Abstract
Background:
Gram-negative bacteria are the most common infectious bacteria and are life-threatening in burn patients. Phage therapy studies can help the hospital community eliminate these bacterial pathogens.Objectives:
This study aimed to evaluate the effects of the isolated bacteriophages from hospital wastewater against Gram-negative pathogenic bacteria and investigate their cytotoxicity on two human skin cell lines.Methods:
The bacterial strains were isolated from burn wound infections. Bacteriophages were isolated from the hospital wastewater treatment plant in Isfahan, Iran. Transmission electron microscopy, spot tests, and restriction enzyme digestion were considered for phage identification. Finally, the lactate dehydrogenase (LDH) and tetrazolium-based MTT assays were used to realize the cytotoxicity impacts of the isolated phages. The statistical analysis of cell viability was performed using a two-way analysis of variance (ANOVA).Results:
The results showed that among the isolated strains, P. aeruginosa strain NEG_RA1300 (GenBank accession number: MW845642) was sensitive to the isolated phage VbɸPA-1. The TEM results showed a possible viral taxonomy of VbɸPA-1 based on its morphology, which belonged to the Myoviridae (T4-like phages). The MTT and LDH experiments showed no cytotoxicity of VbɸPA-1 on two tested cell lines.Conclusions:
Based on the results, the inhibitory effect of isolated phage on P. aeruginosa isolated from burn wounds with no toxic effect on cell lines was very significant. This phage can also be an acceptable selection against burn wound infections.Keywords
Wound Infections P. aeruginosa Bacteriophage Skin Cell Line Cytotoxicity
1. Background
Bacteria play a significant role in the occurrence of wound infections (1, 2). The etiology underlying bacterial wound infections encompasses many mechanisms (3). Studies have suggested that up to 75% of fatalities resulting from burn injuries can be attributed to microbial infections (4). Microbial organisms possess the capacity to produce biofilms and commonly exhibit resistance to traditional antibiotic therapies (5). Plentifully, burn wounds have been observed to harbor Gram-negative infectious pathogens, including Pseudomonas aeruginosa, broad-spectrum β-lactam-producing bacteria, and Enterobacteriaceae, as reported in previous studies (6, 7). Recent studies have shown that the leading causes of death from burn infections are now antimicrobial-resistant (AR) organisms, including P. aeruginosa and Escherichia coli, a bacterium commonly found in the lower intestines of warm-blooded organisms, which has long been a focus of research due to its ability to cause both harmless and harmful infections (8-10). Most of the Gram-negative bacteria are opportunistic pathogens causing healthcare-associated infections (HAI) in burn patients (11). The bacteriophages exhibit site-specific localization within the infectious site, resulting in targeted lysis of bacterial cells. On the other hand, the administration of antibiotics is accompanied by systemic distribution in the body, leading to the possibility of a broad spectrum of adverse effects. There is limited prevalence of adverse events during or after administration of phage treatment. Post-antibiotic therapy, severe anaphylactic reactions, and secondary infections have been reported (12-14). In contemporary times, the scientific advancement of phage therapy has facilitated the utilization of bacteriophages as viable antibacterial agents for the resolution of bacterial ailments (15). The scholarly literature has documented evidence suggesting that bacteriophages possess the potential to curtail instances of bacterial infections among patients hospitalized with burn wounds (16, 17), particularly those stemming from antibiotic-resistant bacterial strains (18). In vitro cell lines are considered a cost-effective and convenient model for screening the interaction of phages, bacteria, and human cells (19, 20). Different phages bind or adsorb differently to the same human cell, whereas comparable phages bind or adsorb differently to distinct human cells in phage binding experiments. Identifying phages and their corresponding receptors on human cells elucidates the diagnostic patterns associated with human cells bound by phages (21). Finding a new phage from burn wounds in this study that has no toxic effect on cell lines can help plan new alternative drugs against burn wound infections caused by Pseudomonas aeruginosa.
2. Objectives
The current investigation seeks to identify bacteriophages capable of inhibiting Gram-negative bacterial infections. The safety of the isolated phages was assessed by investigating their effects on two types of human skin fibroblast-like cell lines: (1) human skin fibroblast-like cell A375, and (2) human skin melanoma-epithelial-like cell HFSF-PI 3. The results were evaluated through the implementation of MTT and lactate dehydrogenase (LDH) assays.
3. Methods
3.1. Isolation and Identification of Bacteria in Burn Wound Samples
Gram-negative isolates like P. aeruginosa and E. coli were collected from burn wounds of patients (March-June 2020) referred to in Isfahan (Iran) burn hospitals. The strains were cultured on EMB, McConkey, and Blood agar media (Merck, Germany) and examined for colony formation and morphological characterization (22). A commercial kit (RIBO-prep, AmpliSens Company, Russia) was used for bacterial DNA extraction. A PCR protocol amplified the 16S rRNA gene in isolated DNA. Universal primers 27F and 1492R were used (13). Bacterial strains were identified by analyzing gene sequences in a DNA sequencer system (Applied Biosystems, USA) and aligning them in GenBank using the BLAST server.
3.2. Isolation and Purification of the Phages
Samples were collected from the hospital wastewater treatment plant and stored at 4°C. After filtering, the samples were centrifuged at 8000 g for 30 minutes at 4°C using an ultracentrifuge (SIGMA.30 K3, Germany). The sample was filtered using 0.22 μm syringe filters (Cobetter, Japan) (23). The double-layer agar method was used. Then, 100 µL of each diluted phage suspension was added to the bacterial culture (OD = 0.3 at 620 nm) in melted BHI agar (45°C) in a tube. The mixture was then poured into a thin layer of BHI agar on a plate. The plates were solidified and incubated overnight at 37°C, then examined for plaques.
3.3. Identifying and Verifying Putative Plaques
For this purpose, an overnight culture was provided from each bacterial isolate on a BHI agar medium with 1.5% agar. Then, 10 μL of each dilution of phage filtrate (10-4 to 10-8) was spotted in an appropriate place on the agar, and the spots were allowed to absorb into the agar medium for 20 min. After incubating at 37°C for 24 hours, positive spot test results were recorded when complete bacteria obliteration was observed (24, 25).
3.4. Transmission Electron Microscopy for Phage Morphology Observation
The study used the Formvar technique to stain TEM grids with purified bacteriophage samples. TEM micrographs were used to observe the phage particles. Accordingly, 20 μL of the phage filtrate (108 PFU/mL) was placed on a carbon-coated copper grid. Next, the grids underwent staining with 2% (w/v) uranyl acetate for four minutes. A detailed analysis of the purified phage particles on the grids was conducted using a Philips EM 208S transmission electron microscope at the Parto Rayan Rastak Co. in Tehran, Iran. The International Committee on Taxonomy of Viruses (ICTV) guidelines are employed to classify the morphology of bacteriophages (26-28).
3.5. Extraction of Phage Genomic DNA
A DNA extraction kit (Norgen Biotek, Canada) was employed to purify the phage genomes. The mixture was filtrated using 0.22 μm syringe filters to obtain the extracted phage DNA as a precipitate. To mitigate the presence of protein residues, a solution of phenol/chloroform/isoamyl alcohol with a ratio of 25:24:1 (v/v/v) was employed. The precipitation of phage DNA was conducted using pure isopropanol (24, 28).
3.6. Restriction Enzyme Digestion Profile
To analyze the phage genome, 1 μg of each DNA was mixed with 1 μL each of HindIII, BamHI, ECORI, KpnI, HaeI, and XhoI obtained from Fementas (USA) in 1 μL of corresponding buffer. The mixture was incubated at 37°C for 16 hours. After extraction, the fragments were electrophoresed on a 0.7% agarose gel and visualized using a UV transilluminator (24, 29).
3.7. Cell Culture
The effects of the phage were evaluated on gram-negative antibiotic-resistant bacteria from burn wounds, as well as normal human skin fibroblast cell line (HFSF PI 3) and human skin epithelial tumor cells (A375) obtained from Pasteur Institute Cell Bank, Tehran, Iran. The cells were prepared in flasks and cultured in RPMI1640 and DMEM medium containing 10% FBS serum. All cells were incubated at 37°C in 5% CO₂ in a 98% humid atmosphere (29].
3.7.1. Evaluation of Plasma Lactate Dehydrogenase
The procedure was performed using the Darman Faraz Kave kit. At first, the amount of released LDH into the cell culture medium was considered. For this purpose, 50 µL of the test sample (cell culture supernatant) was added with 2 × 108 PFU/mL phage particles to a 96-well plate. Then, the sample was decolorized by adding 50 µL SM buffer and then incubated for 30min at room temperature (∼28°C). The reaction was stopped by adding 50 µL of the stop solution to each well. Finally, the absorbance of the wells was read at the wavelength of 340 nm at 1, 2, 3, and 4 min. The changes in absorption per min (ΔOD/min) were obtained from the average of the three ΔOD/min amounts. Lactate dehydrogenase activity (U/L) was calculated by multiplying ΔOD/min by the constant number 16238 (30).
3.7.2. MTT Assay
The MTT method was used to measure the cell’s viability. Each well was a 96-well plate which had 200 μL of the culture medium containing 10⁴ cells, and the wells were inoculated with 2 × 10⁸ PFU/mL phage particles after 4 h incubation at 37°C in the presence of 5% CO₂ and 98% humidity. The plate was placed in the CO₂ incubator for 24 h, and then 50 μL of MTT (5 mg/mL) was added to the wells and incubated for 3 h. The supernatant was removed, and 50 μL of DMSO was added to dissolve the formazan crystals. The solution was read at 570 nm using a microplate reader (Tecan, Switzerland) (31).
3.8. Statistical Analysis
The data output was presented as mean ± SEM. Two-way analysis of variance (ANOVA) was used for data analysis, and all data was entered into Microsoft Excel. SPSS version 20 and GraphPad Prism program (GraphPad Software) were used for analytical statistics.
4. Results
4.1. Bacterial Strain Characterization
P. aeruginosa strains were confirmed by biochemical tests comparing the test results for P. aeruginosa ATCC 10145 (Table 1). The molecular characterization and BLAST analysis showed that most bacterial strains had 100% identity. Among the isolates, P. aeruginosa NEG_RA1300 (GenBank accession number: MW845642) was deposited in NCBI GenBank as a novel strain.
Identification of Physical and Biochemical Characteristics of Strains
Genus and Species | Pseudomonas Aeruginosa | Pseudomonas Aeruginosa ATCC 10145 |
---|---|---|
Gram staining | Negative | Negative |
Shape | Rods | Rods |
TSIA | K/K/- | K/K/- |
Urease | Negative | Negative |
OF | Oxidative | Oxidative |
Motility | Motile | Motile |
VP | Negative | Negative |
MR | Negative | Negative |
Oxidase | Positive | Positive |
Catalase | Positive | Positive |
Citrate | Positive | Positive |
Indole | Negative | Negative |
Nitrate reduction | Positive | Positive |
Gelatin hydrolysis | Positive | Positive |
Gas | Positive | Positive |
Capsule | Encapsulated | Encapsulated |
Pigment | Positive | Positive |
The phylogenic tree of the studied strain is illustrated in Figure 1.
The phylogenetic tree indicating the studied P. aeruginosa strain
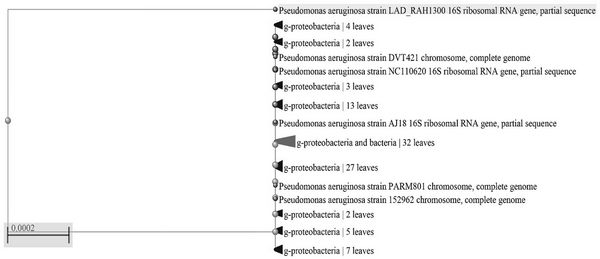
4.2. The Morphological Assessment of Isolated Phage
The phage VbɸPA-1 was isolated from the hospital wastewater treatment plant and visualized by the double-layered method and spot test. The phage plaque had small halos with a round center. The phages formed plaques on the bacterial culture, as shown in Figure 2. The morphology of phage VbɸPA-1, which was illustrated by TEM, revealed a circular head (range 90 ± 10 nm) with a flexible and screw tail (170 nm) in the phage, indicating that the phage belonged to the Myoviridae family (Figure 1).
Inhibition zone (A), and transmission electron micrographs (TEM) (B), of the specific phage for P. aeruginosa NEG_RA1300 isolated from hospital wastewater treatment plant, Isfahan, Iran. A possible viral taxonomy based on morphology is Myoviridae. Scale bars show 100 nm.
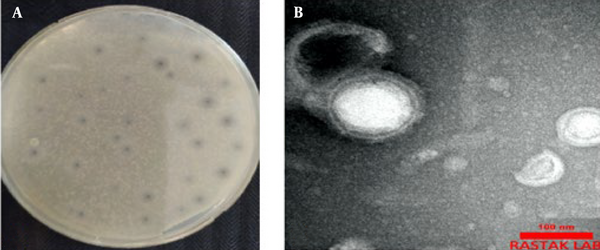
4.3. The Investigation of Phage Sensitivity
Based on Table 2, the results from host range identification based on the lytic activity of bacteriophages VbɸPA-1 on different clinical strains using the spot test showed that phage VbɸPA-1 created a strong transparent zone (++) on P. aeruginosa strain NEG_RA1300 and a transparent solid zone (+) on P. aeruginosa strain NEG_RA1301 and Pseudomonas aeruginosa strain LAD_RAH1300.
Spot Testing and Investigation of Phage Sensitivity
Bacterial Strains | Sensitivity to Phage | ||
---|---|---|---|
VbɸPA-1 a | Accession No. | Collection Source | |
Pseudomonas aeruginosa strain LAD_RAH1300 | + | MW845644 | Clinical samples; culture collection |
Pseudomonas aeruginosa strain NEG_RA1300 | ++ | MW845642 | Clinical samples; culture collection |
Pseudomonas aeruginosa strain NEG_RA1301 | + | MW845643 | Clinical samples; culture collection |
Escherichia coli strain ADB_66-1 | - | MW844043 | Clinical samples; culture collection |
Escherichia coli strain ADB_66-2 | - | MW844044 | Clinical samples; culture collection |
4.4. The Phage DNA Restriction Digestion Pattern
The outcomes of the restriction enzyme digestion profiles on the phage DNA are illustrated in Figure 3. The susceptibility of the phage DNA to HindIII, BamHI, and HaeIII enzymes was observed.
The restriction map of phage VbɸPA-1
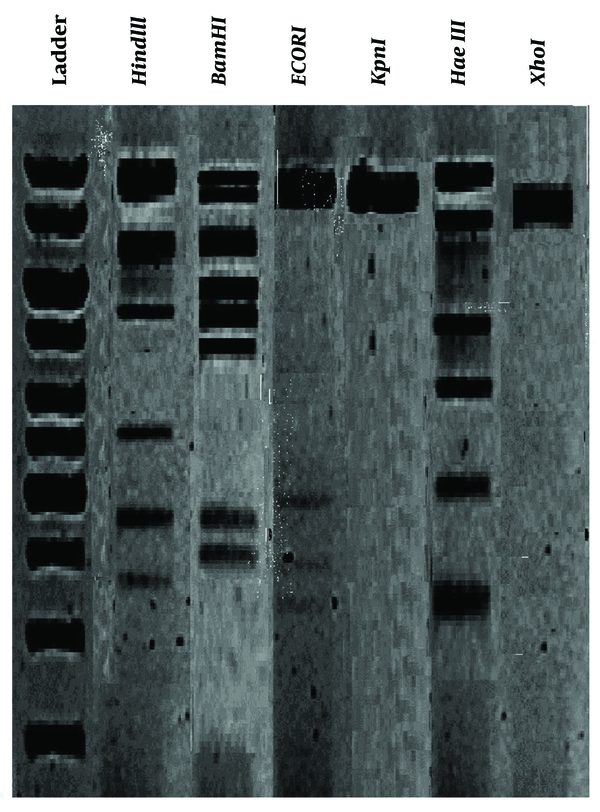
4.5. The Cell Viability Results of the Cells Exposed to the Isolated Phage
Lactate dehydrogenase value and MTT test results in HFSF PI3 and A375 cells were exposed to the phage VbɸPA-1 compared to the control. The concentration of the used phages was about 2 × 10⁸ PFU/mL. The results demonstrated that the phage VbɸPA-1 had no cytotoxic effect on either cell (Figure 4). The stars indicated a significant difference in the mean percentage of cytotoxicity with the control group (P < 0.001).
Lactate dehydrogenase (LDH) assay results for the analysis of HFSF PI3 (A); and A375 cells (B), viability following 24 to 72 h exposure to the phage VbɸPA-1 compared to the control. The data obtained by assessing OD at the wavelength of 490nm; P < 0.001, calculated as the mean ± SEM of three replicates results (A). Analysis of cell viability by MTT assay of HFSF PI3 and A375 cells exposed to the phage VbɸPA-1 compared to the control following 24 to 72h exposure. The data obtained by assessing OD at the wavelength of 570 nm; P < 0.001 and calculated as the mean ± SEM of 3 replicates results (B). *** sig. = 0.001.
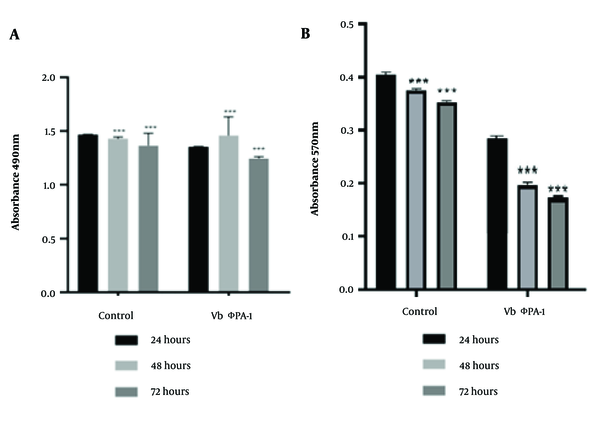
5. Discussion
The performance of cell culture as a robust tool can potentially arrange valid data on the non-toxicity evaluation of the phages. This study investigated the probable toxic effects of phages isolated from hospital wastewater on human cell cultures. The transmission electron microscopy outcomes indicated that the isolated phage, VbɸPA-1, had the morphologies of Myovirus. The impact of other bacteriophages on drug-resistant bacteria has been reported previously. For example, Rahimzadeh Torabi et al. showed that an ESBL-producing strain of Klebsiella pneumoniae was significantly sensitive to three phages, including PɸBw-Kp1, PɸBw-Kp2, and PɸBw-Kp3. The phage PɸBw-Kp3 was related to the Siphoviridae family, and the phages PɸBw-Kp1 and ɸBw-Kp2 were related to the Podoviridae family (T1-like phages) (24). Forti et al. investigated the efficiency of the phage cocktail against MDR pseudomonal strains. The cocktails destroyed the bacterial biofilms and treated acute respiratory infections in mice (32). These effects have also been shown in other infective drug-resistant Gram-negative bacteria, such as Acinetobacter baumannii (33).
Some researchers have used human cell culture models to investigate the interactions between bacteria and phages and their effects on the cells. Shan et al. examined Clostridium difficile to infect two tumor cell lines, HT-29 and Hela, and studied the cytotoxicity of phage phiCDHS1 on the cells. Based on the data obtained from the extent of LDH, phage phiCDHS1 had no cytotoxic effects on the studied cell lines. The findings also illustrated that the studied phage inhibited the specified bacteria (34). The results revealed that isolated phages had no significant toxicity impact on A375 and HFSF PI3 cell lines. Henein et al. also evaluated the cytotoxic effect of the phage 3T3 in mouse fibroblast 3T3 cells using four different assays. The phage concentrations up to 2 × 10⁹ PFU/mL showed little or no impact on the viability of 3T3 cells after 24h exposure. This study found that phage BS46 was non-cytotoxic, and their results were consistent with the present study (30). In agreement with other in vitro studies, a rapid decrease was observed in bacterial loads upon phage treatment at 24h. Shen et al. studied the phage ϕkm18p that ameliorated survival rates of human lung epithelial cells (A549) when infected with Acinetobacter baumannii. A high concentration (10⁶ CFU/mL) of bacteria was used to infect the cells and to analyze the protective efficacy of phages on the cells. The study showed that ϕkm18p increased the survival rate during 24h incubation to the same rate as the control. In addition, the phage ϕkm18p failed to affect the growth of A549 cells (33). In a study, Mendes et al. isolated a new bacteriophage cocktail with a therapeutic ability against organisms that cause diabetic foot infections (DFIs). The phage's antibacterial potential and wound healing properties were studied concerning bacterial infections caused by Staphylococcus aureus, P. aeruginosa, and A. baumannii, causing DFI. Morphological features showed that the phages belonged to the Myoviridae and Podoviridae families, while in our study, the bacteriophages belonged to the Plasmaviride and Myoviridae families (35). Pallavali et al. isolated and identified bacteriophages affecting MDR bacterial isolates isolated from septic ulcer infections. They isolated PA DP4 phage specific for P. aeruginosa. This study showed that phages had efficient antibacterial effects and are honest choices for prophylaxis against septic wounds (36).
The preliminary examination of the human skin cell model before conducting any in vivo experiments provides valuable insights into the safety profile of phages. The phage VbɸPA-1 strongly inhibited the growth of P. aeruginosa NEG_RA1300 from burn wounds, but the more valuable finding was the safety and non-toxic nature of the isolated phage in human skin cell lines. Additional clinical observations may be highly advantageous, mainly through in vivo experiments.
References
-
1.
Agnihotri N, Gupta V, Joshi RM. Aerobic bacterial isolates from burn wound infections and their antibiograms--a five-year study. Burns. 2004;30(3):241-3. [PubMed ID: 15082351]. https://doi.org/10.1016/j.burns.2003.11.010.
-
2.
Sharma S, Hans C. Bacterial infections in burns patients: a three years study at RML Hospital, Delhi. J Commun Dis. 1996;28(2):101-6. [PubMed ID: 8810144].
-
3.
Bowler PG, Duerden BI, Armstrong DG. Wound microbiology and associated approaches to wound management. Clin Microbiol Rev. 2001;14(2):244-69. [PubMed ID: 11292638]. [PubMed Central ID: PMC88973]. https://doi.org/10.1128/CMR.14.2.244-269.2001.
-
4.
Varshochi M, Hasani A, Pour Shahverdi P, Ravanbakhsh Ghavghani F, Matin S. Risk Factors for the Antibiotic Resistant Gram-Negative Bacilli Associated Infections in Burn Patients and the In-Vitro Susceptibility of Colistin. Arch Clinic Infect Dis. 2020;15(3). https://doi.org/10.5812/archcid.91174.
-
5.
Revathi G, Puri J, Jain BK. Bacteriology of burns. Burns. 1998;24(4):347-9. [PubMed ID: 9688200]. https://doi.org/10.1016/s0305-4179(98)00009-6.
-
6.
Church D, Elsayed S, Reid O, Winston B, Lindsay R. Burn wound infections. Clin Microbiol Rev. 2006;19(2):403-34. [PubMed ID: 16614255]. [PubMed Central ID: PMC1471990]. https://doi.org/10.1128/CMR.19.2.403-434.2006.
-
7.
Azzopardi EA, Azzopardi E, Camilleri L, Villapalos J, Boyce DE, Dziewulski P, et al. Gram negative wound infection in hospitalised adult burn patients--systematic review and metanalysis. PLoS One. 2014;9(4). e95042. [PubMed ID: 24751699]. [PubMed Central ID: PMC3994014]. https://doi.org/10.1371/journal.pone.0095042.
-
8.
Norbury W, Herndon DN, Tanksley J, Jeschke MG, Finnerty CC. Infection in Burns. Surg Infect (Larchmt). 2016;17(2):250-5. [PubMed ID: 26978531]. [PubMed Central ID: PMC4790211]. https://doi.org/10.1089/sur.2013.134.
-
9.
Hosseininassab Nodoushan SA, Yadegari S, Moghim S, Isfahani BN, Fazeli H, Poursina F, et al. Distribution of the Strains of Multidrug-resistant, Extensively Drug-resistant, and Pandrug-resistant Pseudomonas aeruginosa Isolates from Burn Patients. Adv Biomed Res. 2017;6:74. [PubMed ID: 28706882]. [PubMed Central ID: PMC5501067]. https://doi.org/10.4103/abr.abr_239_16.
-
10.
Williams FN, Herndon DN, Hawkins HK, Lee JO, Cox RA, Kulp GA, et al. The leading causes of death after burn injury in a single pediatric burn center. Crit Care. 2009;13(6):R183. [PubMed ID: 19919684]. [PubMed Central ID: PMC2811947]. https://doi.org/10.1186/cc8170.
-
11.
Lachiewicz AM, Hauck CG, Weber DJ, Cairns BA, van Duin D. Bacterial Infections After Burn Injuries: Impact of Multidrug Resistance. Clin Infect Dis. 2017;65(12):2130-6. [PubMed ID: 29194526]. [PubMed Central ID: PMC5850038]. https://doi.org/10.1093/cid/cix682.
-
12.
Salmond GP, Fineran PC. A century of the phage: past, present and future. Nat Rev Microbiol. 2015;13(12):777-86. [PubMed ID: 26548913]. https://doi.org/10.1038/nrmicro3564.
-
13.
Torabi LR, Naghavi NS, Doudi M, Monajemi R. Efficacious antibacterial potency of novel bacteriophages against ESBL-producing Klebsiella pneumoniae isolated from burn wound infections. Iran J Microbiol. 2021;13(5):678-90. [PubMed ID: 34900166]. [PubMed Central ID: PMC8629815]. https://doi.org/10.18502/ijm.v13i5.7435.
-
14.
Sulakvelidze A, Alavidze Z, Morris JJ. Bacteriophage therapy. Antimicrob Agents Chemother. 2001;45(3):649-59. [PubMed ID: 11181338]. [PubMed Central ID: PMC90351]. https://doi.org/10.1128/AAC.45.3.649-659.2001.
-
15.
McVay CS, Velasquez M, Fralick JA. Phage therapy of Pseudomonas aeruginosa infection in a mouse burn wound model. Antimicrob Agents Chemother. 2007;51(6):1934-8. [PubMed ID: 17387151]. [PubMed Central ID: PMC1891379]. https://doi.org/10.1128/AAC.01028-06.
-
16.
Soothill J. Use of bacteriophages in the treatment of Pseudomonas aeruginosa infections. Expert Rev Anti Infect Ther. 2013;11(9):909-15. [PubMed ID: 24053272]. https://doi.org/10.1586/14787210.2013.826990.
-
17.
Rose T, Verbeken G, Vos DD, Merabishvili M, Vaneechoutte M, Lavigne R, et al. Experimental phage therapy of burn wound infection: difficult first steps. Int J Burns Trauma. 2014;4(2):66-73. [PubMed ID: 25356373]. [PubMed Central ID: PMC4212884].
-
18.
Kumari S, Harjai K, Chhibber S. Bacteriophage Treatment of Burn Wound Infection Caused by Pseudomonas aeruginosa PAO in BALB/c Mice. America J Biomed Sci. 2009;1(4):385-94. https://doi.org/10.5099/aj090400385.
-
19.
Shan J, Ramachandran A, Thanki AM, Vukusic FBI, Barylski J, Clokie MRJ. Bacteriophages are more virulent to bacteria with human cells than they are in bacterial culture; insights from HT-29 cells. Sci Rep. 2018;8(1):5091. [PubMed ID: 29572482]. [PubMed Central ID: PMC5865146]. https://doi.org/10.1038/s41598-018-23418-y.
-
20.
Gorski A, Borysowski J, Miedzybrodzki R. Bacteriophage Interactions With Epithelial Cells: Therapeutic Implications. Front Microbiol. 2020;11:631161. [PubMed ID: 33537024]. [PubMed Central ID: PMC7848012]. https://doi.org/10.3389/fmicb.2020.631161.
-
21.
Barr JJ. A bacteriophages journey through the human body. Immunol Rev. 2017;279(1):106-22. [PubMed ID: 28856733]. https://doi.org/10.1111/imr.12565.
-
22.
Elston HR, Baudo JA, Stanek JP, Schaab M. Multi-biochemical test system for distinguishing enteric and other gram-negative bacilli. Appl Microbiol. 1971;22(3):408-14. [PubMed ID: 4940877]. [PubMed Central ID: PMC376323]. https://doi.org/10.1128/am.22.3.408-414.1971.
-
23.
Beheshti Maal K, Soleimani Delfan A, Salmanizadeh S. Isolation and Identification of Two Novel Escherichia coli Bacteriophages and Their Application in Wastewater Treatment and Coliform's Phage Therapy. Jundishapur J Microbiol. 2015;8(3). e14945. [PubMed ID: 25834715]. [PubMed Central ID: PMC4377173]. https://doi.org/10.5812/jjm.14945.
-
24.
Rahimzadeh Torabi L, Doudi M, Naghavi NS, Monajemi R. Isolation, characterization, and effectiveness of bacteriophage Pɸ-Bw-Ab against XDR Acinetobacter baumannii isolated from nosocomial burn wound infection. Iran J Basic Med Sci. 2021;24(9):1254-63. [PubMed ID: 35083013]. [PubMed Central ID: PMC8751751]. https://doi.org/10.22038/ijbms.2021.57772.12850.
-
25.
Mohammed-Ali MN, M. Jamalludeen N. Isolation and Characterization of Bacteriophage against Methicillin Resistant Staphylococcus aureus. J Med Microbiol Diagn. 2016;5(1). https://doi.org/10.4172/2161-0703.1000213.
-
26.
Ackermann HW. Basic phage electron microscopy. Methods Mol Biol. 2009;501:113-26. [PubMed ID: 19066816]. https://doi.org/10.1007/978-1-60327-164-6_12.
-
27.
Bradley DE. Ultrastructure of bacteriophage and bacteriocins. Bacteriol Rev. 1967;31(4):230-314. [PubMed ID: 4865539]. [PubMed Central ID: PMC408286]. https://doi.org/10.1128/br.31.4.230-314.1967.
-
28.
Krupovic M, Dutilh BE, Adriaenssens EM, Wittmann J, Vogensen FK, Sullivan MB, et al. Taxonomy of prokaryotic viruses: update from the ICTV bacterial and archaeal viruses subcommittee. Arch Virol. 2016;161(4):1095-9. [PubMed ID: 26733293]. https://doi.org/10.1007/s00705-015-2728-0.
-
29.
Ghasemi SM, Bouzari M, Shaykh Baygloo N, Chang HI. Insights into new bacteriophages of Lactococcus garvieae belonging to the family Podoviridae. Arch Virol. 2014;159(11):2909-15. [PubMed ID: 24928734]. https://doi.org/10.1007/s00705-014-2142-z.
-
30.
Henein AE, Hanlon GW, Cooper CJ, Denyer SP, Maillard JY. A Partially Purified Acinetobacter baumannii Phage Preparation Exhibits no Cytotoxicity in 3T3 Mouse Fibroblast Cells. Front Microbiol. 2016;7:1198. [PubMed ID: 27536286]. [PubMed Central ID: PMC4971803]. https://doi.org/10.3389/fmicb.2016.01198.
-
31.
Mosmann T. Rapid colorimetric assay for cellular growth and survival: application to proliferation and cytotoxicity assays. J Immunol Methods. 1983;65(1-2):55-63. [PubMed ID: 6606682]. https://doi.org/10.1016/0022-1759(83)90303-4.
-
32.
Forti F, Roach DR, Cafora M, Pasini ME, Horner DS, Fiscarelli EV, et al. Design of a Broad-Range Bacteriophage Cocktail That Reduces Pseudomonas aeruginosa Biofilms and Treats Acute Infections in Two Animal Models. Antimicrob Agents Chemother. 2018;62(6). [PubMed ID: 29555626]. [PubMed Central ID: PMC5971607]. https://doi.org/10.1128/AAC.02573-17.
-
33.
Shen GH, Wang JL, Wen FS, Chang KM, Kuo CF, Lin CH, et al. Isolation and characterization of phikm18p, a novel lytic phage with therapeutic potential against extensively drug resistant Acinetobacter baumannii. PLoS One. 2012;7(10). e46537. [PubMed ID: 23071586]. [PubMed Central ID: PMC3465330]. https://doi.org/10.1371/journal.pone.0046537.
-
34.
Shan J, Ramachandran A, Thanki AM, Vukusic FBI, Barylski J, Clokie MRJ. Bacteriophages are more virulent to bacteria with human cells than they are in bacterial culture; insights from HT-29 cells. Scientific Rep. 2018;8(1). https://doi.org/10.1038/s41598-018-23418-y.
-
35.
Mendes JJ, Leandro C, Corte-Real S, Barbosa R, Cavaco-Silva P, Melo-Cristino J, et al. Wound healing potential of topical bacteriophage therapy on diabetic cutaneous wounds. Wound Repair Regen. 2013;21(4):595-603. [PubMed ID: 23755910]. https://doi.org/10.1111/wrr.12056.
-
36.
Pallavali RR, Degati VL, Lomada D, Reddy MC, Durbaka VRP. Isolation and in vitro evaluation of bacteriophages against MDR-bacterial isolates from septic wound infections. PLoS One. 2017;12(7). e0179245. [PubMed ID: 28719657]. [PubMed Central ID: PMC5515400]. https://doi.org/10.1371/journal.pone.0179245.