Abstract
Background:
Erythropoietin plays a significant role in the growth of red blood cells, hemoglobin levels, and tissue oxygenation in critically ill patients, as well as anti-inflammatory and neuroprotective effects.Objectives:
This study aimed to evaluate the effect of recombinant erythropoietin on improving COVID-19 patients.Methods:
This study was conducted on 20 COVID-19 participants with hemoglobin of ≥ 9. The inclusion criteria was at least one severe COVID-19 symptom/sign in this interventional study. The primary outcome was a combination of hospital stay length and paraclinical evaluation (LDH and hemoglobin level). The outcomes and side effects were evaluated on day 0 (before the intervention) and five (post-intervention).Results:
The mean hemoglobin level was 10 ± 1.1 gr/dL in the intervention group and 8 ± 0.7 gr/dL in the control group post-treatment, indicating a significant difference between the groups (P = 0.04). The mean hospital stay length (6 ± 2 days) in the intervention group was significantly less than the control group (9 ± 4 days) (P = 0.001). At the end of the treatment, the mean LDH was significantly lower in the intervention group (503 ± 264 µ/L) than in the control group (725 ± 320 µ/L; P = 0.017).Conclusions:
According to the results, this study provides the first solid evidence for the positive effects of recombinant erythropoietin on COVID-19.Keywords
Recombinant Erythropoietin COVID-19 Coronavirus 2 Disease, and Immunity
1. Background
The severe acute respiratory syndrome Coronavirus 2 (SARS-CoV-2) outbreak was reported in late 2019 in Wuhan, China, which turned into a pandemic within several months with millions of reported cases (1). The range of the clinical spectrum of COVID-19 varies from asymptomatic to fatal pneumonia (2). According to the severity of symptoms, COVID‐19 has been classified into four levels, including mild, moderate, severe, and critical (3). Various criteria, including dyspnea, characterize the severe and critical levels of COVID-19, oxygen saturation of less than 93% in ambient air, respiratory system dysfunction, septic shock, and multiple organ abnormalities (3). Therefore, the use of immediate and effective treatments to increase the immune response and strengthen the respiratory system of patients with severe or critical levels of COVID-19 is very vital to save lives (4). Although vaccines and medications have been developed, treatments for the disease are still controversial, especially in severe cases (5, 6). When severe hypoxemia is present with COVID-19, pneumolysis rapidly develops and mechanical ventilation is required (7). Therefore, these patients’ need for better tissue oxygenation, and the effects of hemoglobin on tissue oxygenation have received research attention. Huang et al. reported a reduction in hemoglobin levels in 38.2% of COVID-19 patients (8). In addition, Wang et al. reported low hemoglobin levels in 19.23% of COVID-19 patients, despite a concurrently elevated hemoglobin level in 7.69% (9). However, Xu et al., who examined asymptomatic patients, did not report hemoglobin level reduction (10).
Erythropoietin is a significant growth factor increasing red blood cells, hemoglobin levels, and tissue oxygenation among patients in critical conditions (11). Additionally, erythropoietin exhibits neuroprotective and anti-inflammatory properties, which have led to hypothesized effects of erythropoietin prescription in COVID-19 patients (12). In a brief review, Ehrenreich et al. have introduced erythropoietin as a potentially effective medication for relieving the significant problems of severely affected COVID-19 patients (12). Moreover, Fishbane and Hirsch reported that using erythropoiesis-stimulating agents (ESAs) for treatment in hospitalized COVID-19 patients with anemia may be a good idea (13). However, the efficacy of ESAs can be limited due to inflammation in most cases, so more research is needed to address the possible efficacy of these agents in these patients (13). Furthermore, in another study, it has been reported that antiviral treatment along with recombinant human erythropoietin may reduce respiratory distress syndrome and confront the severe acute respiratory syndrome in patients with COVID-19. However, more well‐organized clinical trials are suggested to be conducted to assess the potential clinical benefits of erythropoietin with its possible adverse effects in COVID‐19 patients (14). Despite the potential positive effect of ESAs on respiratory performance indicators based on the studies, a sufficient number of relevant clinical trials have yet to demonstrate the significant efficacy of ESAs among critically ill COVID-19 patients in a variety of populations. In addition, the interventional studies that investigated the possibility of erythropoietin side effects (such as increasing patients' prothrombotic status and embolism) in COVID-19 patients were inconclusive.
2. Objectives
This study aimed to evaluate the immunity-induction and effectiveness of recombinant erythropoietin for patients with COVID-19 in a randomized two-armed study.
3. Methods
3.1. Study Patients
The flowchart of the study population is presented in Figure 1. This study follows the CONSORT 2010 guidelines for reporting clinical trials involving parallel groups (15). The entire protocol of this study is published in another report (16).
Flowchart of the study population
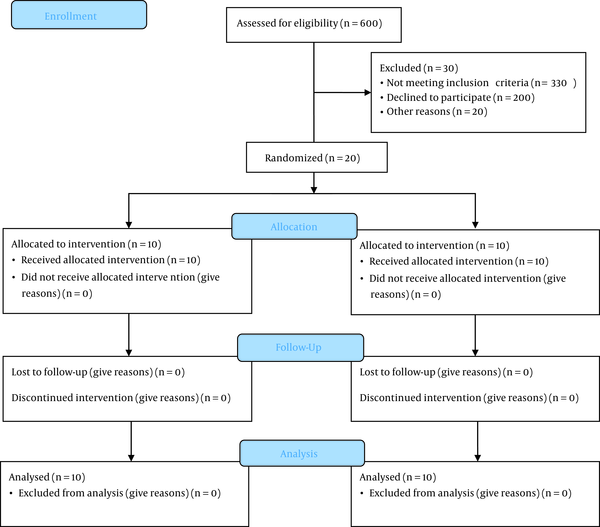
This study was conducted on all patients (n = 20 participants) with a definitive diagnosis of COVID-19 visiting Shahid Mohammadi Hospital of Bandar-e Abbas, Iran. the inclusion criteria were hemoglobin of ≥ 9 and at least one severe COVID-19 symptom/sign such as tachypnea: respiration rate > 30 per minute; hypoxemia: oxygen saturation ≤ 93; arterial oxygen partial pressure ratio ≤ 300; pulmonary infiltration: ≥ 50% of the pulmonary field in 24-48 hours; progressive lymphocytopenia, LDH > 245 U/I, CRP > 100. The exclusion criteria were a history of coagulation disorders, thrombosis, deep vein thrombosis, chronic pulmonary disease, diabetes mellitus, immunodeficiency, end-stage renal disease, hepatic disease, taking contraceptives, systolic blood pressure > 160 mmHg, diastolic blood pressure > 90 mmHg, age > 65 years, erythropoietin > 500, history of myocardial infarction or unstable angina, and history of malignancies. The sampling continued until 20 individuals were selected.
3.2. Protocol of Intervention
The patients were randomly assigned to the intervention and control groups using online randomization tools with a 1: 1 ratio after providing informed consent for participation. The patients were blinded to their group allocation. The intervention group comprised ten patients who received standard of care (SOC) based on the Iranian National Committee of COVID-19 protocols and recombinant erythropoietin (EPREX Manufactured by Johnson and Johnson Pharmaceutical Company with 300 units/kg or 4000 IU subcutaneously three times a day for five days. The patients also received Enoxaparin 1 mg/kg daily subcutaneously to prevent thrombosis in the intervention group. The control group received SOC based on the Iranian National Committee of COVID-19 protocols and the placebo. The primary outcome was a combination of hospital stay length and paraclinical evaluation of the patients (LDH and hemoglobin level), as well as the side effects of the medication such as thrombosis, deep vein thrombosis, coagulation disorders, myocardial infarction, CVA, or embolism. The outcomes and side effects were evaluated on day 0 before the intervention and day five after intervention.
3.3. Statistical Analysis
The collected data were analyzed in SPSS software version 26. The baseline characteristics of subjects were expressed as mean ± SD continuous variables and percentage for qualitative variables. Since the sample size of the current study was small, we used nonparametric tests to analyze the data. The Mann-Whitney U test was performed to compare the quantitative data between the two groups, and the Wilcoxon test was conducted to compare the quantitative data pre-and post-treatment. The percentage of gender distribution between intervention and control groups was compared using the chi-square test. P-values < 0.05 were considered statistically significant.
4. Results
This study was conducted on 20 hospitalized patients with COVID-19 (65% men and 35% women) with a mean age of 53 ± 12 years old. The patients were divided into two groups of 10 based on receiving erythropoietin. The first group (intervention) received erythropoietin three times a day for four days, while the second group (control) received the same dose of the placebo. The para-clinical findings were examined and compared before and after the intervention.
Table 1 presents that the mean age of the patients is 53.4 ± 11.7 years in the intervention group and 52.9 ± 12.2 years in the control group, showing no significant difference (P = 0.700). The intervention group had 60% men and 40% women, while the control group had 70% men and 30% women (P = 0.210) (Table 1).
The Characteristics of Participants Based on Intervention and Control Groups Classification
Variables | Intervention Group (n = 10) | Control Group (n = 10) | P-Value a |
---|---|---|---|
Age (y) (mean ± SD) | 53.4 ± 11.7 | 52.9 ± 12.2 | 0.700 |
Sex (%) | 0.210 | ||
Men | 60 | 70 | |
Women | 40 | 30 |
According to Table 2, the mean hemoglobin level is 10.0 ± 1.1 g/dL in the intervention group and 8.0 ± 0.7 g/dL in the control group after treatment, showing a significant difference between the two groups (P = 0.040). In the intervention group, the mean hemoglobin level was 9.0 ± 0.8 g/dL before and 10.0 ± 1.1 g/dL after the treatment, indicating a significant increase (P = 0.001). In the control group, the mean hemoglobin level was 9.0 ± 0.8 g/dL before and 8.0 ± 0.7 g/dL g/dL after the treatment, showing a significant reduction (P = 0.002). The mean MCV was 73.0 ± 10.5 fL in the intervention group and 57.0 ± 6.0 fL in the control group after receiving the medication or placebo, indicating a significant difference (P = 0.017). In the intervention group, the mean MCV was 73.0 ± 11.1 fL before and 73.0 ± 10.5 after the treatment, but this difference was insignificant (P = 0.60). In the control group, the mean MCV was 68.0 ± 8.0 fL before and 57.0 ± 6.0 fL after the treatment, indicating a significant decrease (P = 0.002).
Hemoglobin and MCV Level Changes of Patients in Intervention and Control Groups During the Study Period
The mean hospital stay length (6 ± 2 days) in the intervention was significantly less group than in the control group (9 ± 4 days) (P = 0.001). At the end of the treatment, the mean LDH in the intervention group (503 ± 264 u/L) was significantly lower than the control group (725 ± 320 u/L) (P= 0.017) (Table 3).
The Change in Mean Hospital Stay Length and LDH Levels of Participants Based on Intervention and Control Groups During the Study Period a
Variables | Intervention Group (n = 10) | Control Group (n = 10) | P-Value b |
---|---|---|---|
Hospital stay length (day) | 6 ± 2 | 9 ± 4 | 0.001 |
LDH level (u/L) | 503 ± 264 | 725 ± 320 | 0.017 |
No side effect was reported for any patient during the hospital stay and the follow-up period due to recombinant erythropoietin or placebo injection, including thrombosis, deep vein thrombosis, coagulation disorders, myocardial infarction, CVA, or embolism.
5. Discussion
The purpose of this study was to examine the hemoglobin levels of patients with COVID-19 after they had received recombinant erythropoietin. The results demonstrated a significant increase in hemoglobin level of the intervention group after receiving recombinant erythropoietin compared to the pre-intervention level (P = 0.001). The comparison of mean hemoglobin levels in the two groups also revealed a significant difference after receiving erythropoietin and placebo (P = 0.04). As noted in the Introduction, the severity of COVID-19 may affect the reduction in hemoglobin levels (8, 10). Cai et al. examined the factors affecting COVID-19 ICU admission and found no relationship between hemoglobin level and ICU admission (17). Other studies have reported a relationship between COVID-19 progress-related reduced hemoglobin and the higher prevalence of anemia cases in deceased patients (18, 19). The comparison of hemoglobin pre- and post-placebo in the control group suggested a significant reduction in hemoglobin level (P = 0.002).
Almost all diseases are influenced by iron metabolism, which is essential for erythrocytosis and oxygen transport (20). Thus, the similarity in the distant amino acid sequence between ARS-CoV-2-spiked glycoprotein cytoplasmic tail and hepcidin protein, as well as the possibility of SARS-CoV-2 attacking the beta chain of hemoglobin could be due to hemoglobin reduction in these patients (21, 22). The results of Yagci et al. showed a significant decrease in hemoglobin and haptoglobin in critical and deceased COVID-19 patients (20), explaining the effectiveness of recombinant erythropoietin in the current study. The findings of Viruez-Soto et al. also demonstrated a 2.5-times lower mean erythropoietin level and a 25% reduction in hemoglobin level in deceased patients than in surviving patients (7). Erythropoietin is the primary growth factor that promotes red blood cell formation and tissue oxygenation (23), but it also has nonerythropoietic effects. Erythropoietin acts against vascular constriction, thereby improving oxygenation to the heart, brain, and other organs by increasing the endothelial capacity for producing nitric acid (24). COVID-19 also directly affects the respiratory centers of the brain stem, stimulates normoxic and hypoxic respiration, as well as preventing the inflammation caused by it (7, 24, 25). In addition, COVID-19 affects the retinoic acid-inducible gene I (RIG-I) and melanoma differentiation-associated gene 5 (MDA5) viral pattern recognition receptor that produces interferon and maintains vascular endothelium, which may reduce thrombotic events by activating innate immunity against virus RNAs through the beta-globin (26). As a result of these factors and the adjusted dose, erythropoietin injections may have had reduced side effects in our study.
The findings of post-recombinant erythropoietin injection in the intervention group did not show any significant difference in MCV compared to pre-intervention. However, the comparison between the control and erythropoietin groups showed a significant reduction in MCV in the control group (P = 0.017). In line with our findings, the results of Wang et al. demonstrated a significant decrease in the MCV of patients with a poor outcome compared to those with a good outcome (27). Taj et al. did not show any significant difference in the MCV of mild, moderate, and severe disease groups, which could be explained by the reduced statistical power due to the sample size (28). The MCV level was significantly lower post-placebo in the control group (P = 0.002), indicating the protective effect of the recombinant erythropoietin injected into the intervention group compared to the control group.
Moreover, the hospital stay length in the intervention was significantly less than in the control group (P = 0.001). To date, there has not been a study of erythropoietin administration in patients with COVID-19 to determine how long they are hospitalized, but studies have been conducted on patients with other diseases. Powe et al. found that the hospital stay length of patients undergoing dialysis from the first to the second nine-month period in the group receiving recombinant erythropoietin was less than in the control groups (29). Comparing the post-intervention LDH in the two groups indicated a significant decrease in LDH of the group receiving erythropoietin compared to the group receiving placebo (P = 0.017). In a meta-analysis by Ghahramani et al., LDH elevation in severe cases was introduced as a diagnostic factor for COVID-19 severity compared to non-severe cases (30). Zhang et al.’s meta-regression also mentioned LDH as a predictor of mortality, hospitalization, and acute respiratory distress syndrome (ARDS) (31). Several studies, as well as other studies, report LDH as a risk factor for increased severity (32-35). According to our findings, recombinant erythropoietin administration in the intervention group prevented the exacerbation of COVID-19, though its mechanism of action and possible effects on COVID-19 severity require further investigation.
This small sample size limited this study due to the multiplicity of exclusion criteria to avoid the side effects of erythropoietin, and the possible hazards associated with its administration. In addition, the information of the current study on some characteristics of participants, including body mass index, socioeconomic status, physical activity, and smoking, has not been determined, which could allow for more analysis and a more robust interpretation of the results.
5.1. Conclusions
The current study showed a high degree of confidence that recombinant erythropoietin had a positive effect on COVID-19 patients due to the random allocation of patients into the groups.
Acknowledgements
References
-
1.
Guan WJ, Ni ZY, Hu Y, Liang WH, Ou CQ, He JX, et al. Clinical Characteristics of Coronavirus Disease 2019 in China. N Engl J Med. 2020;382(18):1708-20. [PubMed ID: 32109013]. [PubMed Central ID: PMC7092819]. https://doi.org/10.1056/NEJMoa2002032.
-
2.
Park SE. Epidemiology, virology, and clinical features of severe acute respiratory syndrome -coronavirus-2 (SARS-CoV-2; Coronavirus Disease-19). Clin Exp Pediatr. 2020;63(4):119-24. [PubMed ID: 32252141]. [PubMed Central ID: PMC7170784]. https://doi.org/10.3345/cep.2020.00493.
-
3.
Wang Y, Wang Y, Chen Y, Qin Q. Unique epidemiological and clinical features of the emerging 2019 novel coronavirus pneumonia (COVID-19) implicate special control measures. J Med Virol. 2020;92(6):568-76. [PubMed ID: 32134116]. [PubMed Central ID: PMC7228347]. https://doi.org/10.1002/jmv.25748.
-
4.
Robinson PC, Liew DFL, Tanner HL, Grainger JR, Dwek RA, Reisler RB, et al. COVID-19 therapeutics: Challenges and directions for the future. Proc Natl Acad Sci U S A. 2022;119(15). e2119893119. [PubMed ID: 35385354]. https://doi.org/10.1073/pnas.2119893119.
-
5.
Lu H. Drug treatment options for the 2019-new coronavirus (2019-nCoV). Biosci Trends. 2020;14(1):69-71. [PubMed ID: 31996494]. https://doi.org/10.5582/bst.2020.01020.
-
6.
Zheng L, Zhang L, Huang J, Nandakumar KS, Liu S, Cheng K. Potential treatment methods targeting 2019-nCoV infection. Eur J Med Chem. 2020;205:112687. [PubMed ID: 32771797]. [PubMed Central ID: PMC7385720]. https://doi.org/10.1016/j.ejmech.2020.112687.
-
7.
Viruez-Soto A, Lopez-Davalos MM, Rada-Barrera G, Merino-Luna A, Molano-Franco D, Tinoco-Solorozano A, et al. Low serum erythropoietin levels are associated with fatal COVID-19 cases at 4,150 meters above sea level. Respir Physiol Neurobiol. 2021;292:103709. [PubMed ID: 34087493]. [PubMed Central ID: PMC8169280]. https://doi.org/10.1016/j.resp.2021.103709.
-
8.
Huang Y, Tu M, Wang S, Chen S, Zhou W, Chen D, et al. Clinical characteristics of laboratory confirmed positive cases of SARS-CoV-2 infection in Wuhan, China: A retrospective single center analysis. Travel Med Infect Dis. 2020;36:101606. [PubMed ID: 32114074]. [PubMed Central ID: PMC7102650]. https://doi.org/10.1016/j.tmaid.2020.101606.
-
9.
Wang L, Duan Y, Zhang W, Liang J, Xu J, Zhang Y, et al. Epidemiologic and Clinical Characteristics of 26 Cases of COVID-19 Arising from Patient-to-Patient Transmission in Liaocheng, China. Clin Epidemiol. 2020;12:387-91. [PubMed ID: 32308494]. [PubMed Central ID: PMC7154005]. https://doi.org/10.2147/CLEP.S249903.
-
10.
Xu T, Huang R, Zhu L, Wang J, Cheng J, Zhang B, et al. Epidemiological and clinical features of asymptomatic patients with SARS-CoV-2 infection. J Med Virol. 2020;92(10):1884-9. [PubMed ID: 32346873]. [PubMed Central ID: PMC7267538]. https://doi.org/10.1002/jmv.25944.
-
11.
Jelkmann W. Erythropoietin: structure, control of production, and function. Physiol Rev. 1992;72(2):449-89. [PubMed ID: 1557429]. https://doi.org/10.1152/physrev.1992.72.2.449.
-
12.
Ehrenreich H, Weissenborn K, Begemann M, Busch M, Vieta E, Miskowiak KW. Erythropoietin as candidate for supportive treatment of severe COVID-19. Mol Med. 2020;26(1):58. [PubMed ID: 32546125]. [PubMed Central ID: PMC7297268]. https://doi.org/10.1186/s10020-020-00186-y.
-
13.
Fishbane S, Hirsch JS. Erythropoiesis-Stimulating Agent Treatment in Patients With COVID-19. Am J Kidney Dis. 2020;76(3):303-5. [PubMed ID: 32479920]. [PubMed Central ID: PMC7256552]. https://doi.org/10.1053/j.ajkd.2020.05.002.
-
14.
Hadadi A, Mortezazadeh M, Kolahdouzan K, Alavian G. Does recombinant human erythropoietin administration in critically ill COVID-19 patients have miraculous therapeutic effects? J Med Virol. 2020;92(7):915-8. [PubMed ID: 32270515]. [PubMed Central ID: PMC7262240]. https://doi.org/10.1002/jmv.25839.
-
15.
Schulz KF, Altman DG, Moher D, Consort Group. CONSORT 2010 Statement: updated guidelines for reporting parallel group randomised trials. BMC Med. 2010;8:18. [PubMed ID: 20334633]. [PubMed Central ID: PMC2860339]. https://doi.org/10.1186/1741-7015-8-18.
-
16.
Samimagham HR, Azad MH, Hooshyar D, Haddad M, Arabi M, KazemiJahromi M. Evaluation of the efficacy and safety of recombinant erythropoietin on the improvement of hospitalised COVID-19 patients: A structured summary of a study protocol for a randomised controlled trial. Trials. 2021;22(1):435. [PubMed ID: 34229729]. [PubMed Central ID: PMC8258474]. https://doi.org/10.1186/s13063-021-05363-w.
-
17.
Cai SH, Liao W, Chen SW, Liu LL, Liu SY, Zheng ZD. Association between obesity and clinical prognosis in patients infected with SARS-CoV-2. Infect Dis Poverty. 2020;9(1):80. [PubMed ID: 32600411]. [PubMed Central ID: PMC7322704]. https://doi.org/10.1186/s40249-020-00703-5.
-
18.
Cen Y, Chen X, Shen Y, Zhang XH, Lei Y, Xu C, et al. Risk factors for disease progression in patients with mild to moderate coronavirus disease 2019-a multi-centre observational study. Clin Microbiol Infect. 2020;26(9):1242-7. [PubMed ID: 32526275]. [PubMed Central ID: PMC7280135]. https://doi.org/10.1016/j.cmi.2020.05.041.
-
19.
Giacomelli A, Ridolfo AL, Milazzo L, Oreni L, Bernacchia D, Siano M, et al. 30-day mortality in patients hospitalized with COVID-19 during the first wave of the Italian epidemic: A prospective cohort study. Pharmacol Res. 2020;158:104931. [PubMed ID: 32446978]. [PubMed Central ID: PMC7242199]. https://doi.org/10.1016/j.phrs.2020.104931.
-
20.
Yagci S, Serin E, Acicbe O, Zeren MI, Odabasi MS. The relationship between serum erythropoietin, hepcidin, and haptoglobin levels with disease severity and other biochemical values in patients with COVID-19. Int J Lab Hematol. 2021;43:142-51. [PubMed ID: 33554466]. [PubMed Central ID: PMC8014125]. https://doi.org/10.1111/ijlh.13479.
-
21.
Ehsani S. COVID-19 and iron dysregulation: distant sequence similarity between hepcidin and the novel coronavirus spike glycoprotein. Biol Direct. 2020;15(1):19. [PubMed ID: 33066821]. [PubMed Central ID: PMC7563913]. https://doi.org/10.1186/s13062-020-00275-2.
-
22.
Liu W, Li H. COVID-19 disease: ORF8 and surface glycoprotein inhibit heme metabolism by binding to porphyrin. ChemRxiv. 2020;Preprint. https://doi.org/10.26434/chemrxiv.11938173.v2.
-
23.
Jelkmann W. Erythropoietin after a century of research: younger than ever. Eur J Haematol. 2007;78(3):183-205. [PubMed ID: 17253966]. https://doi.org/10.1111/j.1600-0609.2007.00818.x.
-
24.
Beleslin-Cokic BB, Cokic VP, Wang L, Piknova B, Teng R, Schechter AN, et al. Erythropoietin and hypoxia increase erythropoietin receptor and nitric oxide levels in lung microvascular endothelial cells. Cytokine. 2011;54(2):129-35. [PubMed ID: 21324713]. [PubMed Central ID: PMC3074932]. https://doi.org/10.1016/j.cyto.2011.01.015.
-
25.
Wang M, Xiong H, Chen H, Li Q, Ruan XZ. Renal Injury by SARS-CoV-2 Infection: A Systematic Review. Kidney Dis (Basel). 2021;7(2):100-10. [PubMed ID: 33821207]. [PubMed Central ID: PMC7705946]. https://doi.org/10.1159/000512683.
-
26.
Brenner SR. Erythropoietin-induced hemoglobin subunit beta may stimulate innate immune RNA virus pattern recognition, suppress reactive oxygen species, reduce ACE2 viral doorway opening, and neutrophil extracellular traps against COVID-19. J Med Virol. 2021;93(1):180-1. [PubMed ID: 32644208]. [PubMed Central ID: PMC7361735]. https://doi.org/10.1002/jmv.26284.
-
27.
Wang C, Zhang H, Cao X, Deng R, Ye Y, Fu Z, et al. Red cell distribution width (RDW): a prognostic indicator of severe COVID-19. Ann Transl Med. 2020;8(19):1230. [PubMed ID: 33178762]. [PubMed Central ID: PMC7607068]. https://doi.org/10.21037/atm-20-6090.
-
28.
Taj S, Kashif A, Arzinda Fatima S, Imran S, Lone A, Ahmed Q. Role of hematological parameters in the stratification of COVID-19 disease severity. Ann Med Surg (Lond). 2021;62:68-72. [PubMed ID: 33437468]. [PubMed Central ID: PMC7792501]. https://doi.org/10.1016/j.amsu.2020.12.035.
-
29.
Powe NR, Griffiths RI, Watson AJ, Anderson GF, de Lissovoy G, Greer JW, et al. Effect of recombinant erythropoietin on hospital admissions, readmissions, length of stay, and costs of dialysis patients. J Am Soc Nephrol. 1994;4(7):1455-65. [PubMed ID: 8161727]. https://doi.org/10.1681/ASN.V471455.
-
30.
Ghahramani S, Tabrizi R, Lankarani KB, Kashani SMA, Rezaei S, Zeidi N, et al. Laboratory features of severe vs. non-severe COVID-19 patients in Asian populations: a systematic review and meta-analysis. Eur J Med Res. 2020;25(1):30. [PubMed ID: 32746929]. [PubMed Central ID: PMC7396942]. https://doi.org/10.1186/s40001-020-00432-3.
-
31.
Zhang JJY, Lee KS, Ang LW, Leo YS, Young BE. Risk Factors for Severe Disease and Efficacy of Treatment in Patients Infected With COVID-19: A Systematic Review, Meta-Analysis, and Meta-Regression Analysis. Clin Infect Dis. 2020;71(16):2199-206. [PubMed ID: 32407459]. [PubMed Central ID: PMC7239203]. https://doi.org/10.1093/cid/ciaa576.
-
32.
Terpos E, Ntanasis-Stathopoulos I, Elalamy I, Kastritis E, Sergentanis TN, Politou M, et al. Hematological findings and complications of COVID-19. Am J Hematol. 2020;95(7):834-47. [PubMed ID: 32282949]. [PubMed Central ID: PMC7262337]. https://doi.org/10.1002/ajh.25829.
-
33.
Zheng Z, Peng F, Xu B, Zhao J, Liu H, Peng J, et al. Risk factors of critical & mortal COVID-19 cases: A systematic literature review and meta-analysis. J Infect. 2020;81(2):e16-25. [PubMed ID: 32335169]. [PubMed Central ID: PMC7177098]. https://doi.org/10.1016/j.jinf.2020.04.021.
-
34.
Izcovich A, Ragusa MA, Tortosa F, Lavena Marzio MA, Agnoletti C, Bengolea A, et al. Prognostic factors for severity and mortality in patients infected with COVID-19: A systematic review. PLoS One. 2020;15(11). e0241955. [PubMed ID: 33201896]. [PubMed Central ID: PMC7671522]. https://doi.org/10.1371/journal.pone.0241955.
-
35.
Henry BM, Benoit SW, de Oliveira MHS, Hsieh WC, Benoit J, Ballout RA, et al. Laboratory abnormalities in children with mild and severe coronavirus disease 2019 (COVID-19): A pooled analysis and review. Clin Biochem. 2020;81:1-8. [PubMed ID: 32473151]. [PubMed Central ID: PMC7251358]. https://doi.org/10.1016/j.clinbiochem.2020.05.012.