Abstract
Context:
Neisseria meningitidis is the causative agent of a life-threatening infection with high mortality and morbidity worldwide. The most common types of this bacterium are serogroups A, B, C, W135, X, and Y. Although in some countries, such as Iran, the meningococcal meningitis has been well monitored and controlled by the use of divalent and quadrivalent vaccines, other fatal infections caused by these bacteria are still an important threat. For the above reason, this review focused on the differences of Neisseria characteristics, particularly in capsular composition, pathogenic and commensal stages to a better understanding of how to manage Neisseria infections.Evidence Acquisition:
In this review, PubMed, EMBASE, ScienceDirect, Scopus, and Google Scholar were searched for English-language publications on pathogenic or commensal strains of Neisseria, meningococcal disease, Neisseria biology, genetic diversity, molecular typing, serogroups, diagnostic, and epidemiology around the world up to July 2019. All articles and academic reports in the defined area of this research were considered too. The data were extracted and descriptively discussed.Results:
We included 85 studies in the survey. The data analysis revealed that the distribution of meningococcal serogroups was different regionally. For example, the serogroups C and W-135 accounted for Africa and Latin America regions, serogroup B in the European countries, and rarely in the Western Pacific, and serogroups A and C were dominant in Asian countries. Although data set for laboratory-based diagnosis of N. meningitidis are available for all countries, only 30% of the countries rely on reference laboratories for serogroup determination, and more than half of the countries lack the ability of surveillance system. Nevertheless, molecular detection procedure is also available for all countries. The use of the meningococcal vaccine is a variable country by country, but most countries have applied the meningococcal vaccine, either divalent or quadrivalent, for the protection of high-risk groups.Conclusions:
Owing to the geographical distribution of N. meningitidis serogroups in circulating, each country has to monitor for changes in serogroups diversity and its control management. Furthermore, laboratories should scale up the epidemiology and disease burden. It should be mentioned that quadrivalent meningococcal vaccines reduce the meningococcal disease burden sharply.Keywords
1. Context
One of the most important agents of the human’s oral microbiome is Neisseria spp., which may cause infectious diseases globally (1). Although immunization programs have been introduced worldwide, in order to maintain surveillance and a better understanding of the changing nature of disease pertaining to Neisseria population would be crucial for identifying disease control (2). In addition, extensive efforts have been made to design an effective vaccine against Neisseria disease, and several trials have been reported regarding this issue (3), but their results have revealed new challenges of which inadequate epidemiologic information, especially in developing countries. Commensal Neisseria strains colonized in the nasopharynx, species diversity, and circulating serogroups in some areas highly necessitate enhancing surveillance systems for Neisseria differentiation with more efficiency and precision (4). However, a few studies have mentioned the role of commensal Neisseria strains in some diseases.
The two most important pathogenic Neisseria species are the Neisseria gonorrhoea and N. meningitidis. Meanwhile, many Neisseria species are commensal. Neisseria is the resident of the human’s nasopharynx (5). The commensal Neisseria, including N. mucosa, N. cinerea, N. lactamica, N. polysaccharea, N. subflava, N. sicca, N. mucosa, N. flavescens, and N. elongata are not well-known yet. It may be due to the lack of accessible research detailed as well as the unpublished information. Plenty of research has been reported on the isolation and determination of Neisseria serotypes (6, 7). This review aimed to determine the characteristics of the Neisseria microbiome both in the healthy and disease condition.
2. Characteristics of Neisseria genus
Neisseria members are Gram-negative diplococci, which reside in the mucous membranes of man and animals (8). All Neisseria species are aerobic, chemoorganotrophic, and positive oxidase and catalase, as well as have the potential for carbohydrate fermentation. Some strains of N. elongate are catalase-negative (9). The oxidation-fermentation of carbohydrates, which is the main mechanism for utilization sugars by Neisseria species, is the hallmark diagnostic feature (Table 1). Some Neisseria species can utilize carbohydrates such as glucose, maltose, lactose, sucrose, and fructose (10). Other differential characteristics are shown in Table 1. The cultivation, isolation, and differentiation of colonized species in the nasopharynx of patients and healthy carriers are of great importance. Neisseria’s fimbriae (pili) and other surface antigens are associated with attachment, twitching motility, and genetic competence of the bacterium (11). Pathogenic Neisseria strains nutritionally are fastidious and grow at 35 - 37°C. Some species are capsule producers, and others produce a greenish-yellow pigment (12). Although the role of the commensal Neisseria species in the nasopharynx is not well understood yet, opportunistic members of the Neisseria genus were characterized (13).
Differential Characteristics of Human Neisseria Species
Species | Growth on Different Media and Temperature | Acid Production from Carbohydrates | Reduction of | Catalase | Oxidase | DNase | Pigment Production | |||||||
---|---|---|---|---|---|---|---|---|---|---|---|---|---|---|
A, 35°C | B, 22°C | C, 35°C | glu | malt | lac | suc | fru | NO3- | NO2- | |||||
Neisseria gonorrhoea | + | ± | - | + | - | - | - | - | - | - | + | + | - | - |
N. meningitidis | + | - | ± | + | + | - | - | - | - | - | + | + | - | - |
N. lactamica | + | ± | + | + | + | + | - | - | - | ± | + | + | - | - |
N. cinerea | ± | - | + | - | - | - | - | - | - | + | + | + | - | - |
N. polysaccharea | ± | + | + | + | + | - | - | - | - | + | + | + | - | - |
N. subflava | ± | + | + | + | + | - | ± | ± | - | + | + | + | - | + |
N. sicca | - | + | + | + | + | - | + | + | - | - | + | + | - | ± |
N. mucosa | - | + | + | + | + | - | + | + | + | + | + | + | - | + |
N. flavescens | - | + | + | - | - | - | - | - | - | - | + | + | - | + |
N. elongata | - | + | + | ± | - | - | - | - | ± | + | ± | + | - | + |
3. Growth Inquiry
All Neisseria species grow well in the atmosphere containing 5% - 10% carbon dioxide and the incubation temperature of 35 - 37°C and pH of 7 - 7.5; however, pathogenic Neisseria species do not grow at 22°C. In addition, Neisseria species may have complex growth requirements such as five amino acids and two vitamins; however, some require glucose and lactate, or both glucose and lactate for optimal growth (14). Some species may produce large amounts of ammonia during their growth in selective media, which will interfere with the detection of acid production from sugars such as glucose (15). For many years, researchers have stated that the Neisseria strains can grow on media containing mineral salts, lactate, and amino acids (16). In some cases, cysteine is required by less than 10% of the strains (17). For example, the N. gonorrhoea as a fastidious organism could not grow in the absence of glucose, pyruvate, or lactate (18). Furthermore, essential factors such as cysteine, glutamine, and carboxylase are required for primary isolation of gonococcal strains (19). Growth factor requirements for differentiating gonococcal strains are a useful tool for epidemiological studies (20). So design new protocols may be helpful for the identification of new diversities in this bacterium.
4. Laboratory Diagnosis
4.1. Conventional Identification
Over the past fifty years, the diagnostic methods of Neisseria have developed. The phenotypic and biochemical observation (Gram staining of urethral specimens is accurate only for symptomatic males), oxidase and catalase test, as well as carbohydrate utilization, will be decisive. In recent years, the gonococcal nucleic acid amplification tests, which have their own sensitivity and specificity compared with the value of bacterial cultures, are inevitable in the diagnosis of N. gonorrhoea as the gold standard tests (21). However, more than 25% of infected patients with N. gonorrhoea strains have missed in current culture-dependent surveillance. It may be due to non-cultivable forms of bacteria, which help the dissemination of multidrug-resistant N. gonorrhoea strains. However, the genomes of Neisseria species have been completely sequenced (22), and several determinants of biochemical reaction and enzymatic properties have been suggested for detection, prevention, and vaccine candidates (23). High homology has been reported between genes that are encoded by N. gonorrhoea and N. meningitides in the past several decades. For example, mechanisms of iron acquisition and chromosomal regions shared by N. meningitidis and N. gonorrhoea involved in the host interaction of both pathogens are expressing in more than 90% of genes (24).
4.2. Molecular Diagnosis
A few targeted genes have been proposed for the detection of Neisseria pathogenic species (25). Nucleic acid hybridization assay based on the pilin gene of N. gonorrhoea has reported, and the results of different studies revealed that the probes APTIMA Combo 2 and APTIMA for N. gonorrhoea had shown 100% specificity and 100% sensitivity for gonococcal and nongonococcal Neisseria species, respectively (26). However, molecular studies, based on 16SrRNA sequence analysis and DNA hybridization technique, have revealed similarities between some species of Eikenella and Simonsiella genera with Neisseria genus and vice versa (27). Another protocol for Branhamella catarrhalis (formerly N. catarrhalis) and Moraxella genus have been assigned well (28). Despite these, 16SrRNA sequence homology analysis has been used for the diagnosis of Neisseria species. The great problem for this approach is the method that cannot be used for monitoring antibiotic resistance and minimum inhibitory concentration (MIC) determinant of the identified pathogens.
4.3. Bacteriological Assay
Some essential precautions have to be considered. Collecting and processing the specimens are crucial, e.g., N. gonorrhoea can be readily isolated from genital specimens. As recommended in Table 1, all genital, rectal, and pharyngeal specimens must be inoculated on both selective and non-selective media because of interferences with commensal Neisseria species, which normally reside in mucosal surfaces. A non-selective medium should be used because some gonococcal strains are inhibited by the different antibacterial agents such as vancomycin present in selective media. The organisms may be inhibited by the fatty acids and trace elements present in the peptone hydrolysates and agar in laboratory media (29). Thus, it is necessary to use Muller Hinton agar as a base medium and then add growth enrichment materials to cultivate and isolate the gonococcus.
The results of a research suggested that gonococcus is more sensitive than the meningococcal bacterium. Therefore, gonococci could die rapidly if the specimens are allowed to dry; thus, dry and cold temperatures should be avoided by directly inoculating the specimen on to pre-warmed media at the time of sample collection. Furthermore, failure to perform the isolation and antibiotic susceptibility testing of gonococcal species may lead to the development of antibiotic resistance and consequently, children and even adults’ eye infection and blindness (30).
5. Pathogenicity and Spectrum of Diseases
During the last two centuries, N. meningitidis, the causative of meningitis and meningococcemia with rapid death and consequent disabilities, has feared human populations (31). However, this bacterium has been recognized as a commensal organism found in human’s mucus membrane surfaces. Therefore, the pathogenesis study of human commensal Neisseria represents important data, especially in meningococcal carriage (12). In recent years, researches have revealed that the spectrum of diseases caused by N. meningitidis has been raised sharply. Thus, pieces of evidence for meningococcal myogenic squamous ranges have increased. Because N. meningitidis is involved in the invasive pyomyositis (PM) (32), meningococcal sepsis, and septic arthritis in HIV-positive patients. In addition, septic shock, pneumonia, and fulminant purpura have been reported in adults with complement deficiency (33).
On the other hand, gonococci attach to mucous membrane and penetrate and multiply into the cells, then pass through the subepithelial cells, where the infection is established (34). Pili, PorB, and Opa proteins mediate the attachment and penetration into the host cells (35). The gonococcal Lipooligosaccharides (LOS) stimulates the release of the pro-inflammatory cytokines, i.e., tumor necrosis factor (TNF- α), which causes most of the symptoms associated with gonococcal diseases (36). The IgG3 is the predominant antibody formed in response to gonococcal infection, while the antibody to PorB and serum antibodies to pilin, Opa protein, and LOS were reported at a minimal level. Furthermore, antibodies to LOS can activate complement, thereby releasing complement component C5a, which has a chemotactic effect on neutrophils. However, IgG and secretory IgA1 antibodies directed against Rmp protein can block this bactericidal antibody response (37).
6. Neisseria meningitidis: Pathogenesis and Immunity
Cellular microbiology, genomics, and immunological studies have shed light on the pathogenic mechanisms and new prophylactic interventions (38). Recently, researchers have analyzed a gene library consisting of 2,850 insertional mutants of N. meningitidis in a rat model to show the essential step in the pathogenesis of N. meningitidis. They constructed and modified Neisseria genomic DNA by components of Tn10 transposon and revealed that out of 73 genes in the N. meningitidis genome only eight insertion components are essential for bacterial pathogenesis (39). However, these findings provide new insights for discussion of the meningococcal infection’s mechanisms. It has been now clarified that N. meningitidis has evolved a number of surface structural determinants (Figure 1), which mediate host cells’ interactions, as well as inhibiting the immune system and complement-mediated killing of bacteria (40, 41).
Antigens on the outer membrane of Neisseria meningitidis are shown. In this figure, the outer membrane proteins of N. meningitides, which interact with complement components, have illustrated. LOS (lipooligosaccharide); NalP (Neisseria autotransporter serine protease); porA (porin A); fhbp (factor heparin-binding protein); NspA (Neisseria surface protein A); porB2 (porin B); NHBA (Neisserial heparin-binding antigen) (41).
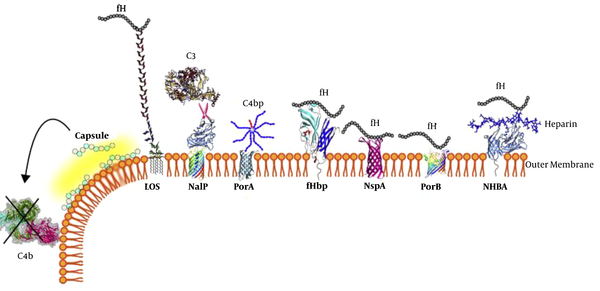
In addition to the above surface antigens, a lot of other outer membrane antigenic determinants exist, which were characterized during the molecular epidemiology and functional genomics studies by “omics” technologies in carriages and invasive isolated strains. However, it is important to implement laboratory animal models (in vivo) and in vitro studies for the study of mucosal and systemic interactions (42). Nasopharyngeal tissue organ studies have shown that the role of a meningococcal factors interferes with epithelial cell attachment. It resulted in the enhanced ability of bacterial adhesion to epithelial cells and release into the airways; thus, it may the person-to-person spread of the disease potentially has increased (43). Meningococcal disease occurs in patients who lack specific antibodies directed against the polysaccharide capsule and other bacterial antigens. In addition, the central nervous system (CNS) is very susceptible to microorganisms such as N. meningitidis, which can activate polymorphonuclear leukocytes (PMCs) and is transferred to the subarachnoid space; subsequently, initiates an inflammatory response and spreads to the brain tissue (44).
Based on available data, the immune responses can be stimulated by the colonization of N. meningitidis. The antigenic components of none capsulated Neisseria species and Escherichia coli K1 can cross-react with the group B capsular polysaccharide. The analysis of cross-reaction of different Gram-negative bacteria, including Neisseria, indicated that some common proteins with a range of 60 - 75 KDa and proteins p100, p41, OspA, as well as pC, have cross-reacted with immune sera against those bacteria (45). One of the outer membrane proteins is FeRP-70, which is strongly immunogenic and cross-react with different strains of bacteria (46).
These data demonstrated new, promising approaches to design and develop a wide-range vaccine; however, further investigation is needed to confirm these data. However, it is important to know that each N. meningitidis serogroup contains several serotypes. The hallmark characteristic of N. meningitidis serogroup is antigenic polysaccharide capsules. While the serotypes of other serogroups are characterized by antigenic properties of the outer surface membrane proteins. However, there are several reports on the cross-reactivity between N. meningitidis and N. lactamica (47). The relationship between serotypes and clonality of them remained unknown. For example, LOS of the eight immunotypes found in the serogroup B of N. meningitidis, have similar cross-reactivity with N. meningitidis M986 (immunotype 7 epitope), a strain used for the production of a serotype 2a vaccine (48), which has no protective effects on the disease caused by serogroup B. In this regard, further advanced molecular studies are needed.
7. Neisseria Adhesion as Attachment Tools
A few Neisseria adhesion molecules have a crucial role in the survival of the bacterium in the blood. For example, the Opa and PorB as mannose-binding lectin (MBL) have caused a decrease in fixing the complement system and blocking the bacterial killing (49). Models of human dermal microvessel studies showed that the Pili, Opa, and Opc proteins are the most important adhesion molecules for the pathogenesis of Neisseria (50). A few Neisseria adhesion molecules and their host cell’s receptors are illustrated in Figure 2. Glycemic research results have suggested that N. meningitidis strains express a range of surface glycosylate components, including polysaccharide, lipooligosaccharide, and O-linked glycoproteins, While N. gonorrhoea does not have the same antigens. In addition, the N. gonorrhoea, as a scavenger of host sialic acids, play a key role in the serum resistance and survival in the host anyway (51).
A; The Neisseria meningitidis surface antigens and their interaction with host epithelial cells are shown. Based on available data, these structures are absent in gonococci, whereas OmpA is specific for this organism. B; shows Neisseria Opa and Opc proteins involved in binding of the ligands. It must be mentioned that Opa proteins are expressed by meningococci and gonococci equally, while Opc is only expressed by meningococcal strains (52)
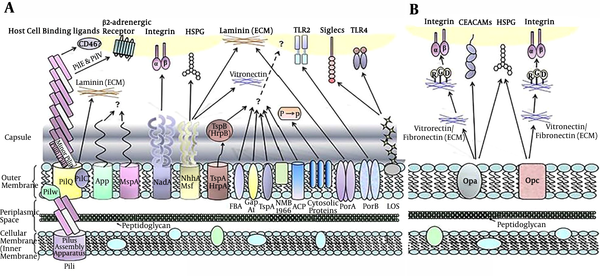
A study revealed that the complexity of outer membrane heterodimer leads to the provocation of signaling pathways of actin polymerization and accumulation of ezrin, which are crucial for N. meningitidis colonizing in blood vessels as well as G protein receptor activation (53). Another study revealed human glycan as an important target (Figure 2) for interactions with bacteria such as N. meningitidis, which binds to the host and may occur meningococcal infection and colonization (54). Thus, the meningococcal surface proteins and/or glycans that are responsible for all interactions can potentially be targeted for the development of novel antibiotics or vaccines to prevent meningococcal diseases.
Furthermore, the bacterial outer membrane antigens as auto transporter and secretion systems have critical roles in host-pathogen interactions, evasion from immunity, activation of iron-binding proteins of the host’s cell, prevention of complement activation, neutralization of antimicrobial peptides, degradation of immunoglobulins, permeabilization of epithelial layers, the formation of biofilms and even the suppression of the same ecological niche (55). Numerous encoding genes of Neisseria surface antigens have been identified (Figure 2). For example, in the pathogenic Neisseria strains, more than 20 genes are involved in the biology and function of the Type IV pilus (Tfp, RpoD) and the integration host factor (IHF) proteins (52).
8. Capsular Polysaccharides and Serotyping
The N. meningitidis strains can produce polysaccharide capsules and exopolysaccharides (Table 2) with immunogenic properties and as a tool associated with pathogenicity, virulence, and typing of the bacterial strains, while N. gonorrhoea strains cannot produce immunogenic polysaccharide capsules. However, the capsule production studies suggested that N. gonorrhoea strains may express unusual capsule layer, such as polyphosphate, which may be involved in N. gonorrhoea infection but their prevention has not been demonstrated yet (56).
The Basic Structural and Major Chemical Compositions of Neisseria meningitidis and other Neisseria Species Polysaccharide Capsule
Neisseria Species | Serogroups | Basic Structural Units and Their Ligands | Acetylation | Ref |
---|---|---|---|---|
Neisseria meningitidis | Serogroup A | ManNac-(1-P→6)-3-OAc | + | (57) |
Serogroup B | NeuNAc-(2→8) | - | (58) | |
Serogroup C | NeuNAc-(2→9), 7 OAc and 8-OAc | + | ||
Serogroup H | → 4)α-D-Gal-(1→2)-Gro-(3-P→ | - | (59) | |
Serogroup I | → 4)α-L-GulNAcA(1-3)β-D-ManNAcA(1→40 OAc | + | ||
Serogroup K | →3)-β-D- ManNAcA-(1→4)-β-D-ManNAcA-(1→ and 4OAc | - | ||
Serogroup L | →3)-β-D-GlcNAc-(1→3)β-D-GlcNAc-(1→3)α-D-GlcNAc-(1-P- | - | (60) | |
Serogroup W- 135 | 6-D-Glc(1→4)-NeuNAc(2→6) | + | (61) | |
Serogroup X | GlcNAc(1-P-4)- | - | ||
Serogroup Y | D-Glc(1→4)-NeurNAc(3’-p→4) and OAc | + | ||
Serogroup Z | D-GalNAc(1→)-Gro-(3’-p→4) | - | ||
Serogroup E- 29 | D- GalNnc(1→7)-KDO (2→3)-4 and 5-OAc | + | (62) | |
N. gonorrhoea | Not detected yet | Not detected yet | ND | ND |
N. lactamica | Not detected yet | Antigenically similar to capsular polysaccharide of N. meningitidis serogroup B | ND | ND |
N. polysaccharea | Not detected yet | Glycogen by branches 1,4-linked to α- D- glucopyranosyl residues; and about 6% 4,6-di-O-substituted α -D-glucopyranosyl branch point | ND | ND |
N. Sicca | Not detected yet | Polymer of GalNA | ND | ND |
N. subflava | Not detected yet | Similar to N. polysaccharea | ND | ND |
Instead, N. meningitidis expresses and produces polysaccharide capsules, which have shown a major antigenic differences, and have provided an essential tool for serogrouping of N. meningitidis strains. There are 13 serogroups of N. meningitidis, which alphabetically are named as follows: A, B, C, D, E- 29, H, I, K, L, W-135, X, Z, and Y., six serogroups out of which mostly cause fatal diseases; these serogroups are A, B, C, W-135, and Y (63). The essential characterizations of N. meningitidis serogroups’ exopolysaccharides are presented in Table 2; however, the crucial point for meningococcal meningitides disease by serogroups A, B, C, W-135, X, Y is the reliance on the overexpression of exopolysaccharides and switching to the acetylation by which evades from the immune system and enhances the pathogenicity (64).
An important question is, why only 6 out of 13 N. meningitidis serogroups can produce immunogenic and protective capsules. Although the answer to the question is not clear; likely, the addition of the residual acetyl groups to the capsular polysaccharide could increase immunogenic properties. While other serogroups and Neisseria commensal strains lack this ability as well as different capsule compositions (Table 2). So, because the acetylated component is more common in different strains, it results in cross-reactivity and enhances protective properties (65). The results of researches have indicated that biochemistry and genetics of group B polysaccharide capsules are also different.
Based on bacterial genome sequences, 24 invasive strains of N. meningitidis serogroup C from Brazil were mapped, and enzyme-encoding gene studies for the synthesis of meningococcal polysaccharide capsule showed that these genes are located on pathogenicity islands and organized as an operon (66). Furthermore, the whole genome sequencing (WGS) method leads to advances in the detection of isolated N. meningitidis strains (67).
Other research results suggested that the capsular manufacturing unit of N. meningitidis may relatively be similar to nonpathogenic Neisseria species, including N. polysacchareae, N. canis, N. cinerea, and N. subflava. They all have possessed the enzymes that can synthesize extracellular polysaccharides, which are glycogen-like when growing on a medium containing 1-5% sucrose (68). The components and types of capsule constituent of the different Neisseria strain are shown in Table 2.
9. State of Neisseria Carriage
Genomic study of Neisseria strains showed relatively a type II-C CRISPR/Cas system similarity and, thus, invasiveness lineages in comparison to knock-out strains, which lack the Cas9 protein (69). Although the commensal species have not the ability to damage the host, recent researches indicated that the pathogenic serogroups are different from the commensal species, which may be dependent on the site of colonization and adaptive immunity (70). This is established for healthy people. Likewise, the commensal Neisseria strains can cause meningeal infection in people with immune deficiencies, especially in complement disorders and or with low levels of personal hygiene.
To date, it is not fully understood why the use of new biological drugs provokes the pathogenesis of some commensal Neisseria strains. Up to now, seven cases of disease from commensal Neisseria species in Eculizumab recipients have reported (71). However, microbiome studies have shown that the people carried N. lactamica, and may reduce the meningococcal carriages. Recently, a protocol detailing a controlled human infection experiment was published that uses genetically modified N. lactamica. The commensal bacterium was modified to produce the meningococcal outer membrane adhesin NadA, which the researchers hypothesis will act to immunize participants against the heterologous antigen. Importantly, they describe that genetic modification of the bacterium does not influence the pathogenicity of the organism in any way, and their strains remain completely non-pathogenic (72). In recent years, the encoded capsular genes were identified in non-pathogenic Neisseria species, including N. subflava and N. elongate. These species were homologous to those of N. meningitidis, as well as the novel synthetic capsule exopolysaccharides (73).
10. Molecular Typing
In recent years, the WGS method was reported for molecular surveillance of bacteria. This new method for the identification of gene variations has been considered (74). The WGS has performed by using the Illumina MiSeq platform, and data were processed by the Velvet software so that detailed characteristics of N. meningitidis isolates are provided for researchers. However, they believed that high-quality WGS data made it possible to identify gene diversity that could not be recognized by conventional sequencing methods (75). The most accurate information on the genetic diversity of isolates was obtained by the comparison of WGS data with conventional sequencing data. Thus, the WGS method showed higher potential for accurately determining the genetic characteristics of N. meningitidis that may be desirable as a routine molecular method to detect invasive meningococcal disease (76). In addition, the genome assembly of an invasive N. meningitidis (IMD) isolate revealed the usefulness of this method. In fact, WGS can also be used for not only the diagnosis but also epidemiological and antibiotic resistance surveillance of IMD (77). Therefore, this method seems to be applied to microbiological studies for the future and may be replaced with other existing methods. It should be mentioned that a few countries had made more scientific databases than ever before and asserted their sovereignty around the world.
11. Epidemiology
There is no comprehensive epidemiological study covering worldwide of pathogenic Neisseria species. While regional epidemiological information is abundant. For example, the data of outbreaks was stratified by geographical region by the World Health Organization (WHO). The major outbreaks identified were in the United State (41/83) and then in the European region (30/83). While in the Western Pacific, Eastern Mediterranean, and South-East Asian regions, there were < 10 outbreaks reported. The predominant serogroups in the majority of outbreaks were serogroup C (61%), followed by serogroup B (29%), serogroup A (5%), and serogroup W-135 (4%) (78). Currently, the epidemiological studies of meningococcal meningitis in the Islamic Republic of Iran over the past 10 years indicate that the disease subsides over the age of 15 years and is under control (79). However, N. meningitidis is the leading global cause of meningitis and rapidly fatal sepsis.
A recent report indicates that serogroups B and C are the predominant serogroups among meningococci causing invasive diseases in Italy from 2013 to 2016. Moreover, an increase in serogroup W-135 of N. meningitidis (MenW) was observed, which showed co-circulation of the Hajj pilgrims and the South American sublineages, belonging to MenW/clonal complex and might gradually surpass in Italy (80). However, continued surveillance supported by genomic characterization and pathogen dissemination and the detection of epidemic-associated strains would be crucial for control of the meningococcal diseases.
12. Other Meningeal Diseases
Recently, a significant increase in meningococcal urethritis primarily among active heterosexual men has reported in the United States. The causative clone of the bacterium was a unique none-encapsulated of N. meningitidis, which belonged to the cc11/ET-15 hyperinvasive lineage (81). The new emergence of meningococcal disease has been reported in both developed and developing countries; thus, the absence of a universal vaccine covering all pathogenic strain necessitates further investigations (82). However, antibiotic eradication of N. meningitidis in healthy carriers is ineffective. Thus, the prevention of disease has focused on the enhancement of immunity by the use of vaccines directed against the serogroups most commonly associated with disease (83).
Two quadrivalent vaccines (a polysaccharide vaccine and a polysaccharide-protein conjugate vaccine) were effective against A, C, Y, and W-135 serogroups. Which currently licensed in the United States (84). The latter is recommended for all adolescents aged 11 or 12 years, and a booster should be given at age 16. Other at-risk adults increased for meningococcal disease should be vaccinated with a divalent or quadrivalent vaccine. It is reported that the serogroup B polysaccharide is a weak immunogenic and is antigenically related to a polysaccharide in human neurologic tissues (85). Efforts are ongoing to develop the serogroup B protein vaccines. However, a new meningococcal A conjugate vaccine has been recently demonstrated in some Africa regions, and it planned that by 2016 all 26 African meningitis belt countries will be provided a new meningococcal A conjugate vaccine.
Acknowledgements
References
-
1.
Yamashita Y, Takeshita T. The oral microbiome and human health. J Oral Sci. 2017;59(2):201-6. [PubMed ID: 28637979]. https://doi.org/10.2334/josnusd.16-0856.
-
2.
Safadi MA, Bettinger JA, Maturana GM, Enwere G, Borrow R, Global Meningococcal I. Evolving meningococcal immunization strategies. Expert Rev Vaccines. 2015;14(4):505-17. [PubMed ID: 25494168]. https://doi.org/10.1586/14760584.2015.979799.
-
3.
Yaesoubi R, Trotter C, Colijn C, Yaesoubi M, Colombini A, Resch S, et al. The cost-effectiveness of alternative vaccination strategies for polyvalent meningococcal vaccines in Burkina Faso: A transmission dynamic modeling study. PLoS Med. 2018;15(1). e1002495. [PubMed ID: 29364884]. [PubMed Central ID: PMC5783340]. https://doi.org/10.1371/journal.pmed.1002495.
-
4.
Shaker R, Fayad D, Dbaibo G. Challenges and opportunities for meningococcal vaccination in the developing world. Hum Vaccin Immunother. 2018;14(5):1084-97. [PubMed ID: 29393729]. [PubMed Central ID: PMC5989893]. https://doi.org/10.1080/21645515.2018.1434463.
-
5.
Liu G, Tang CM, Exley RM. Non-pathogenic Neisseria: members of an abundant, multi-habitat, diverse genus. Microbiology. 2015;161(7):1297-312. [PubMed ID: 25814039]. https://doi.org/10.1099/mic.0.000086.
-
6.
Ataee RA, Mehrabi-Tavana A, Hosseini SM, Kaviani F. Carriage rates of Neisseria meningitidis serogroups: determination among freshmen conscripts before vaccination. Iran J Microbiol. 2016;8(3):203-9. [PubMed ID: 27928488]. [PubMed Central ID: PMC5139924].
-
7.
Ataee RA, Mehrabi A, Ghorbani GH, Mosavi SA, Karimi ZA, Hajia M. Recurrent meningococcal meningitis in an Iranian conscript: a brief report. Clinical Microbiology Newsletter. 2005;27(17):136-7. https://doi.org/10.1016/j.clinmicnews.2005.08.006.
-
8.
Smith NH, Holmes EC, Donovan GM, Carpenter GA, Spratt BG. Networks and groups within the genus Neisseria: analysis of argF, recA, rho, and 16S rRNA sequences from human Neisseria species. Mol Biol Evol. 1999;16(6):773-83. [PubMed ID: 10368955]. https://doi.org/10.1093/oxfordjournals.molbev.a026162.
-
9.
Hollis DG, Wiggins GL, Weaver RE. Neisseria lactamicus sp. n., a lactose-fermenting species resembling Neisseria meningitidis. Appl Microbiol. 1969;17(1):71-7. [PubMed ID: 4975454]. [PubMed Central ID: PMC377615].
-
10.
Knapp JS, Holmes KK. Modified oxidation-fermentation medium for detection of acid production from carbohydrates by Neisseria spp. and Branhamella catarrhalis. J Clin Microbiol. 1983;18(1):56-62. [PubMed ID: 6885992]. [PubMed Central ID: PMC270744].
-
11.
Mukhopadhyay TK, Halliwell D, O'Dwyer C, Shamlou PA, Levy MS, Allison N, et al. Rapid characterization of outer-membrane proteins in Neisseria lactamica by SELDI-TOF-MS (surface-enhanced laser desorption ionization-time-of-flight MS) for use in a meningococcal vaccine. Biotechnol Appl Biochem. 2005;41(Pt 2):175-82. [PubMed ID: 15315478]. https://doi.org/10.1042/BA20040098.
-
12.
Stephens DS. Biology and pathogenesis of the evolutionarily successful, obligate human bacterium Neisseria meningitidis. Vaccine. 2009;27 Suppl 2:B71-7. [PubMed ID: 19477055]. [PubMed Central ID: PMC2712446]. https://doi.org/10.1016/j.vaccine.2009.04.070.
-
13.
Denis F, Brisou J, Hoppeler A, Fizazi T, Creusot G. [A commensal neisseria (Neisseria perflava) responsible for a case of meningitis]. Presse Med. 1970;78(51):2284. [PubMed ID: 5496186].
-
14.
Johnson AP. The pathogenic potential of commensal species of Neisseria. J Clin Pathol. 1983;36(2):213-23. [PubMed ID: 6338050]. [PubMed Central ID: PMC498155]. https://doi.org/10.1136/jcp.36.2.213.
-
15.
Knapp JS. Historical perspectives and identification of Neisseria and related species. Clin Microbiol Rev. 1988;1(4):415-31. [PubMed ID: 3069201]. [PubMed Central ID: PMC358063]. https://doi.org/10.1128/cmr.1.4.415.
-
16.
Sakai K, Yamauchi T, Nakasu F, Ohe T. Biodegradation of cellulose acetate by Neisseria sicca. Biosci Biotechnol Biochem. 1996;60(10):1617-22. [PubMed ID: 8987659]. https://doi.org/10.1271/bbb.60.1617.
-
17.
McDonald IJ, Johnson KG. Nutritional requirements of some non-pathogenic Neisseria grown in simple synthetic media. Can J Microbiol. 1975;21(8):1198-204. [PubMed ID: 1164696]. https://doi.org/10.1139/m75-179.
-
18.
Keevil CW, Major NC, Davies DB, Robinson A. Physiology and virulence determinants of Neisseria gonorrhoeae grown in glucose-, oxygen- or cystine-limited continuous culture. J Gen Microbiol. 1986;132(12):3289-302. [PubMed ID: 3116154]. https://doi.org/10.1099/00221287-132-12-3289.
-
19.
Dewhirst FE, Chen CK, Paster BJ, Zambon JJ. Phylogeny of species in the family Neisseriaceae isolated from human dental plaque and description of Kingella oralis sp. nov [corrected]. Int J Syst Bacteriol. 1993;43(3):490-9. [PubMed ID: 8347509]. https://doi.org/10.1099/00207713-43-3-490.
-
20.
Yates E, Gao L, Woodcock N, Parsons N, Cole J, Smith H. In a medium containing glucose, lactate carbon is incorporated by gonococci predominantly into fatty acids and glucose carbon incorporation is increased: implications regarding lactate stimulation of metabolism. Int J Med Microbiol. 2000;290(7):627-39. [PubMed ID: 11200544]. https://doi.org/10.1016/S1438-4221(00)80012-0.
-
21.
Morse SA, Bartenstein L. Purine metabolism in Neisseria gonorrhoeae: the requirement for hypoxanthine. Can J Microbiol. 1980;26(1):13-20. [PubMed ID: 6773641]. https://doi.org/10.1139/m80-003.
-
22.
Barlow D. Culture of the gonococcus: a reliable gold standard? Sex Transm Infect. 2017;93(3):178. [PubMed ID: 28196837]. https://doi.org/10.1136/sextrans-2016-053004.
-
23.
van der Veer BM, Wolffs PF, Hoebe CJ, Dukers-Muijrers NH, van Alphen LB. Culture-free genotyping of Neisseria gonorrhoeae revealed distinct strains at different anatomical sites in a quarter of patients, the Netherlands, 2012 to 2016. Euro Surveill. 2018;23(50). [PubMed ID: 30563596]. [PubMed Central ID: PMC6299510]. https://doi.org/10.2807/1560-7917.ES.2018.23.50.1800253.
-
24.
Zhu B, Yao P, Zhang L, Gao Y, Xu L, Xie N, et al. Genetic Analysis of Neisseria meningitidis Sequence Type 7 Serogroup X Originating from Serogroup A. Infect Immun. 2017;85(6). [PubMed ID: 28320835]. [PubMed Central ID: PMC5442631]. https://doi.org/10.1128/IAI.01019-16.
-
25.
McCarthy PC, Saksena R, Peterson DC, Lee CH, An Y, Cipollo JF, et al. Chemoenzymatic synthesis of immunogenic meningococcal group C polysialic acid-tetanus Hc fragment glycoconjugates. Glycoconj J. 2013;30(9):857-70. [PubMed ID: 23949787]. https://doi.org/10.1007/s10719-013-9490-x.
-
26.
Rohde KH, Dyer DW. Mechanisms of iron acquisition by the human pathogens Neisseria meningitidis and Neisseria gonorrhoeae. Front Biosci. 2003;8:d1186-218. [PubMed ID: 12957813]. https://doi.org/10.2741/1133.
-
27.
Ataee RA, Ghorban Alizadegan M, Hajia M, Gudarzi Z, Mherabi Tavana A, Nakhjavani FA. Rapid diagnosis of neisseria meningitides by pcr method. Cell Journal (Yakhteh). 2006;8(2 (30)).
-
28.
Golparian D, Tabrizi SN, Unemo M. Analytical specificity and sensitivity of the APTIMA Combo 2 and APTIMA GC assays for detection of commensal Neisseria species and Neisseria gonorrhoeae on the Gen-Probe Panther instrument. Sex Transm Dis. 2013;40(2):175-8. [PubMed ID: 23324980]. https://doi.org/10.1097/OLQ.0b013e3182787e45.
-
29.
Rossau R, Heyndrickx L, Van Heuverswyn H. Nucleotide sequence of a 16S ribosomal RNA gene from Neisseria gonorrhoeae. Nucleic Acids Res. 1988;16(13):6227. [PubMed ID: 3135537]. [PubMed Central ID: PMC336862]. https://doi.org/10.1093/nar/16.13.6227.
-
30.
Heiske A, Mutters R. Differentiation of selected members of the family Neisseriaceae (Alysiella, Eikenella, Kingella, Simonsiella and CDC groups EF-4 and M-5) by carbohydrate fingerprints and selected phenotypic features. Zentralblatt für Bakteriologie. 1994;281(1):67-79. https://doi.org/10.1016/s0934-8840(11)80639-0.
-
31.
Dolange V, Churchward CP, Christodoulides M, Snyder LAS. The Growing Threat of Gonococcal Blindness. Antibiotics (Basel). 2018;7(3). [PubMed ID: 30002340]. [PubMed Central ID: PMC6164567]. https://doi.org/10.3390/antibiotics7030059.
-
32.
Strelow VL, Vidal JE. Invasive meningococcal disease. Arq Neuropsiquiatr. 2013;71(9B):653-8. [PubMed ID: 24141498]. https://doi.org/10.1590/0004-282X20130144.
-
33.
Barakat MT, Gajurel K, Fischer K, Stevens K, Ozdalga E, Montoya JG. A Case of Meningococcal Pyomyositis in an Otherwise Healthy Adult. Open Forum Infect Dis. 2016;3(3):ofw087. [PubMed ID: 27703989]. [PubMed Central ID: PMC5047391]. https://doi.org/10.1093/ofid/ofw087.
-
34.
Audemard-Verger A, Descloux E, Ponard D, Deroux A, Fantin B, Fieschi C, et al. Infections Revealing Complement Deficiency in Adults: A French Nationwide Study Enrolling 41 Patients. Medicine (Baltimore). 2016;95(19). e3548. [PubMed ID: 27175654]. [PubMed Central ID: PMC4902496]. https://doi.org/10.1097/MD.0000000000003548.
-
35.
Lenz JD, Dillard JP. Pathogenesis of Neisseria gonorrhoeae and the Host Defense in Ascending Infections of Human Fallopian Tube. Front Immunol. 2018;9:2710. [PubMed ID: 30524442]. [PubMed Central ID: PMC6258741]. https://doi.org/10.3389/fimmu.2018.02710.
-
36.
Stevens JS, Criss AK. Pathogenesis of Neisseria gonorrhoeae in the female reproductive tract: neutrophilic host response, sustained infection, and clinical sequelae. Curr Opin Hematol. 2018;25(1):13-21. [PubMed ID: 29016383]. [PubMed Central ID: PMC5753798]. https://doi.org/10.1097/MOH.0000000000000394.
-
37.
Sanyal A, Shen C, Ding M, Reinhart TA, Chen Y, Sankapal S, et al. Neisseria gonorrhoeae uses cellular proteins CXCL10 and IL8 to enhance HIV-1 transmission across cervical mucosa. Am J Reprod Immunol. 2019;81(6). e13111. [PubMed ID: 30903720]. [PubMed Central ID: PMC6540971]. https://doi.org/10.1111/aji.13111.
-
38.
Hobbs MM, Sparling PF, Cohen MS, Shafer WM, Deal CD, Jerse AE. Experimental Gonococcal Infection in Male Volunteers: Cumulative Experience with Neisseria gonorrhoeae Strains FA1090 and MS11mkC. Front Microbiol. 2011;2:123. [PubMed ID: 21734909]. [PubMed Central ID: PMC3119411]. https://doi.org/10.3389/fmicb.2011.00123.
-
39.
Quillin SJ, Seifert HS. Neisseria gonorrhoeae host adaptation and pathogenesis. Nat Rev Microbiol. 2018;16(4):226-40. [PubMed ID: 29430011]. [PubMed Central ID: PMC6329377]. https://doi.org/10.1038/nrmicro.2017.169.
-
40.
Sun YH, Bakshi S, Chalmers R, Tang CM. Functional genomics of Neisseria meningitidis pathogenesis. Nat Med. 2000;6(11):1269-73. [PubMed ID: 11062540]. https://doi.org/10.1038/81380.
-
41.
Pizza M, Rappuoli R. Neisseria meningitidis: pathogenesis and immunity. Curr Opin Microbiol. 2015;23:68-72. [PubMed ID: 25461575]. https://doi.org/10.1016/j.mib.2014.11.006.
-
42.
Soriani M. Unraveling Neisseria meningitidis pathogenesis: from functional genomics to experimental models. F1000Res. 2017;6:1228. [PubMed ID: 28781769]. [PubMed Central ID: PMC5531161]. https://doi.org/10.12688/f1000research.11279.1.
-
43.
Laver JR, Hughes SE, Read RC. Neisserial Molecular Adaptations to the Nasopharyngeal Niche. Adv Microb Physiol. 2015;66:323-55. [PubMed ID: 26210107]. https://doi.org/10.1016/bs.ampbs.2015.05.001.
-
44.
Schubert-Unkmeir A. Molecular mechanisms involved in the interaction of Neisseria meningitidis with cells of the human blood-cerebrospinal fluid barrier. Pathog Dis. 2017;75(2). [PubMed ID: 28334198]. https://doi.org/10.1093/femspd/ftx023.
-
45.
Troncoso G, Sanchez S, Moreda M, Criado MT, Ferreiros CM. Antigenic cross-reactivity between outer membrane proteins of Neisseria meningitidis and commensal Neisseria species. FEMS Immunol Med Microbiol. 2000;27(2):103-9. [PubMed ID: 10640604]. https://doi.org/10.1111/j.1574-695X.2000.tb01419.x.
-
46.
Bruckbauer HR, Preac-Mursic V, Fuchs R, Wilske B. Cross-reactive proteins of Borrelia burgdorferi. Eur J Clin Microbiol Infect Dis. 1992;11(3):224-32. [PubMed ID: 1597198]. https://doi.org/10.1007/BF02098084.
-
47.
Ala'Aldeen DA, Wall RA, Borriello SP. Immunogenicity and cross-reactivity of the 70-Kda iron-regulated protein of Neisseria meningitidis in man and animals. J Med Microbiol. 1990;32(4):275-81. [PubMed ID: 2118187]. https://doi.org/10.1099/00222615-32-4-275.
-
48.
Sanchez S, Troncoso G, Criado MT, Ferreiros C. In vitro induction of memory-driven responses against Neisseria meningitidis by priming with Neisseria lactamica. Vaccine. 2002;20(23-24):2957-63. [PubMed ID: 12126908]. https://doi.org/10.1016/s0264-410x(02)00224-4.
-
49.
Tsai CM, Mocca LF, Frasch CE. Immunotype epitopes of Neisseria meningitidis lipooligosaccharide types 1 through 8. Infect Immun. 1987;55(7):1652-6. [PubMed ID: 2439458]. [PubMed Central ID: PMC260573].
-
50.
Estabrook MM, Jack DL, Klein NJ, Jarvis GA. Mannose-binding lectin binds to two major outer membrane proteins, opacity protein and porin, of Neisseria meningitidis. J Immunol. 2004;172(6):3784-92. [PubMed ID: 15004183]. https://doi.org/10.4049/jimmunol.172.6.3784.
-
51.
Melican K, Michea Veloso P, Martin T, Bruneval P, Dumenil G. Adhesion of Neisseria meningitidis to dermal vessels leads to local vascular damage and purpura in a humanized mouse model. PLoS Pathog. 2013;9(1). e1003139. [PubMed ID: 23359320]. [PubMed Central ID: PMC3554624]. https://doi.org/10.1371/journal.ppat.1003139.
-
52.
Hung MC, Christodoulides M. The biology of Neisseria adhesins. Biology (Basel). 2013;2(3):1054-109. [PubMed ID: 24833056]. [PubMed Central ID: PMC3960869]. https://doi.org/10.3390/biology2031054.
-
53.
Mubaiwa TD, Semchenko EA, Hartley-Tassell LE, Day CJ, Jennings MP, Seib KL. The sweet side of the pathogenic Neisseria: the role of glycan interactions in colonisation and disease. Pathog Dis. 2017;75(5). [PubMed ID: 28633281]. [PubMed Central ID: PMC5808653]. https://doi.org/10.1093/femspd/ftx063.
-
54.
Virion Z, Marullo S, Coureuil M. Methods to Study the Roles of beta-Arrestins in Meningococcal Signaling. Methods Mol Biol. 2019;1957:325-34. [PubMed ID: 30919363]. https://doi.org/10.1007/978-1-4939-9158-7_20.
-
55.
Seib KL, Jennings MP, Day CJ. Characterizing the meningococcal glycointeractome: what is new? Future Microbiol. 2018;13:279-82. [PubMed ID: 29441801]. https://doi.org/10.2217/fmb-2017-0222.
-
56.
Rendon MA, Lona B, Ma M, So M. RpoN and the Nps and Npa two-component regulatory system control pilE transcription in commensal Neisseria. Microbiologyopen. 2019;8(5). e00713. [PubMed ID: 30079633]. [PubMed Central ID: PMC6528607]. https://doi.org/10.1002/mbo3.713.
-
57.
MacKenzie CR, Perry MB, McDonald IJ, Johnson KG. Structure of the D-glucans produced by Neisseria perflava. Can J Microbiol. 1978;24(11):1419-22. [PubMed ID: 743649]. https://doi.org/10.1139/m78-227.
-
58.
Bundle DR, Smith IC, Jennings HJ. Determination of the structure and conformation of bacterial polysaccharides by carbon 13 nuclear magnetic resonance. Studies on the group-specific antigens of Neisseria meningitidis serogroups A and X. J Biol Chem. 1974;249(7):2275-81. [PubMed ID: 4206552].
-
59.
Dell A, Azadi P, Tiller P, Thomas-Oates J, Jennings HJ, Beurret M, et al. Analysis of oligosaccharide epitopes of meningococcal lipopolysaccharides by fast-atom-bombardment mass spectrometry. Carbohydr Res. 1990;200:59-76. [PubMed ID: 1696172]. https://doi.org/10.1016/0008-6215(90)84182-t.
-
60.
Gamian A, Beurret M, Michon F, Brisson JR, Jennings HJ. Structure of the L2 lipopolysaccharide core oligosaccharides of Neisseria meningitidis. J Biol Chem. 1992;267(2):922-5. [PubMed ID: 1730681].
-
61.
Bhattacharjee AK, Jennings HJ, Kenny CP. Structural elucidation of the 3-deoxy-D-manno-octulosonic acid containing meningococcal 29-e capsular polysaccharide antigen using carbon-13 nuclear magnetic resonance. Biochemistry. 1978;17(4):645-51. [PubMed ID: 414779]. https://doi.org/10.1021/bi00597a013.
-
62.
Ashton FE, Ryan A, Diena B, Jennings HJ. A new serogroup (L) of Neisseria meningitidis. J Clin Microbiol. 1983;17(5):722-7. [PubMed ID: 6408117]. [PubMed Central ID: PMC272732].
-
63.
Richardson WP, Sadoff JC. Production of a capsule of Neisseria gonorrhoeae. Infect Immun. 1977;15(2):663-4. [PubMed ID: 403142]. [PubMed Central ID: PMC421417].
-
64.
Takada S, Fujiwara S, Inoue T, Kataoka Y, Hadano Y, Matsumoto K, et al. Meningococcemia in Adults: A Review of the Literature. Intern Med. 2016;55(6):567-72. [PubMed ID: 26984070]. https://doi.org/10.2169/internalmedicine.55.3272.
-
65.
Tzeng YL, Thomas J, Stephens DS. Regulation of capsule in Neisseria meningitidis. Crit Rev Microbiol. 2016;42(5):759-72. [PubMed ID: 26089023]. [PubMed Central ID: PMC4893341]. https://doi.org/10.3109/1040841X.2015.1022507.
-
66.
Fiebig T, Freiberger F, Pinto V, Romano MR, Black A, Litschko C, et al. Molecular cloning and functional characterization of components of the capsule biosynthesis complex of Neisseria meningitidis serogroup A: toward in vitro vaccine production. J Biol Chem. 2014;289(28):19395-407. [PubMed ID: 24849599]. [PubMed Central ID: PMC4094051]. https://doi.org/10.1074/jbc.M114.575142.
-
67.
Marin MA, Fonseca E, Encinas F, Freitas F, Camargo DA, Coimbra RS, et al. The invasive Neisseria meningitidis MenC CC103 from Brazil is characterized by an accessory gene repertoire. Sci Rep. 2017;7(1):1617. [PubMed ID: 28487566]. [PubMed Central ID: PMC5431661]. https://doi.org/10.1038/s41598-017-01671-x.
-
68.
Vackova Z, Křížová P, Jandova Z, Musilek M, Kozakova J. [The possibilities for the implementation of whole genome sequencing (WGS) of Neisseria meningitidis in molecular surveillance of invasive meningococcal disease in the Czech Republic]. Epidemiol Mikrobiol Imunol. 2017;66(1):50-2.
-
69.
Heidrich N, Hagmann A, Bauriedl S, Vogel J, Schoen C. The CRISPR/Cas system in Neisseria meningitidis affects bacterial adhesion to human nasopharyngeal epithelial cells. RNA Biol. 2019;16(4):390-6. [PubMed ID: 30059276]. [PubMed Central ID: PMC6546440]. https://doi.org/10.1080/15476286.2018.1486660.
-
70.
Seifert HS. Location, Location, Location-Commensalism, Damage and Evolution of the Pathogenic Neisseria. J Mol Biol. 2019;431(16):3010-4. [PubMed ID: 30986425]. https://doi.org/10.1016/j.jmb.2019.04.007.
-
71.
Crew PE, McNamara L, Waldron PE, McCulley L, Jones SC, Bersoff-Matcha SJ. Unusual Neisseria species as a cause of infection in patients taking eculizumab. J Infect. 2019;78(2):113-8. [PubMed ID: 30408494]. [PubMed Central ID: PMC7224403]. https://doi.org/10.1016/j.jinf.2018.10.015.
-
72.
Gbesemete D, Laver JR, de Graaf H, Ibrahim M, Vaughan A, Faust S, et al. Protocol for a controlled human infection with genetically modified Neisseria lactamica expressing the meningococcal vaccine antigen NadA: a potent new technique for experimental medicine. BMJ Open. 2019;9(4). e026544. [PubMed ID: 31048443]. [PubMed Central ID: PMC6501966]. https://doi.org/10.1136/bmjopen-2018-026544.
-
73.
Clemence MEA, Maiden MCJ, Harrison OB. Characterization of Capsule Genes in Non-Pathogenic Neisseria Species. Microb Genom. 2018;4(9). e000208. [PubMed ID: 30074474]. [PubMed Central ID: PMC6202450]. https://doi.org/10.1099/mgen.0.000208.
-
74.
Yero D, Vipond C, Climent Y, Sardinas G, Feavers IM, Pajon R. Variation in the Neisseria meningitidis FadL-like protein: an evolutionary model for a relatively low-abundance surface antigen. Microbiology. 2010;156(Pt 12):3596-608. [PubMed ID: 20817647]. https://doi.org/10.1099/mic.0.043182-0.
-
75.
Krizova P, Honskus M. Surveillance of invasive meningococcal disease based on whole genome sequencing (WGS), Czech Republic, 2015. Epidemiol Microbiol Imunol. 2018;67(2):64-73. [PubMed ID: 30126283].
-
76.
Tran TX, Le TT, Trieu LP, Austin CM, Van Quyen D, Nguyen HM. Whole-genome sequencing and characterization of an antibiotic resistant Neisseria meningitidis B isolate from a military unit in Vietnam. Ann Clin Microbiol Antimicrob. 2019;18(1):16. [PubMed ID: 31060558]. [PubMed Central ID: PMC6501280]. https://doi.org/10.1186/s12941-019-0315-z.
-
77.
van Kessel F, van den Ende C, Oordt-Speets AM, Kyaw MH. Outbreaks of meningococcal meningitis in non-African countries over the last 50 years: a systematic review. J Glob Health. 2019;9(1):10411. [PubMed ID: 30937163]. [PubMed Central ID: PMC6441124]. https://doi.org/10.7189/jogh.09.010411.
-
78.
Tavana A, Ataee RA. Meningococcal Meningitis Control in Iran: Five Year Comparative Study 2000-2004. J Med Sci (Faisalabad). 2009;9(1):51-4. https://doi.org/10.3923/jms.2009.51.54.
-
79.
Fazio C, Neri A, Vacca P, Ciammaruconi A, Arghittu M, Barbui AM, et al. Cocirculation of Hajj and non-Hajj strains among serogroup W meningococci in Italy, 2000 to 2016. Euro Surveill. 2019;24(4). [PubMed ID: 30696530]. [PubMed Central ID: PMC6352001]. https://doi.org/10.2807/1560-7917.ES.2019.24.4.1800183.
-
80.
Tzeng YL, Bazan JA, Turner AN, Wang X, Retchless AC, Read TD, et al. Emergence of a new Neisseria meningitidis clonal complex 11 lineage 11.2 clade as an effective urogenital pathogen. Proc Natl Acad Sci U S A. 2017;114(16):4237-42. [PubMed ID: 28373547]. [PubMed Central ID: PMC5402416]. https://doi.org/10.1073/pnas.1620971114.
-
81.
Zouheir Y, Atany T, Boudebouch N. Emergence and spread of resistant N. meningitidis implicated in invasive meningococcal diseases during the past decade (2008-2017). J Antibiot (Tokyo). 2019;72(3):185-8. [PubMed ID: 30479393]. https://doi.org/10.1038/s41429-018-0125-0.
-
82.
Martin I, Sawatzky P, Allen V, Lefebvre B, Hoang L, Naidu P, et al. Multidrug-resistant and extensively drug-resistant Neisseria gonorrhoeae in Canada, 2012-2016. Can Commun Dis Rep. 2019;45(2-3):45-53. [PubMed ID: 31015818]. [PubMed Central ID: PMC6461123]. https://doi.org/10.14745/ccdr.v45i23a01.
-
83.
Rotman E, Webber DM, Seifert HS. Analyzing Neisseria gonorrhoeae Pilin Antigenic Variation Using 454 Sequencing Technology. J Bacteriol. 2016;198(18):2470-82. [PubMed ID: 27381912]. [PubMed Central ID: PMC4999939]. https://doi.org/10.1128/JB.00330-16.
-
84.
Coldiron ME, Alcoba G, Ciglenecki I, Hitchings M, Djibo A, Page AL, et al. Ciprofloxacin for contacts of cases of meningococcal meningitis as an epidemic response: study protocol for a cluster-randomized trial. Trials. 2017;18(1):294. [PubMed ID: 28646924]. [PubMed Central ID: PMC5482956]. https://doi.org/10.1186/s13063-017-2028-y.
-
85.
Harrison LH. Vaccines for prevention of group B meningococcal disease: Not your father's vaccines. Vaccine. 2015;33 Suppl 4:D32-8. [PubMed ID: 26116255]. https://doi.org/10.1016/j.vaccine.2015.05.101.