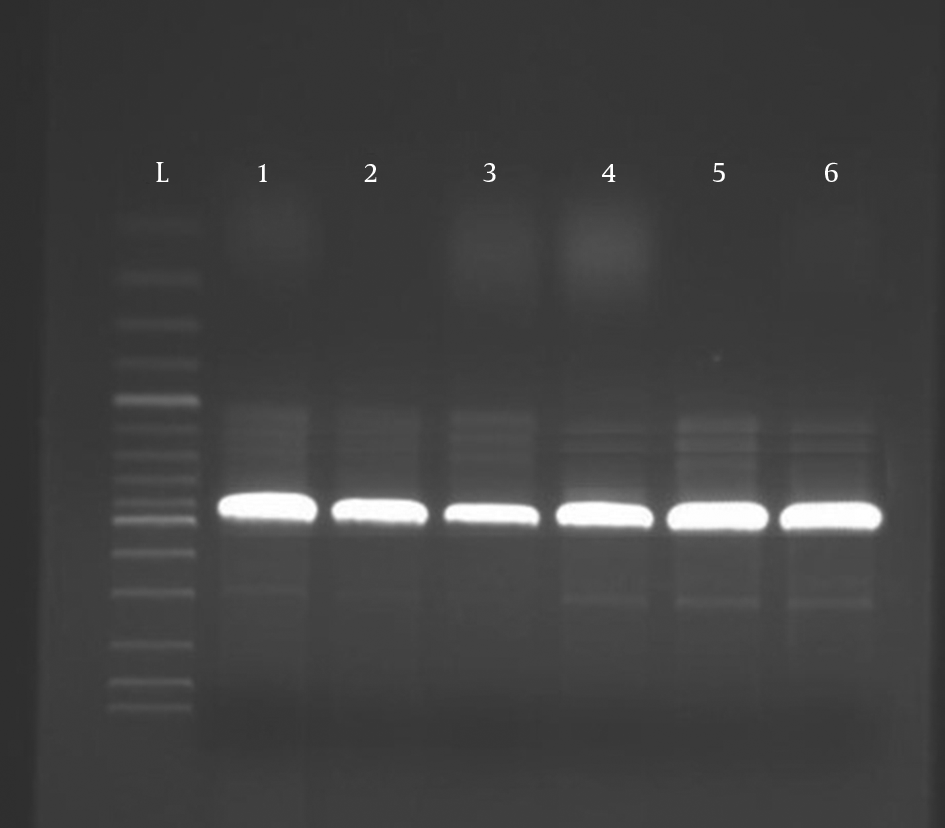
4.2. The Scanning Electron Microscopy and Zeta Potential Results
4.2.1. Scanning Electron Microscopy
The scanning electron microscopy was used to investigate the structure of nano-liposomal terbinafine. The results of higher and lower magnification images of SEM showed that the forms of nanoparticles were spherical and the particle size was 58.8 to 96.3 nm (Figure 2A and 2B).
A, Image of Nanopartilces with High Magnification; B, Image of Nanoparticles with Low Magnifications
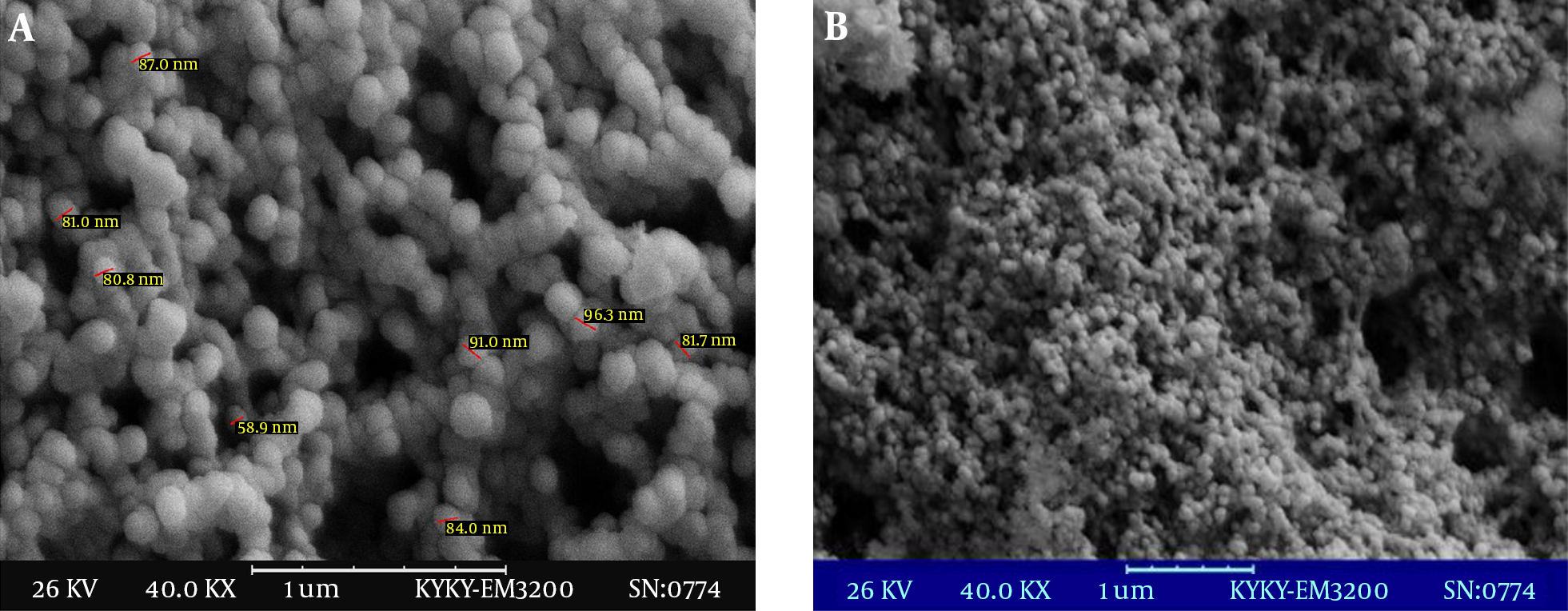
4.3. Zeta Potential
As shown in Figure 3, zeta potential of terbinafine nano-liposimal formation was -21.14.
Curve of Zeta Potential of Liposome Containing Terbinafine
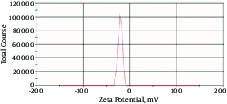
4.4. Antifungal Susceptibility Testing
Table 3 shows the results of the micro dilution test to compare the MICs of terbinafine with nano-liposomal terbinafine for all isolates of T. rubrum and M. canis.
In-Vitro Antifungal Drug Susceptibility of All Isolates of T. rubrum and M. canisa
Species/Antifungal Agent MIC Range, μ/mL | MIC50, μ/mL | MIC90, μ/mL | MICGM |
---|---|---|---|
T. rubrum (n = 60) | |||
Terbinafine 0.0625 - 1 | 0.5 | 1 | 0.2905 |
Nanoterbinafine 0.0156 - 0.25 | 0.0625 | 0.125 | 0.0569 |
M. canis (n = 60) | |||
Terbinafine 0.0313 - 0.5 | 0.125 | 0.5 | 0.174 |
Nanoterbinafine 0.0078 - 0.125 | 0.0313 | 0.125 | 0.04 |
Comparison of the MICs of terbinafine with nano-liposomal terbinafine against M. canis and T. rubrum showed that by the use of nanoparticles, the MIC values of the 2 fungal species were decreased significantly (P < 0.0001) (Figure 4). These results indicated that nanoliposomal terbinafine is more effective than terbinafine in growth inhibition of fungi, so that 30% of the T. rubrum strains were inhibited in 0.0625 μ/mL concentration and 29% of the M. canis strains were inhibited in 0.0313 μ/mL concentration. As shown in Table 1, the highest MIC90 (1 μ/mL) of terbinafine among the examined isolates was for T. rubrum species yet the amount of MIC90 was decreased to 0.125 μ/mL after exposure to nano-liposomal terbinafine.
Mean ± SEM of Terbinafine and Nanoliposomal Terbinafine Againts T. rubrum and M. canis Clinical Isloates
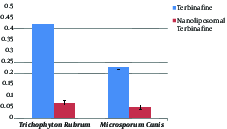
5. Discussion
In the current study, terbinafine was prepared as the nano-liposomal loaded drug, confirmed by SEM and zeta potential. The spherical and nano size of the drug was determined, when examined by SEM. Zeta potential is the electric potential at surrounding of the double layer, which is important for evaluating the stability of the colloidal system. Adding cholesterol can increase the stability of liposomes (18) and on the other hand in the lipid composition of cholesterol and lecithin, zeta potential was negative. In the current examination, it was found that zeta potential was reasonable. In a research by shah et al. in 2017 on liposome formulation, loaded terbinafine zeta potential was -26.8 mV (11). The zeta potential of amphotericin B nanoparticles was between -18 mV and -19 mV, as reported by Tang et al. 2014 (19).
This study observed that some strains of T. rubrum had high MIC values for terbinafine. The highest MIC value (1 μ/mL) obtained in this study for some T. rubrum strains could be explained with resistance mechanisms of pathogenic fungi to terbinafine. Similarly, Mukhrjee et al. (11) pointed out that the clinical strains of T. rubrum were resistant to terbinafine with MIC of > 4. Other reports showed that resistance to terbinafine increased among T. rubrum strains. These findings are similar to the current results, which showed increased MICs of terbinafine in T. rubrum strains (20, 21); several mechanisms have been identified, such as modification of target enzymes by mutation, increased drug efflux, and stress adaptation. In the current study, the most important reason for resistance to terbinafine among the isolates was increased expression of high amounts of transporter membrane proteins, which could reduce the accumulation of drug in the cell. ATP-binding-cassette is the main drug release pump, which is encoded by TruMDR2 genes in T. rubrum resistance isolates (22).
The possible reason for decreasing the MICs of M. canis and T. rubrum strains with application of nanoparticles may be explained by attaching nano-liposomal terbinafine to the cell wall fungi due to hydrophobic outer membrane; therefore, the small sized drug could penetrate to the cell wall of the fungi and can protect from enzymes. Consequently, controlled release of drug is responsible for decreasing MICs of studied strains that were exposed to nanoparticles. In a study by sharma et al. (23), a nanoparticle was used in vivo as a terbinafine carrier in a drug delivery system to improve the efficacy and decrease side effects of terbinafine. In another study, Tayel et al. (24) observed that terbinafine loaded by nanoparticles, as an eye drop, could improve bioavailability of terbinafine and increase drug residence time. In the present study, use of nano-liposome form of terbinafine led to a more effective drug.
6. Conclusions
In conclusion, this is the first report on vitroevaluation of antifungal effects of nanoliposomal terbinafine on M. canis and T. rubrum strains isolated frompatients with dermatophytosis lesions in Tehran. The findings in this study showed better antifungal activity of nano-liposomal terbinafine than the common form of the drug on all isolates of M. canis and T. rubrum; thus, it can be used under in vivo conditions as alternative agent for dermatophytosis treatments.
References
-
1.
Abd Elmegeed ASM, Ouf S, Moussa TA, Eltahlawi S. Dermatophytes and other associated fungi in patients attending to some hospitals in Egypt. Brazil J Microbiol. 2015;46(3):799-805. [PubMed ID: 26413063]. https://doi.org/10.1590/S1517-838246320140615.
-
2.
Cox RA. Immunology of the fungal diseases. CRC Press; 1989.
-
3.
Zamani S, Sadeghi G, Yazdinia F, Moosa H, Pazooki A, Ghafarinia Z, et al. Epidemiological trends of dermatophytosis in Tehran, Iran: A five-year retrospective study. J Mycol Med. 2016;26(4):351-8. [PubMed ID: 27520535]. https://doi.org/10.1016/j.mycmed.2016.06.007.
-
4.
Faure-Cognet O, Fricker-Hidalgo H, Pelloux H, Leccia MT. Superficial Fungal Infections in a French Teaching Hospital in Grenoble Area: Retrospective Study on 5470 Samples from 2001 to 2011. Mycopathologia. 2016;181(1-2):59-66. [PubMed ID: 26452757]. https://doi.org/10.1007/s11046-015-9953-7.
-
5.
Budak A, Bogusz B, Tokarczyk M, Trojanowska D. Dermatophytes isolated from superficial fungal infections in Krakow, Poland, between 1995 and 2010. Mycoses. 2013;56(4):422-8. [PubMed ID: 23346893]. https://doi.org/10.1111/myc.12043.
-
6.
Vena GA, Chieco P, Posa F, Garofalo A, Bosco A, Cassano N. Epidemiology of dermatophytoses: retrospective analysis from 2005 to 2010 and comparison with previous data from 1975. New Microbiol. 2012;35(2):207-13. [PubMed ID: 22707134].
-
7.
Roberts DT, Evans EG. Subungual dermatophytoma complicating dermatophyte onychomycosis. Br J Dermatol. 1998;138(1):189-90. [PubMed ID: 9536247].
-
8.
Zhu M, Li L, Wang J, Zhang C, Kang K, Zhang Q. Tinea capitis in Southeastern China: a 16-year survey. Mycopathologia. 2010;169(4):235-9. [PubMed ID: 19936963]. https://doi.org/10.1007/s11046-009-9260-2.
-
9.
Fallahi AA, Rezaei-Matehkolaei A, Rezaei S. Epidemiological status of dermatophytosis in Guilan, north of Iran. Curr Med Mycol. 2017;3(1):20. [PubMed ID: 29302626]. https://doi.org/10.29252/cmm.3.1.20.
-
10.
Revankar SG, Graybill JR, Patterson TF. Antifungal Agents and Antifungal Susceptibility Testing. Topley Wilson's Microbiol Microb Infect. https://doi.org/10.1002/9780470688618.taw0134.
-
11.
Mukherjee PK, Leidich SD, Isham N, Leitner I, Ryder NS, Ghannoum MA. Clinical Trichophyton rubrum strain exhibiting primary resistance to terbinafine. Antimicrob Agents Chemother. 2003;47(1):82-6. [PubMed ID: 12499173].
-
12.
Mendes AI, Silva AC, Catita JA, Cerqueira F, Gabriel C, Lopes CM. Miconazole-loaded nanostructured lipid carriers (NLC) for local delivery to the oral mucosa: improving antifungal activity. Colloids Surf B Biointerfaces. 2013;111:755-63. [PubMed ID: 23954816]. https://doi.org/10.1016/j.colsurfb.2013.05.041.
-
13.
Gupta M, Vyas SP. Development, characterization and in vivo assessment of effective lipidic nanoparticles for dermal delivery of fluconazole against cutaneous candidiasis. Chem Phys Lipids. 2012;165(4):454-61. [PubMed ID: 22309657]. https://doi.org/10.1016/j.chemphyslip.2012.01.006.
-
14.
Azanza Perea JR, Barberan J. Anfotericina B forma liposómica: un perfil farmacocinético exclusivo. Una historia inacabada. Revista Espanola de Quimioterapia. 2012;25(1).
-
15.
Mozafari MR. Nanoliposomes: preparation and analysis. Liposomes. Springer; 2010. p. 29-50.
-
16.
John HR. Reference method for broth dilution antifungal susceptibility testing of filamentous fungi, approved standard. M38-A2. Clin Lab Stand Inst. 2008;16:1-35.
-
17.
White TJ, Thomas Bruns SJ, Lee WT, Taylor JL. Amplification and direct sequencing of fungal ribosomal RNA genes for phylogenetics." PCR protocols: a guide to methods and applications. 1990. Available from: http://msafungi.org/wp-content/uploads/Inoculum/64(1).pdf.
-
18.
Panyam J, Labhasetwar V. Biodegradable nanoparticles for drug and gene delivery to cells and tissue. Adv Drug Deliv Rev. 2003;55(3):329-47. [PubMed ID: 12628320].
-
19.
Tang X, Zhu H, Sun L, Hou W, Cai S, Zhang R, et al. Enhanced antifungal effects of amphotericin B-TPGS-b-(PCL-ran-PGA) nanoparticles in vitro and in vivo. Int J Nanomedicine. 2014;9:5403-13. [PubMed ID: 25473279]. https://doi.org/10.2147/IJN.S71623.
-
20.
Osborne CS, Leitner I, Hofbauer B, Fielding CA, Favre B, Ryder NS. Biological, biochemical, and molecular characterization of a new clinical Trichophyton rubrum isolate resistant to terbinafine. Antimicrob Agents Chemother. 2006;50(6):2234-6. [PubMed ID: 16723593]. https://doi.org/10.1128/AAC.01600-05.
-
21.
Osborne CS, Leitner I, Favre B, Ryder NS. Amino acid substitution in Trichophyton rubrum squalene epoxidase associated with resistance to terbinafine. Antimicrob Agents Chemother. 2005;49(7):2840-4. [PubMed ID: 15980358]. https://doi.org/10.1128/AAC.49.7.2840-2844.2005.
-
22.
Paiao FG, Segato F, Cursino-Santos JR, Peres NT, Martinez-Rossi NM. Analysis of Trichophyton rubrum gene expression in response to cytotoxic drugs. FEMS Microbiol Lett. 2007;271(2):180-6. [PubMed ID: 17425668]. https://doi.org/10.1111/j.1574-6968.2007.00710.x.
-
23.
Sharma S, Sharma U. Synthesis, characterization and determination of encapsulation efficiency of chitosan nanoparticles for terbinafine. Indo Am J Pharm Res. 2013;3(12):1564-7.
-
24.
Tayel SA, El-Nabarawi MA, Tadros MI, Abd-Elsalam WH. Positively charged polymeric nanoparticle reservoirs of terbinafine hydrochloride: preclinical implications for controlled drug delivery in the aqueous humor of rabbits. AAPS PharmSciTech. 2013;14(2):782-93. [PubMed ID: 23615773]. https://doi.org/10.1208/s12249-013-9964-y.