Abstract
Background:
Probiotic products with valuable nutritional and therapeutic properties have attracted great attention in the fields of industry, nutrition, and medicine.Objectives:
The present study aimed to investigate the survival of Lactobacillus plantarum probiotic bacteria in capsulated and non-capsulated forms in malt products during storage. We also evaluated the pH, brix, sugar content, and organoleptic properties of the three products, a control malt (non-probiotic extract), an extract containing the probiotic bacteria without capsules, and an extract containing encapsulated probiotic bacteria.Methods:
The probiotic strain of L. plantarum was inoculated into a barley malt extract in free and encapsulated forms. Bacterial microencapsulation was performed using the extrusion technique with sodium alginate. In addition, bacterial viability was determined using the mixed culture method in MRS agar medium at the temperature of 37°C for 48 hours. Data analysis was performed using one-way analysis of variance (ANOVA) in the Minitab 16 software.Results:
The count of L. plantarum in the non-capsulated form did not change significantly although it increased in the capsulated form. No significant changes were observed in the sugar content, pH, and brix during the storage of the three products. Moreover, the sensory acceptance of malt with capsulated bacteria was higher compared to the other samples.Conclusions:
According to the results, the probiotic strain of L. plantarum in the free and microencapsulated forms was maintained in the barley malt extract for two months of storage at the temperature of 4°C. Therefore, it seems that barley malt extract may be a favorable environment for the preservation of probiotic L. plantarum.Keywords
Malt Probiotic Microencapsulation Lactobacillus plantarum Functional Food
1. Background
Functional foods are products with beneficial effects on human health and provide basic nutrients. As such, foods fortified with probiotics are among the most functional food products (1). Probiotics are living microorganisms with beneficial effects on the health status of the host through improving the intestinal microbial balance (2). For this effect to be exerted, probiotic products must be consumed in appropriate amounts (more than 100 grams of probiotic product containing at least 7 log CFU/g of probiotic microorganisms) (3).
Probiotic microorganisms are commonly used in dairy products, juices, and meat products (4). Cereals may be a healthier choice for non-dairy probiotic food items as they lack disadvantages such as lactose intolerance and have no effects on cholesterol (5). Therefore, the production of new functional products that simultaneously have the beneficial effects of cereals and probiotic bacteria could be of great interest (6).
Malt is produced through a controlled germination process, followed by the desiccation of cereal grains (especially barley) (7). During the germination phase of barley and due to increased enzymatic activity, nutrients such as vitamins B and essential amino acids also increase. Malt contains all vitamins B, as well as iron, zinc, calcium, magnesium, phosphorus, and amino acids (8). Various cereal malts are used in food industries, including beverages, pastries, baby food, malt vinegar, breakfast cereals, malt extracts, and biscuits, while they are also used as additives (sweetener, flavoring, and coloring) (9).
Some studies have investigated the effects of malt and cereal substrates on the growth and survival of probiotic bacteria. For instance, Marhamatizadeh et al. evaluated the effects of malt extract on increasing the growth of Lactobacillus acidophilus and Bifidobacterium bifidum probiotic bacteria in probiotic milk and yoghurt (10). On the other hand, Mohammadi et al. investigated the biochemical and microbiological properties of several probiotic strains in non-alcoholic beer during the storage period (11). In another research, Zakipour Rhomabadi et al. assessed the production of a synbiotic malt beverage using inulin and various species of Lactobacillus bacteria (12). Charalampopoulos et al. also studied the growth of potentially probiotic lactic acid bacteria in cereal-based substrates (13).
In the study conducted by Rozada-Sanchez et al., Bifidobacterium spp. was evaluated for the production of a potentially probiotic malt-based beverage (6). Rathore et al. also studied the production of potentially probiotic beverages using single and mixed cereal substrates fermented with lactic acid bacteria cultures (5). In another study, Nakkarach and Withayagiat compared synbiotic beverages produced from riceberry malt extract using selected free and encapsulated probiotic lactic acid bacteria (14).
In order to produce new probiotic products, it is essential to assess the viability of probiotic microorganisms and use appropriate methods to improve their viability if necessary. Microencapsulation is an optimal approach to increasing the viability of probiotics in stressful conditions (3). In this process, small capsules are formed with different sizes, ranging from a few micrometers to a few millimeters, and contain a central nucleus, such as probiotics (15). Different methods and materials are used for micro-coating; alginate is such an example, which is generally preferred given its biocompatibility, non-toxicity, low cost, and available process (16).
2. Objectives
The present study aimed to evaluate the effect of malt extract on the survival of the probiotic strain Lactobacillus plantarum in free and microencapsulated forms with calcium alginate.
3. Methods
The probiotic bacteria used in the study (L. plantarum) were prepared in the freeze-dried (purchased from Tehran Zist Takhmir Pharmaceutical Company, Iran), and the barley malt extract was the product of Pardis Shirin Malt Factory. MRS broth and agar were obtained from Ibresco chemical (Merck, Germany).
3.1. Lactobacillus plantarum Preparation
In order to reactivate the bacteria, one gram of L. plantarum was transferred to 100 milliliters of sterile MRS broth culture medium, mixed evenly, and incubated at the temperature of 37°C for 48 hours (17). Afterwards, 20 milliliters of culture medium prepared in the activation stage was transferred to 500 milliliters of fresh sterile MRS broth culture medium and re-incubated in the same conditions (18). At the next stage, the cells were harvested via centrifugation at 3,200 rpm for four minutes at 4°C. The cells were dispersed in the appropriate volume of 0.85% sterile saline solution, and the cell concentration of the suspension was determined for the inoculation of the malt samples (free and microencapsulated) (17).
3.2. Encapsulation
The extrusion technique was employed for the microencapsulation of the bacteria. Initially, three milliliters of the bacterial suspension was mixed with 27 milliliters of a sterile 2% sodium alginate solution. In sterile conditions, the obtained mixture was injected into 10 milliliters of 0.1 M calcium chloride solution using an insulin syringe. Following that, the mixture was allowed to harden for 30 minutes, and the produced beads were separated using a sterile filter paper (19).
3.3. Preparation of Test Samples
At this stage, three samples of the malt extract were prepared and stored at the temperature of 4°C. The samples included control malt (extract without probiotic L. plantarum), malt extract containing the probiotic bacteria in the free form (3 mL of bacterial suspension prepared after centrifugation mixed with 27 milliliters of sterile 0.85% saline solution with the final mixture added to 300 grams of barley malt extract in sterile conditions), and malt extract containing the microencapsulated probiotic bacteria.
3.4. Chemical and Microbial Tests
The count of L. plantarum and chemical tests (sugar content, pH, and brix) were determined at two-week intervals (20, 21). To release the microencapsulated bacteria from the beads before culturing, 10 grams of the malt containing the microencapsulated bacteria was homogenized in 90 milliliters of 0.1 M sterile phosphate buffer (pH: 7) in a stirrer for 30 minutes. After the preparation of the serial dilutions, the bacterial count was determined using the pour-plate technique in MRS agar medium (22).
3.5. Sensory Evaluation of the Malt Samples
The sensory analysis of the malt samples was performed by 30 semi-trained panelists using the nine-point hedonic method in week nine of the storage period (23).
3.6. Statistical Analysis
This experiment was performed with a completely randomized design, three treatments, and three replications. Data analysis was performed in the Minitab software version 16.0 using one-way analysis of variance (ANOVA). The significant differences between the mean values were determined using Fisher’s least significant difference (LSD) at the significance level of P < 0.05.
4. Results
According to the comparison of the count of L. plantarum (log CFU/mL) during shelf life (Table 1), the bacterial count did not change significantly in the free form, while it increased in the encapsulated form of the bacteria. In addition, bacterial enumeration before addition to the malt samples indicated that bacterial count per milliliter of the suspension was 10.7 log CFU/mL. Notably, three milliliters of the suspension was added to 27 milliliters of alginate or saline solution, followed by addition to 300 grams of malt. As a result, the bacterial count decreased in the malt samples on the first day of enumeration. The initial concentration of the bacteria in the malt samples was estimated at 6.98 and 7.44 log CFU/mL in the capsulated and non-capsulated bacteria, respectively.
Viability of Lactobacillus plantarum in Malt Samples a
Measurement Day | Bacterial Enumeration (log CFU/g) | |
---|---|---|
Malt with Free Probiotic Bacteria | Malt with Microencapsulated Probiotic Bacteria | |
1 | 7.44 A | 6.98 B |
15 | 6.71 A | 6.92 B |
30 | 6.73 A | 6.95 B |
45 | 7.43 A | 7.18 AB |
58 | 7.10 A | 7.25 A |
Statistical analyses revealed that during two months of storage, the pH and sugar content of the malt samples did not change significantly (Tables 2 and 3). In addition, the obtained results indicated that although storage time had a significant effect on the brix of the malt samples, the changes were small and in the tenth unit (Table 4). According to the results of sensory evaluation (Figure 1), the malt samples containing microencapsulated probiotic bacteria had better sensory acceptance compared to the other samples. In other words, microencapsulation improved the sensory properties of the product.
Mean pH Changes of Malt Samples during Storage a
Measurement Day | pH | ||
---|---|---|---|
Control Malt | Malt with Free Probiotic Bacteria | Malt with Microencapsulated Probiotic Bacteria | |
1 | 4.0 ± 26.06 A | 4.0 ± 32.08 A | 4.0 ± 78.03 A |
15 | 4.0 ± 3.02 A | 4.0 ± 34.04 A | 4.0 ± 79.03 A |
30 | 4.0 ± 26.02 A | 4.0 ± 25.03 A | 4.0 ± 78.03 A |
45 | 4.0 ± 26.04 A | 4.0 ± 29.03 A | 4.0 ± 80.02 A |
58 | 4.0 ± 28.05 A | 4.0 ± 28.03 A | 4.0 ± 81.01 A |
Mean Changes in Sugar Content of Malt Samples During Storage a
Measurement Day | Sugar Content (%) | ||
---|---|---|---|
Control Malt | Malt with Free Probiotic Bacteria | Malt with Microencapsulated Probiotic Bacteria | |
1 | 58.12 ± 1.45 A | 54.13 ± 0.8 A | 50.18 ± 0.79 A |
15 | 57.21 ± 1.03 A | 53.18 ± 0.47 A | 51.77 ± 0.84 A |
30 | 57.79 ± 1.09 A | 53.66 ± 0.89 A | 51.79 ± 1.55 A |
45 | 58.81 ± 1.07 A | 52.7 ± 1.03 A | 50.35 ± 0.6 A |
58 | 59.52 ± 1.09 A | 53.78 ± 3.22 A | 51.94 ± 0.65 A |
Mean Brix Changes of Malt Samples during Storage a
Measurement Day | Brix | ||
---|---|---|---|
Control Malt | Malt with Free Probiotic Bacteria | Malt with Microencapsulated Probiotic Bacteria | |
1 | 77.0 ± 5.00 B | 68.0 ± 00.00 C | 71.0 ± 00.00 B |
15 | 77.0 ± 00.00 C | 68.0 ± 00.00 C | 71.0 ± 33.29 A |
30 | 77.0 ± 00.00 C | 68.0 ± 33.29 B | 71.0 ± 00.00 B |
45 | 77.0 ± 33.29 B | 68.0 ± 33.29 B | 71.0 ± 00.00 B |
58 | 77.0 ± 83.29 A | 69.0 ± 00.00 A | 71.0 ± 00.00 B |
Mean sensory acceptance according to Hedonic test.
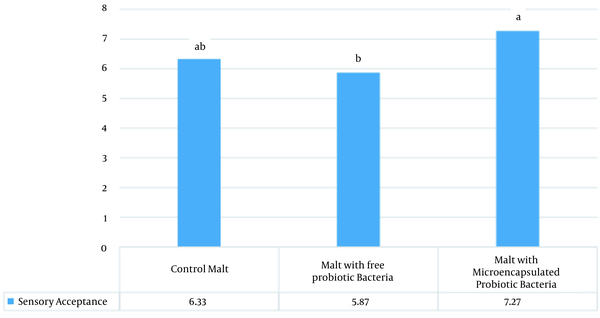
5. Discussion
Cereals are among the most favorable substrates for the development of food products containing probiotic bacteria. Cereals are an abundant source of protein, carbohydrates, vitamins, minerals, and fiber. They also contain indigestible carbohydrates, which exert positive effects on human health (24). In the present study, barley malt extract was selected, and the survival of the probiotic bacterium L. plantarum was evaluated in the product. The reason for choosing this bacterium was that it has been classified as a beneficial and essential bacterium in the intestinal microbial flora owing to its high resistance to pasteurization (25).
According to the national standard of Iran, barley malt extract for home and industrial use should have a sugar content of 52 and 40 and 75 and 60% brix, respectively (20). In the present study, the sugar content of the control malt extract, malt containing free bacteria, and malt containing microencapsulated bacteria was respectively estimated at 58.12, 54.13, and 50.18% on the first day of production, and the brix of these samples was calculated at 77.5, 68, and 71%, respectively. Due to the diluting effect of the suspension and alginate, the added probiotic bacteria to the malt extract in the free and microencapsulated states reduced the sugar content of the bacteria-containing samples compared to the control samples. However, the presence of alginate in the malt samples containing the encapsulated bacteria increased the brix of soluble solids compared to the malt extract containing the free bacteria.
In both of the malt samples in the current research, the count of probiotic bacteria remained at a favorable level during two months of storage and even slightly increased in the microencapsulated state. Numerous factors affect the survival of probiotic bacteria throughout the processing and storage of the product. Some of these factors include the intrinsic properties of the probiotic bacterial strain, product pH, storage temperature, the presence of microbial inhibitors, and dissolved oxygen (26).
Due to its high content of fermentable sugars and nitrogenous compounds, malt extract promotes the growth of probiotic bacteria (26). Some studies have examined the effects of malt and cereal substrates on the growth and survival of probiotic bacteria. For instance, Rathore et al. reported that the growth of L. plantarum and L. acidophilus increased in the culture media containing 5% malt. In another study, malt extract was added to milk and yogurt at the concentrations of 2, 4, and 6% to investigate its effect on the increased growth of probiotic bacteria L. acidophilus and B. bifidum (5). The results of the mentioned study indicated that during the shelf life of the products, the growth rate of probiotics increased at the higher concentrations of the malt extract (10).
In the study conducted by Charalampopoulos and Pandiella, the higher survival of the probiotic bacterium L. plantarum was observed at the concentrations of 20 and 30% of malt extract and attributed to the presence of sugar and other protective compounds (26). In the present study, the barley malt extract had a higher brix and sugar content and was evaluated in terms of the survival of probiotic L. plantarum, and bacterial survival was maintained in both samples.
Oligosaccharides and dietary fiber in malt extract are among the compounds that are effective in the protection of bacteria. Compounds such as raffinose and dietary fiber are found in legumes, cereals, and fruits and are known to protect probiotics in various products (26). Cereals, legumes, fruits, and vegetables also contain non-digestible fibers such as cellulose, which may protect probiotic microorganisms in the intestinal tract (27).
In a study conducted by Landry et al., the survival rate of the probiotic strains of L. plantarum was assessed in honey, and the findings indicated that all the studied strains had favorable survival in honey during 28 days of storage at the temperature of 4°C (28). In the mentioned study, the mean moisture and sugar contents of the honey samples was estimated at 17.9 and 61.5%, respectively, which is in line with the results of the present study. Bacterial survival in honey has been attributed to the presence of oligosaccharides (i.e., fructo and gluco oligosaccharides), which are known to have prebiotic properties as well. In the study by Landry et al., the counts of the three strains of L. plantarum did not increase due to the low moisture and protein content of honey. Consistently, the malt extracts used in the current research had a high sugar content and a low moisture content. Considering the optimal growth temperature of this bacterium (37°C), preserving the malt samples at the temperature of 4°C caused no significant changes in the bacteria count. At the temperature of 4°C, the metabolism of bacterial cells slows down (28), and fermentable sugars are slowly metabolized (26).
In the present study, the pH of the control malt extract, malt containing the free bacteria, and malt containing the microencapsulated bacteria was estimated at 4.26, 4.32, and 4.78 on the first day of production, respectively, and no significant changes were observed during storage. Similarly, Landry et al. reported the mean pH of the honey samples to be 4.32, which did not change significantly over time possibly due to the slow metabolism of the bacteria. In our research, the malt extracts containing the microencapsulated bacteria had a higher pH compared to the samples containing the free bacteria, which could explain the slight increase in the bacterial counts of these samples (28). In this regard, the findings of Giraud et al. showed that the pH of six is the most suitable pH for increasing the count of L. plantarum (29). In another study, L. plantarum had the highest bioavailability in a malt beverage, with the initial pH reported to be 4.8 during refrigerated storage (11).
In the current research, the results of sensory evaluation indicated that the malt containing the microencapsulated bacteria had better acceptance despite a lower sugar content compared to the other two samples. This finding could be attributed to the presence of alginate and its effect on the texture of the product. According to Nakkarach and Withayagiat, microencapsulation with alginate hydrogel could significantly improve the survival of L. plantarum in gastrointestinal conditions (14). Therefore, alginate micro-coating may affect the survival of bacteria in barley malt extract and the gastrointestinal tract, thereby improving the sensory acceptance of the product and reducing the sugar content of the product. Considering the costs of micro-coating, adding bacteria in the free form is an alternative in this regard.
5.1. Conclusion
According to the results, the survival of the probiotic bacterium L. plantarum was maintained in both the free and microencapsulated states in the barley malt extract stored at the temperature of 4°C for almost two months. On the other hand, the sugar content, pH, and brix of the products did not change significantly during the storage period. Considering the sensory acceptance of these products, it seems that barley malt extract could be a favorable environment for the preservation of the probiotic bacterium L. plantarum. Further research is required to evaluate the effects of bacteria on the other nutritional properties of a product.
References
-
1.
Perricone M, Corbo MR, Sinigaglia M, Speranza B, Bevilacqua A. Viability of Lactobacillus reuteri in fruit juices. J Funct Foods. 2014;10:421-6. https://doi.org/10.1016/j.jff.2014.07.020.
-
2.
Mattila-Sandholm T, Myllärinen P, Crittenden R, Mogensen G, Fondén R, Saarela M. Technological challenges for future probiotic foods. Int Dairy J. 2002;12(2-3):173-82. https://doi.org/10.1016/s0958-6946(01)00099-1.
-
3.
Trabelsi I, Bejar W, Ayadi D, Chouayekh H, Kammoun R, Bejar S, et al. Encapsulation in alginate and alginate coated-chitosan improved the survival of newly probiotic in oxgall and gastric juice. Int J Biol Macromol. 2013;61:36-42. [PubMed ID: 23817092]. https://doi.org/10.1016/j.ijbiomac.2013.06.035.
-
4.
Gomes AM, Malcata F. Bifidobacterium spp. and Lactobacillus acidophilus: Biological, biochemical, technological and therapeutical properties relevant for use as probiotics. Trends Food Sci Technol. 1999;10(4-5):139-57. https://doi.org/10.1016/s0924-2244(99)00033-3.
-
5.
Rathore S, Salmeron I, Pandiella SS. Production of potentially probiotic beverages using single and mixed cereal substrates fermented with lactic acid bacteria cultures. Food Microbiol. 2012;30(1):239-44. [PubMed ID: 22265307]. https://doi.org/10.1016/j.fm.2011.09.001.
-
6.
Rozada-Sánchez R, Sattur AP, Thomas K, Pandiella SS. Evaluation of Bifidobacterium spp. for the production of a potentially probiotic malt-based beverage. Process Biochemistry. 2008;43(8):848-54. https://doi.org/10.1016/j.procbio.2008.04.002.
-
7.
Jadhav SJ, Lutz SE, Ghorpade VM, Salunkhe DK. Barley: Chemistry and value-added processing. Crit Rev Food Sci Nutr. 1998;38(2):123-71. [PubMed ID: 9526682]. https://doi.org/10.1080/10408699891274183.
-
8.
Jalali S, Jalali H, Hosseini Ghaboos SH. [Effect of pumpkin powder and malt on physicochemical, textural and sensory properties of sponge cake]. Iranian Journal Food Science and Technology Research. 2018;14(4):451-60.
-
9.
Kashiri M, Maghsoudlou Y, Kashaninezhad M, Hosseini SH. [Comparison of physico-chemical properties of triticale and barley malt]. Iranian Journal of Food Science and Technology. 2008;5(3):61-8. Persian.
-
10.
Marhamatizadeh MH, Karmand M, Farokhi AR, Rafatjou R, Rezazadeh S. The effects of malt extract on the increasing growth of probiotic bacteria Lactobacillus acidophilus and Bifidobacterium bifidum in probiotic milk and yoghurt. J Food Tech Nutr. 2011;8(2 (30)):78-84. Persian.
-
11.
Mohammadi R, Zabihzadeh M, Delshadian Z, Sarlak Z, Mortazavian AM, Hosseini M. [Study on the biochemical and microbiological characteristics of several probiotic strains in non-alcoholic beer during storage period]. Iranian Journal of Nutrition Sciences & Food Technology. 2016;11(3):53-62. Persian.
-
12.
Zakipour Rhomabadi N, Sohrabvandi S, Ruzbeh Nasiraie L. [Production of synbiotic malt beverage using inulin and different probiotic strains of Lactobacillus bacteria]. Iranian Journal of Nutrition Sciences & Food Technology. 2018;13(3):39-46. Persian.
-
13.
Charalampopoulos D, Pandiella SS, Webb C. Growth studies of potentially probiotic lactic acid bacteria in cereal-based substrates. J Appl Microbiol. 2002;92(5):851-9. [PubMed ID: 11972688]. https://doi.org/10.1046/j.1365-2672.2002.01592.x.
-
14.
Nakkarach A, Withayagiat U. Comparison of synbiotic beverages produced from riceberry malt extract using selected free and encapsulated probiotic lactic acid bacteria. Agric Nat Resour. 2018;52(5):467-76. https://doi.org/10.1016/j.anres.2018.11.013.
-
15.
Heidebach T, Forst P, Kulozik U. Microencapsulation of probiotic cells for food applications. Crit Rev Food Sci Nutr. 2012;52(4):291-311. [PubMed ID: 22332594]. https://doi.org/10.1080/10408398.2010.499801.
-
16.
Burgain J, Gaiani C, Linder M, Scher J. Encapsulation of probiotic living cells: From laboratory scale to industrial applications. J Food Eng. 2011;104(4):467-83. https://doi.org/10.1016/j.jfoodeng.2010.12.031.
-
17.
Homayouni A, Azizi A, Ehsani MR, Yarmand MS, Razavi SH. Effect of microencapsulation and resistant starch on the probiotic survival and sensory properties of synbiotic ice cream. Food Chem. 2008;111:50-5. https://doi.org/10.1016/j.foodchem.2008.03.036.
-
18.
Haynes IN, Playne MJ. Survival of probiotic cultures in low-fat ice-cream. Aust J Dairy Technol. 2002;57(1):10.
-
19.
Mirzaei H, Pourjafar H, Homayouni A. Effect of calcium alginate and resistant starch microencapsulation on the survival rate of Lactobacillus acidophilus La5 and sensory properties in Iranian white brined cheese. Food Chem. 2012;132(4):1966-70. https://doi.org/10.1016/j.foodchem.2011.12.033.
-
20.
Iranian National Standardization Organization. [Barley malt extract – Specifications and test methods]. ICS: 67.060. 2nd ed. Tehran, Iran: Iranian National Standardization Organization; 2014. Persian.
-
21.
Sheu TY, Marshall RT. Microentrapment of Lactobacilli in calcium alginate gels. J Food Sci. 1993;58(3):557-61. https://doi.org/10.1111/j.1365-2621.1993.tb04323.x.
-
22.
Sultana K, Godward G, Reynolds N, Arumugaswamy R, Peiris P, Kailasapathy K. Encapsulation of probiotic bacteria with alginate-starch and evaluation of survival in simulated gastrointestinal conditions and in yoghurt. Int J Food Microbiol. 2000;62(1-2):47-55. [PubMed ID: 11139021]. https://doi.org/10.1016/s0168-1605(00)00380-9.
-
23.
Poste LM, Mackie DA, Butler G, Larmond E. Laboratory methods for sensory analysis of food. Michigan, USA: Canada Communication Group Pub Centre; 1991.
-
24.
Savedboworn W, Niyomrat S, Naknovn J, Phattayakorn K. Impact of inulin on viability and storage stability of probiotic Lactobacillus plantarum TISTR 2075 in fermented rice extract. Agric Nat Resour. 2017;51(6):463-9. https://doi.org/10.1016/j.anres.2018.03.008.
-
25.
Frazier W, Westhoff D. Mortazavi A, Kashani Nejad M, Ziaolhagh H, translators. [Food microbiology]. Mashhad, Iran: Ferdowsi University of Mashhad; 2007. Persian.
-
26.
Charalampopoulos D, Pandiella SS. Survival of human derived Lactobacillus plantarum in fermented cereal extracts during refrigerated storage. LWT - Food Sci Technol. 2010;43(3):431-5. https://doi.org/10.1016/j.lwt.2009.09.006.
-
27.
Rasika DMD, Vidanarachchi JK, Luiz SF, Azeredo DRP, Cruz AG, Ranadheera CS. Probiotic delivery through non-dairy plant-based food matrices. Agriculture. 2021;11(7):599. https://doi.org/10.3390/agriculture11070599.
-
28.
Landry BKU, Francois ZN, Wang RY, Taicheng Z, Li Y. Viability and stress response of putative probiotic Lactobacillus plantarum strains in honey environment. Probiotics Antimicrob Proteins. 2018;10(4):629-37. [PubMed ID: 29196921]. https://doi.org/10.1007/s12602-017-9358-7.
-
29.
Giraud E, Lelong B, Raimbault M. Influence of pH and initial lactate concentration on the growth of Lactobacillus plantarum. Appl Microbiol Biotechnol. 1991;36(1):96-9. https://doi.org/10.1007/bf00164706.