Abstract
Context:
Active packaging (AP) is an innovative approach employed in the food industry with the objective of satisfying consumers' elevated expectations concerning the safety, nutritional value, and overall excellence of food products.Objectives:
Various methods and technologies are used to control the release of active agents. Biodegradable polymers are, at present, a viable option for replacing non-biodegradable plastics in AP.Results:
Nanotechnology has been used to improve the functionality and storage shelf life of food packaging systems. This integration uses metal nanoparticles, nanoemulsions, and biomaterials to create smart packaging systems with antibacterial properties, indicator capabilities, and degradation. This aligns with the clean-label trend in the food industry, where consumers look for products with fewer ingredients and natural production methods. The use of AP technology extends product shelf-life by using absorption and diffusion systems for carbon dioxide, oxygen, and ethanol.Conclusions:
This manuscript reviews the latest advancements in advanced packaging technologies for the food industry. This text presents a detailed analysis of cutting-edge innovations in AP techniques, including scavengers, diffusion systems, and antimicrobial packaging.Keywords
Active Packaging Food Antimicrobial Packaging Nanotechnology in Packaging
1. Context
Packaging plays a vital role in the preservation of food items, as it enables their exportation, storage, and maintenance of quality for consumption. The societal shift toward a healthier lifestyle has resulted in an increased demand for food products that are of high quality, unprocessed, and easily consumable. Moreover, these products must be devoid of any contamination and possess an extended shelf life. Contemporary packaging techniques are imperative in safeguarding against alterations in color, formation of taste and smell, depletion of nutrients, changes in texture, and other detrimental impacts (1). There are different classifications available for modern packages capable of responding to and altering the state of the package. One commonly used categorization encompasses mechanical packaging that incorporates self-warming and self-refrigerating components; active packaging (AP) that utilizes absorbing substances, discharging agents, and antimicrobial agents; and finally, intelligent packaging that incorporates Radio frequency identification(RFID), identifiers, and biosensors (2).
Active packaging is a contemporary and cutting-edge method to prolong the duration of food preservation and enhance its overall quality by means of intentional engagements with the food itself or its immediate environment. Active packaging effectively regulates multiple factors that exert an impact on the longevity of food, encompassing physiological, chemical, physical, and microbiological processes (3).
Active packaging improves its performance by incorporating specialized components into the packaging material or its basic elements. The purpose of AP is to enhance the functionality of packaging by being situated in the space above the packaging itself, ultimately leading to an improvement in the collection process. Active packaging refers to packaging that intentionally includes additives to improve its performance. These additives have the capability to absorb or release oxygen, ethylene, carbon dioxide, and flavors and control humidity. Moisture can have a significant impact on the texture, appearance, and microbial activity of food, thereby altering its quality. In the AP sector, commonly employed technologies include absorbers (e.g., gas absorbers and diffusers) that contain preservatives, flavorings, antimicrobial substances, and more (4-6). With the growing level of scrutiny toward AP technologies in the realm of food, the objective of this examination is to thoroughly assess the most recent developments in this field. The aim is to enhance the uniformity of products and offer increased advantages to consumers. This inquiry delves into the intricacies of AP techniques (e.g., scavengers, diffusion systems, and antimicrobial packaging) to enhance the technological aspects of food packaging.
2. Types of Active Packaging
2.1. Absorbers
2.1.1. Oxygen Absorbers
Oxygen causes oxidation, decreasing food quality and storage time while promoting aerobic microorganism growth. Oxygen absorbers, introduced by Mitsubishi Gas Chemical Company in 1970, attract and remove oxygen from packages during storage (7, 8). They can also reduce trapped oxygen prior to sealing. The oxygen level in the package's headspace can be reduced to 0; however, lowering it to 5-2% v/v using modified vacuum packaging techniques might be harmful. Oxygen absorbers can decrease oxygen levels below 0.1% v/v, increasing shelf life (9). In 2018, Marangoni JL et al. examined the impact of packaging systems and materials on carotenoid-rich dehydrated potato chips. They employed different controlled packaging systems, such biaxially oriented polypropylene (BOPP) and met BOPP, with and without oxygen absorbers and nitrogen gas, to maintain the quality of sweet potato chips. Chips without nitrogen lasted 153 days, lost 6% beta-carotene, and had taste, odor, and sensory changes. Nitrogen-packaged chips lasted 184 days but had crispness issues. Chips packed with nitrogen and an oxygen scavenger retained 80-90% of β-carotene for 207 days with no sensory changes (10).
Iron powder bags are effective oxygen absorbers, as activated iron oxide reduces internal oxygen levels below 0. 5% success rate (12 out of 100) (11). Faas eta l. developed a palladium-based coating to prevent lipid oxidation in linseed oil. This technique, involving absorbent coating and a modified atmosphere, greatly reduced lipid oxidation in linseed oil, surpassing traditional packaging (12). Maxis and Kontominas tested low-density polyethylene (LDPE) and polyethylene terephthalate (PET) coated with LDPE on dark chocolate with oxygen absorbent under N2 or vacuum conditions at 20°C for 12 months. The chocolate package with oxygen absorption had a consistently low peroxide index after 12 months, regardless of packaging permeability (7). Tocopherol, ascorbic acid, microorganisms, and enzymes have limited absorption and cleaning abilities.
Oxygen absorbers prevent mold growth by removing excess oxygen and are commonly used in food products. Table 1 lists oxygen absorbers with unlimited shelf life (9). Natural and biological oxygen scavengers have advantages over chemical ones, including recycling, safety, material compatibility, and lower production costs. They use biocatalysts, such as glucose oxidase, catalase combination, or immobilized aerobic microorganisms to consume oxygen. A declaration has been issued on using endosperm bacteria as an oxygen scavenger in PET material. The substance absorbs moisture for spore germination activation (13).
Some Commercial Absorbents of Mitsubishi Gas Company of America
Type | Property | Application |
---|---|---|
E | Oxygen and carbon dioxide absorber | Coffee |
ZPT | Standard ion type | Wide variety, such as beef, baked goods, pet food, and snacks |
SS | Quick reaction type | Baked goods and frozen foods |
GT | Type of oxygen absorber and emission of a small amount | Products that are sensitive to shrinkage |
Z | Type of oxygen absorbers | Application in a wide range of foods, such as beef and bread |
FX | Type of oxygen absorbers | Pasta |
S | Type of oxygen absorbers | Cake and bakery |
G | Type of oxygen absorber and carbon dioxide emitter | Nuts, cakes, and bakery |
2.1.2. Ethylene Absorber Scavengers
Ethylene has a major impact on fruits and vegetables, affecting their characteristics, growth, maturation, and senescence. To boost the shelf life of products and control C2H2 gas during packaging, specific C2H2 absorbers have been created to absorb the ethylene emitted by fresh produce (14). Stoichiometric oxidizing systems, with potassium permanganate, control ethylene in packaging and prolong product lifespan. Ethylene absorbers capture ethylene from fresh goods. To be effective, KMnO4 must be adsorbed onto a carrier with a large surface area, such as celite, vermiculite, silica gel, alumina pellets, activated carbon, perlite, or glass. In certain absorbent systems, this compound is immobilized on alumina or silica gel to capture ethylene efficiently. Ethylene oxidation using potassium permanganate involves a two-step procedure (see Figure 1). First, ethylene is oxidized to acetaldehyde, which is then further oxidized to acetic acid. Acetic acid can later oxidize to form water and carbon dioxide (14, 15). Another ethylene absorber uses activated carbon and a metal catalyst to decompose ethylene. Palladium chloride-infused charcoal acts as an oxidizing agent, slowing fruit ripening and generating acetaldehyde (16). Table 2 shows highly absorbent materials used in food packaging.
Oxidation of ethylene with potassium permanganate
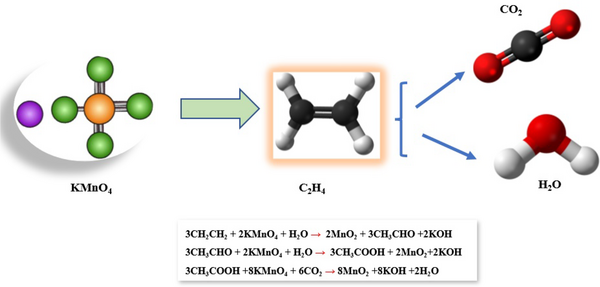
Some Absorbent Materials Used for Food Packaging
Type | Ethylene Absorbent Material |
---|---|
Natural clays | Zeolite, halloysite nanotube, Japanese Oya stone, cristobalite, bentonite, coral, and ceramic |
Regenerable absorbents | Propylene glycol, hexylene glycol, squalene, technical methyl silicone, polyethylene, and polystyrene |
Catalytic oxidizer | Potassium permanganate, potassium dichromate, iodine pentoxide, silver nitrate, and palladium |
2.1.3. Moisture Absorber
High humidity causes food spoilage via mold and bacteria growth. Effective packaging with strong water vapor barriers prevents this process. The study will explore the use of sorbitol and fructose as natural humectants in a polymer matrix for absorbing liquids from food or condensation, which is suitable for tray and cover packaging (17, 18). Fructose, a hygroscopic monosaccharide, absorbs moisture at 55% humidity (19). Organic materials are pricier and have lower moisture absorption than mineral absorbents, resulting in the requirement of bigger sachets for silica gel desiccants. Further research is needed to improve organic moisture absorbents' absorption, commercialization, and stability (1, 18, 20).
Cellulose is a natural organic macromolecule that absorbs moisture and is used for food packaging films. Cellulose absorbs moisture. Carboxymethyl cellulose (CMC) is popular in AP. Due to their film-forming properties and coverage, CMC, a polar macromolecule, absorbs moisture based on humidity and polar groups. Cellulose is ideal for moisture absorption and gains popularity in AP; however, minerals, such as calcium oxide and silica gel, are commonly used for this purpose in industrial manufacturing (19, 21). They are commonly used in sachets to control packaging humidity. Inorganic substances (e.g., potassium carbonate, potassium chloride, bentonite, and calcium chloride) are frequently used as moisture absorbents in food packaging. Suitable sachets for food applications in the market include MiniPax, FreshPax® StripPax, Natrasorb, Desi Pak, Sorb-It, 2-in-1, and Ageless. Ltd, (India) and (Shenzhen Chunwang, China) (18). Key parameters are vital for designing moisture-absorbent sachets, including storage temperature, absorption capacity, and humidity range, to prevent microbial activity. Sachet packaging must be leak-proof, durable, and with low water permeability. Some absorbent bags can change the sensory characteristics of food. Further research is needed to develop new sachet materials that meet the requirements (22). as customers might not realize they are consuming absorbent materials.
To prevent temperature changes during transport and storage, moisture absorber pads must be used to soak up liquid from food in trays, avoid altering its sensory qualities, and promote harmful bacteria growth (23, 24). Superabsorbent polymer powders and paper pads are used in pads. The polymer absorbs up to 500 times its weight in water, depending on salt concentration. Figure 2 shows moisture-absorbent pads for food packaging. Multifunctional absorbents reduce pH, have antimicrobial properties, and absorb odors. Exudates counter migration when compressed by food weight. Determining the ideal puncture distance is crucial to prevent tearing and material leakage, which adds another important limitation (4, 25). A new method for humidity control in food packaging includes using moisture-absorbing material in polymer trays, such as Tenderpac®, Fresh-R-Pax®, and Ultra-Fresh System (18).
The structure of the moisture-absorbing pad
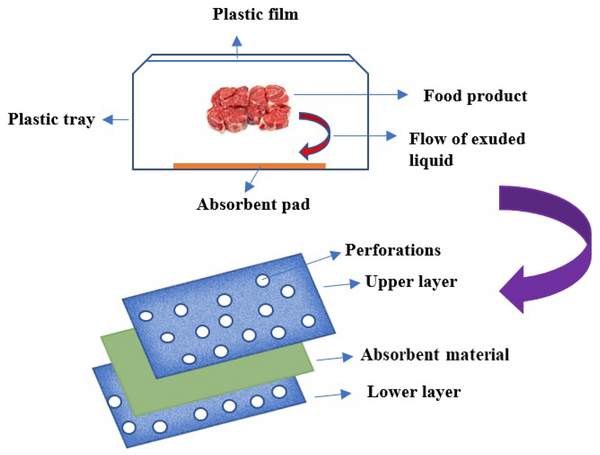
Food moisture can be managed with packaging materials containing sorbitol, glycerin, magnesium chloride, and sodium chloride, improving shelf life by reducing water condensation and stabilizing humidity. This is achieved by dispersing packaging materials into polymers. Packing material production must consider salt solution volume for crystallization. Sangerlaub et al. observed that polymeric films require a porous structure to store absorbed salty water solution from vapor absorption (26). Stretching foamed polypropylene with dispersed salt increases film porosity and diffusion coefficient, improving its water vapor absorption ability (27). A study examined the impact of thermoforming on the absorption and speed of polypropylene monolayers with NaCl dispersion. Results revealed increased porosity in the packaging material by 0.28 and 0.63 mm after thermoforming. Thermoforming is proven suitable for adjusting water vapor absorption of salt-dispersed polypropylene film for humidity regulation. Further research is needed for multilayered films with foaming polymer layers and dissolved salts. Moisture-absorbing salts can be added to a polymeric matrix during hot melt extrusion. However, humidity-regulating films are not much more effective than sachets in terms of capacity and water absorption rate. One concern is the moisture-absorbing materials migrating into food packaging. Addressing the challenge of obtaining sufficient absorbent materials for uniform distribution on packaging films is crucial (28).
2.2. Emitters or Diffusion Systems
Emitter systems in food packaging have many benefits, including improved processes, increased shelf life, and the release of beneficial gases for the food. Emitters or diffusion systems release gases into the package to improve food quality, prevent spoilage, and extend shelf life. Common gas emitters are ethanol, sulfur dioxide, and CO2.
2.2.1. Carbon Dioxide Emitters
Carbon dioxide (CO2) improves food freshness, reduces odors, and is commonly used in the food industry to extend shelf life. High CO2 levels are preferred to inhibit microorganism growth, particularly for fresh meat, fish, poultry, and non-climacteric fruits. In 2016, Hansen et al. discovered multiple mechanisms that delay growth onset. Using modified atmosphere packaging (MAP) technology and CO2 emitters to preserve cod loin and maintain its quality. Emitters are made from a liquid pad that absorbs a solution with 0. Moreover, 237 g citric acid and 0.304 g NAHCO3 are added to the food and pad. CO2 emitters in Modified Atmosphere packaging reduce CO2 by absorbing it from fish. They also increase gas pressure to preserve headspace, which is ideal for vacuum or MAP Packaging improves cod loin preservation with sodium bicarbonate and citric acid, releasing CO2 when in contact with liquid (29, 30). The system operates in accordance with Le Chatelier's principle, which dictates that the introduction of liquid into the system will result in a decrease in pH and initiate the generation of carbon dioxide. The system can be customized to fit specific food product requirements, including dimensions, packing area, gas-to-product volume (g/p) ratio, gas composition, and plastic properties. Activated packaging employs a CO2 release system containing FeCO3 content. These emitters release CO2 by utilizing FeCO3's solubility in acidic conditions (4, 31).
2.2.2. Ethanol Emitter
Ethanol (C2H6O) is used as an antimicrobial agent in packaging to prevent microorganism growth during handling, storage, and sale of produce, preserving quality and shelf life. It also prolongs shelf life and prevents decay of mangoes, wheat bread, and bayberry. Silica removes ethanol through adsorption (32). Ethanol in emission devices is a volatile gas. Concerns regarding taste and consumer hesitancy are addressed by companies such as Anti-mold 102™, EthiCap™, Oitech™, and Negamold™, which offer effective mold prevention solutions. Ltd and Ageless SE produce films and sachets with a barrier capacity of less than 2 g, releasing ethanol efficiently. Rangaraj V et al. reduce daily m-2 packaging material usage to overcome the limitations of traditional ethanol emitters (33). In 2017, researchers created a controlled-release ethanol emitter by combining sodium stearate with ethanol and heating it to 70°C. A translucent gel was created by adding diatomite to enhance its strength and usability. White powder ethanol emitters were created by cooling the final product, resulting in stronger bonds between ethanol and sodium stearate due to higher sodium strain. Sodium stearate is soluble in heated ethanol and regulates the expulsion of ethanol in the form of gel, thereby diminishing the rate of deterioration, conserving solidity, and restraining the elevated malondialdehyde concentration in the packaging of Chinese bayberry. Nonetheless, additional investigation is necessary to comprehend the mechanisms of preservation, impacts on alternative agricultural products, and the influence of environmental circumstances. (34).
2.2.3. Sulfur Dioxide Emitter
Sulfur dioxide (SO2) prevents chromatic degradation in stored farm products, such as walnuts, coconuts, tomatoes, peppers, beans, cabbage, pumpkins, carrots, pulses, and potatoes. Its effectiveness relies on metabisulfite hydrolysis and calcium sulfite reaction with moisture (35). During processing or preservation, sulfites are often used to prevent browning. They are considered significant allergens. The text discusses polyphenol oxidase's ability to prevent decay and the growth of fungi and bacteria that cause malolactic fermentation. The Codex Alimentarius regulates the use of SO2 in food, requiring any concentration exceeding 10 mg L-1 to be labeled as an allergen. Limited scientific studies have examined the effects of sulfites on health, such as urinary tract disease, dermatitis, hypotension, and stomach pain, in relation to their use in food preservation and shelf-life extension (36).
2.3. Antioxidant Release
Antioxidants prevent lipid oxidation in films or coatings. Gemili et al. collaborated in 2010 to create cellulose acetate films with different structures. This allowed them to control the release of natural antioxidants, such as L-ascorbic acid and L-tyrosine (37). Higher cellulose acetate concentration lowers antioxidant diffusion, resulting in smaller particles and less porosity. The AP industry commonly uses synthetic antioxidants, such as Butylated hydroxyanisole (BHA) and butylated hydroxytoluene (BHT) , with BHA being observed to have carcinogenic properties by the US Department of Health and Human Services. It is suggested that safe alternative antioxidants be used instead (38). More individuals prefer natural, additive-free food for health reasons. Eco-friendly antioxidants might be a safer alternative to synthetic ones. Researchers are examining antioxidants in biopolymeric food films (39, 40). Some antioxidants have stability, oxidation, and volatility challenges in packaging. Further research is needed to overcome these obstacles, as some antioxidants also have strong odors that hinder their use in food systems (41, 42).
3. Antimicrobial Packaging
Food packaging prevents microbial growth, prolonging shelf life by releasing agents that inhibit bacteria and fungi. Agents absorbed into food can lead to the loss of certain activities when they react with lipids and proteins. The proposal suggests using three-layer films to address concerns. The inner layer controls compound spread; the middle layer contains antimicrobial agents. The outer layers trap agents; however, the antibacterial film should not affect sensory attributes (43). Commonly used substances in antimicrobial systems are silver, silver zeolite, glucose oxidase, triclosan zeolite, natamycin, chlorine dioxide, and allyl isothiocyanate. Other antimicrobial metals include gold, copper, titanium dioxide, and zinc oxide (44). Metal nanoparticles exhibit superior antimicrobial effectiveness than natural antibacterial. Filtration, light irradiation, and other factors impact film performance against microbes. Additionally, technology, economics, and regulations must be considered for nanomaterials in food contact applications. Manufacturing of these packaging materials has been industrialized to assess their impact on food products. Botanical sources have potent antimicrobial properties, making them excellent additives in food packaging with great potential (45).
In 2020, Felix de Andrade et al. used orange essential oil to combat Escherichia coli (E. coli) growth in a butylene adipate-co-terephthalate butylene adipate-co-terephthalate (PBAT) film. The orange oil reduced E. coli growth significantly (46). The challenge with essential oils for antimicrobial use is their high concentrations, which can affect the sensory qualities of food. Safe concentrations in food packaging are crucial, requiring the development of antimicrobial films for food applications. Despite challenges in evaluating the antibacterial properties of films (e.g., film type and extract composition), considering these factors is crucial. Essential oils' susceptibility to evaporation reduces their antimicrobial effectiveness in active films. Including essential oils in packaging affects flavor and odor and requires careful consideration. Further research is needed to develop effective antimicrobial oils without impacting temperature, scent, or color (Maisanaba et al.) (45). In 2017, a study explored essential oils for AP for food preservation. Proteins and peptides, such as lactoferrin and nisin, enzymes, such as lysozyme, and polysaccharides, such as chitosan, show potential as antimicrobial agents in the food industry (47). Research teams have developed antimicrobial films for meat packaging using a polypeptide antibacterial agent to fight against spoilage and illness caused by microorganisms. New studies suggest that combining nisin with other preservatives is effective in food packaging (48).
In 2013, McArdle et al. observed that AP delays oxidation from high-pressure processing, increasing chicken meat shelf life (49). Chitosan is safe and eco-friendly for food packaging; however, its limited properties limit its use in the food industry. To address these concerns, scholars studied chitosan's bioactive components. In 2019, a chitosan and PA packaging film was created with antioxidant and antimicrobial properties. Adding polyhydroxyl groups boosted the film's antioxidant capacity, mainly due to proanthocyanidins (PA) improved effect (50). The film was tested against Salmonella, E. coli, Listeria monocytogenes, and Staphylococcus aureus for antimicrobial efficacy. Heterocyclic in PA improved antimicrobial ability, binding to bacterial cell wall polymers and inhibiting synthesis. The results showed that 20 wt% chitosan and proanthocyanidins (CS-PA ) film had better antimicrobial activity. Phenolic constituents enhance film's properties by interacting with chitosan and have antioxidant and antimicrobial qualities. PA improves chitosan film's oxygen-blocking ability. This was achieved by bonding hydrogen with chitosan chains in a study by Wang et al. In a 2015 study, researchers investigated nisin-loaded chitosan/poly (L-lactic acid) antimicrobial film production using a coating technique. These films effectively released nisin, reducing Staphylococcus aureus, the potential for use in food packaging, but not properly evaluated on edible items (47). To keep active ingredient levels in AP stable, regulated discharge is crucial. Options include multilayer films, bio-polymers, cross-linking, encapsulation, and fatty acid addition. Fatty acids improve zein films' flexibility, processability, and water resistance. Oleic acid affects antimicrobial properties based on film composition and structure (51).
4. Conclusions
The utilization of AP technology in packaging has experienced growth as a result of the escalating demand for food quality surveillance and prolonged product shelf-life. Active packaging methodologies amplify the safety of food and extend the duration of its shelf-life. Technologies derived from antimicrobial peptides (APs) act as safeguards for the quality of food and augment its safety. The task at hand lies in the development of functional materials that uphold their distinctive attributes throughout the course of distribution and storage. Supplementary hindrances within mass production procedures, the absence of legal provisions, and the reception from consumers necessitate a greater concentration from researchers toward the technological predicaments that are vital for the commercialization of these techniques in food packaging applications. Researchers are focusing more on antimicrobial systems and their impact on food's sensory attributes. It is important to study the residues of antimicrobial agents in packaged food and packaging headspace. This study can improve the safety and acceptability of these systems. In short, continuous research and focused improvements to reduce costs and complexities will greatly increase the use of these technologies in the food industry.
References
-
1.
Soltani Firouz M, Mohi-Alden K, Omid M. A critical review on intelligent and active packaging in the food industry: Research and development. Food Res Int. 2021;141:110113. [PubMed ID: 33641980]. https://doi.org/10.1016/j.foodres.2021.110113.
-
2.
Han JW, Ruiz-Garcia L, Qian JP, Yang XT. Food Packaging: A Comprehensive Review and Future Trends. Compr Rev Food Sci Food Saf. 2018;17(4):860-77. [PubMed ID: 33350114]. https://doi.org/10.1111/1541-4337.12343.
-
3.
Wyrwa J, Barska A. Innovations in the food packaging market: active packaging. European Food Research and Technology. 2017;243(10):1681-92. https://doi.org/10.1007/s00217-017-2878-2.
-
4.
Yildirim S, Rocker B, Pettersen MK, Nilsen-Nygaard J, Ayhan Z, Rutkaite R, et al. Active Packaging Applications for Food. Compr Rev Food Sci Food Saf. 2018;17(1):165-99. [PubMed ID: 33350066]. https://doi.org/10.1111/1541-4337.12322.
-
5.
Amin U, Khan MKI, Maan AA, Nazir A, Riaz S, Khan MU, et al. Biodegradable active, intelligent, and smart packaging materials for food applications. Food Packaging and Shelf Life. 2022;33. https://doi.org/10.1016/j.fpsl.2022.100903.
-
6.
Sani MA, Azizi-Lalabadi M, Tavassoli M, Mohammadi K, McClements DJ. Recent Advances in the Development of Smart and Active Biodegradable Packaging Materials. Nanomaterials (Basel). 2021;11(5). [PubMed ID: 34070054]. [PubMed Central ID: PMC8158105]. https://doi.org/10.3390/nano11051331.
-
7.
Mexis SF, Kontominas MG. Effect of oxygen absorber, nitrogen flushing, packaging material oxygen transmission rate and storage conditions on quality retention of raw whole unpeeled almond kernels (Prunus dulcis). LWT - Food Science and Technology. 2010;43(1):1-11. https://doi.org/10.1016/j.lwt.2009.06.024.
-
8.
Hasani-Javanmardi M, Fallah AA, Abbasvali M. Effect of safflower oil nanoemulsion and cumin essential oil combined with oxygen absorber packaging on the quality and shelf-life of refrigerated lamb loins. Lwt. 2021;147. https://doi.org/10.1016/j.lwt.2021.111557.
-
9.
Dey A, Neogi S. Oxygen scavengers for food packaging applications: A review. Trends in Food Science & Technology. 2019;90:26-34. https://doi.org/10.1016/j.tifs.2019.05.013.
-
10.
Marangoni Júnior L, Ito D, Ribeiro SML, Silva MGD, Alves RMV. Stability of β-carotene rich sweet potato chips packed in different packaging systems. Lwt. 2018;92:442-50. https://doi.org/10.1016/j.lwt.2018.02.066.
-
11.
Kütahneci E, Ayhan Z. Applications of different oxygen scavenging systems as an active packaging to improve freshness and shelf life of sliced bread. Journal of Consumer Protection and Food Safety. 2021;16(3):247-59. https://doi.org/10.1007/s00003-021-01331-3.
-
12.
Faas N, Röcker B, Smrke S, Yeretzian C, Yildirim S. Prevention of lipid oxidation in linseed oil using a palladium-based oxygen scavenging film. Food Packaging and Shelf Life. 2020;24. https://doi.org/10.1016/j.fpsl.2020.100488.
-
13.
Anthierens T, Ragaert P, Verbrugghe S, Ouchchen A, De Geest BG, Noseda B, et al. Use of endospore-forming bacteria as an active oxygen scavenger in plastic packaging materials. Innovative Food Science & Emerging Technologies. 2011;12(4):594-9. https://doi.org/10.1016/j.ifset.2011.06.008.
-
14.
Gaikwad KK, Singh S, Negi YS. Ethylene scavengers for active packaging of fresh food produce. Environmental Chemistry Letters. 2019;18(2):269-84. https://doi.org/10.1007/s10311-019-00938-1.
-
15.
Sadeghi K, Lee Y, Seo J. Ethylene Scavenging Systems in Packaging of Fresh Produce: A Review. Food Reviews International. 2019;37(2):155-76. https://doi.org/10.1080/87559129.2019.1695836.
-
16.
Wei H, Seidi F, Zhang T, Jin Y, Xiao H. Ethylene scavengers for the preservation of fruits and vegetables: A review. Food Chem. 2021;337:127750. [PubMed ID: 32861166]. https://doi.org/10.1016/j.foodchem.2020.127750.
-
17.
Sharma R, Ghoshal G. Emerging trends in food packaging. Nutrition & Food Science. 2018;48(5):764-79. https://doi.org/10.1108/nfs-02-2018-0051.
-
18.
Gaikwad KK, Singh S, Ajji A. Moisture absorbers for food packaging applications. Environmental Chemistry Letters. 2018;17(2):609-28. https://doi.org/10.1007/s10311-018-0810-z.
-
19.
Pinming C, Sukgorn N, Suhatcho T, Saetang B, Kerdkhong P, Maboonchuay T, et al. Humidity sensor using carboxymethyl cellulose hydrogel membrane. 2016 13th International Conference on Electrical Engineering/Electronics, Computer, Telecommunications and Information Technology (ECTI-CON). 2016. p. 1-3.
-
20.
Bourlieu C, Guillard V, Valles-Pamies B, Guilbert S, Gontard N. Edible moisture barriers: how to assess of their potential and limits in food products shelf-life extension? Crit Rev Food Sci Nutr. 2009;49(5):474-99. [PubMed ID: 19399672]. https://doi.org/10.1080/10408390802145724.
-
21.
Guo X, Liu L, Hu Y, Wu Y. Water vapor sorption properties of TEMPO oxidized and sulfuric acid treated cellulose nanocrystal films. Carbohydr Polym. 2018;197:524-30. [PubMed ID: 30007643]. https://doi.org/10.1016/j.carbpol.2018.06.027.
-
22.
Gaikwad KK, Singh S, Lee YS. High adsorption of ethylene by alkali-treated halloysite nanotubes for food-packaging applications. Environmental Chemistry Letters. 2018;16(3):1055-62. https://doi.org/10.1007/s10311-018-0718-7.
-
23.
Azeredo HMC, Otoni CG, Correa DS, Assis OBG, de Moura MR, Mattoso LHC. Nanostructured Antimicrobials in Food Packaging-Recent Advances. Biotechnol J. 2019;14(12). e1900068. [PubMed ID: 31465148]. https://doi.org/10.1002/biot.201900068.
-
24.
Suvarna V, Nair A, Mallya R, Khan T, Omri A. Antimicrobial Nanomaterials for Food Packaging. Antibiotics (Basel). 2022;11(6). [PubMed ID: 35740136]. [PubMed Central ID: PMC9219644]. https://doi.org/10.3390/antibiotics11060729.
-
25.
Otoni CG, Espitia PJ, Avena-Bustillos RJ, McHugh TH. Trends in antimicrobial food packaging systems: Emitting sachets and absorbent pads. Food Research International. 2016;83:60-73. https://doi.org/10.1016/j.foodres.2016.02.018.
-
26.
Sängerlaub S, Lehmann E, Müller K, Wani AA. Porosity, thickness distribution and water vapour sorption of thermoformed foamed PP films with dispersed sodium chloride for humidity regulation. Food Packaging and Shelf Life. 2020;24. https://doi.org/10.1016/j.fpsl.2020.100482.
-
27.
Sängerlaub S, Seibel K, Miesbauer O, Pant A, Kiese S, Rodler N, et al. Functional properties of foamed and/or stretched polypropylene-films containing sodium chloride particles for humidity regulation. Polymer Testing. 2018;65:339-51. https://doi.org/10.1016/j.polymertesting.2017.12.002.
-
28.
Choi HY, Lee YS. Characteristics of moisture-absorbing film impregnated with synthesized attapulgite with acrylamide and its effect on the quality of seasoned laver during storage. Journal of Food Engineering. 2013;116(4):829-39. https://doi.org/10.1016/j.jfoodeng.2013.01.023.
-
29.
Hansen AÅ, Moen B, Rødbotten M, Berget I, Pettersen MK. Effect of vacuum or modified atmosphere packaging (MAP) in combination with a CO 2 emitter on quality parameters of cod loins ( Gadus morhua ). Food Packaging and Shelf Life. 2016;9:29-37. https://doi.org/10.1016/j.fpsl.2016.05.005.
-
30.
Kuswandi B; Jumina. Active and intelligent packaging, safety, and quality controls. Fresh-Cut Fruits and Vegetables. 2020. p. 243-94. https://doi.org/10.1016/b978-0-12-816184-5.00012-4.
-
31.
Lee DS, Wang HJ, Jaisan C, An DS. Active food packaging to control carbon dioxide. Packaging Technology and Science. 2021;35(3):213-27. https://doi.org/10.1002/pts.2627.
-
32.
Vanmathi Mugasundari A, Anandakumar S. Shelf life extension of bread using ethanol emitters with different packaging materials. Journal of Food Processing and Preservation. 2022;46(12). https://doi.org/10.1111/jfpp.17143.
-
33.
Rangaraj V, Rambabu K, Banat F, Mittal V. Natural antioxidants-based edible active food packaging: An overview of current advancements. Food Bioscience. 2021;43. https://doi.org/10.1016/j.fbio.2021.101251.
-
34.
Mu H, Gao H, Chen H, Fang X, Han Q. A novel controlled release ethanol emitter: preparation and effect on some postharvest quality parameters of Chinese bayberry during storage. J Sci Food Agric. 2017;97(14):4929-36. [PubMed ID: 28397258]. https://doi.org/10.1002/jsfa.8369.
-
35.
Yuan L, Gao M, Xiang H, Zhou Z, Yu D, Yan R. A Biomass-Based Colorimetric Sulfur Dioxide Gas Sensor for Smart Packaging. ACS Nano. 2023;17(7):6849-56. [PubMed ID: 36971497]. https://doi.org/10.1021/acsnano.3c00530.
-
36.
Pisoschi AM, Pop A, Gajaila I, Iordache F, Dobre R, Cazimir I, et al. Analytical methods applied to the assay of sulfur-containing preserving agents. Microchemical Journal. 2020;155. https://doi.org/10.1016/j.microc.2020.104681.
-
37.
Gemili S, Yemenicioğlu A, Altınkaya SA. Development of antioxidant food packaging materials with controlled release properties. Journal of Food Engineering. 2010;96(3):325-32. https://doi.org/10.1016/j.jfoodeng.2009.08.020.
-
38.
Kuai L, Liu F, Chiou B, Avena-Bustillos RJ, McHugh TH, Zhong F. Controlled release of antioxidants from active food packaging: A review. Food Hydrocolloids. 2021;120. https://doi.org/10.1016/j.foodhyd.2021.106992.
-
39.
Marangoni Junior L, Vieira RP, Jamroz E, Anjos CAR. Furcellaran: An innovative biopolymer in the production of films and coatings. Carbohydr Polym. 2021;252:117221. [PubMed ID: 33183647]. https://doi.org/10.1016/j.carbpol.2020.117221.
-
40.
Menzel C, Gonzalez-Martinez C, Chiralt A, Vilaplana F. Antioxidant starch films containing sunflower hull extracts. Carbohydr Polym. 2019;214:142-51. [PubMed ID: 30925982]. https://doi.org/10.1016/j.carbpol.2019.03.022.
-
41.
Wrona M, Silva F, Salafranca J, Nerín C, Alfonso MJ, Caballero MÁ. Design of new natural antioxidant active packaging: Screening flowsheet from pure essential oils and vegetable oils to ex vivo testing in meat samples. Food Control. 2021;120. https://doi.org/10.1016/j.foodcont.2020.107536.
-
42.
Rodríguez GM, Sibaja JC, Espitia PJ, Otoni CG. Antioxidant active packaging based on papaya edible films incorporated with Moringa oleifera and ascorbic acid for food preservation. Food Hydrocolloids. 2020;103. https://doi.org/10.1016/j.foodhyd.2019.105630.
-
43.
Kamarudin SH, Rayung M, Abu F, Ahmad S, Fadil F, Karim AA, et al. A Review on Antimicrobial Packaging from Biodegradable Polymer Composites. Polymers (Basel). 2022;14(1). [PubMed ID: 35012197]. [PubMed Central ID: PMC8747113]. https://doi.org/10.3390/polym14010174.
-
44.
Fang Z, Zhao Y, Warner RD, Johnson SK. Active and intelligent packaging in meat industry. Trends in Food Science & Technology. 2017;61:60-71. https://doi.org/10.1016/j.tifs.2017.01.002.
-
45.
Maisanaba S, Llana-Ruiz-Cabello M, Gutiérrez-Praena D, Pichardo S, Puerto M, Prieto AI, et al. New advances in active packaging incorporated with essential oils or their main components for food preservation. Food Reviews International. 2016;33(5):447-515. https://doi.org/10.1080/87559129.2016.1175010.
-
46.
Felix de Andrade M, Diego de Lima Silva I, Alves da Silva G, David Cavalcante PV, Thayse da Silva F, Bastos de Almeida YM, et al. A study of poly (butylene adipate-co-terephthalate)/orange essential oil films for application in active antimicrobial packaging. Lwt. 2020;125. https://doi.org/10.1016/j.lwt.2020.109148.
-
47.
Wang H, Liu H, Chu C, She Y, Jiang S, Zhai L, et al. Diffusion and Antibacterial Properties of Nisin-Loaded Chitosan/Poly (L-Lactic Acid) Towards Development of Active Food Packaging Film. Food and Bioprocess Technology. 2015;8(8):1657-67. https://doi.org/10.1007/s11947-015-1522-z.
-
48.
Basch CY, Jagus RJ, Flores SK. Physical and Antimicrobial Properties of Tapioca Starch-HPMC Edible Films Incorporated with Nisin and/or Potassium Sorbate. Food and Bioprocess Technology. 2012;6(9):2419-28. https://doi.org/10.1007/s11947-012-0860-3.
-
49.
McArdle RA, Marcos B, Mullen AM, Kerry JP. Influence of HPP conditions on selected lamb quality attributes and their stability during chilled storage. Innovative Food Science & Emerging Technologies. 2013;19:66-72. https://doi.org/10.1016/j.ifset.2013.04.003.
-
50.
Sobeh M, Mahmoud MF, Abdelfattah MAO, Cheng H, El-Shazly AM, Wink M. A proanthocyanidin-rich extract from Cassia abbreviata exhibits antioxidant and hepatoprotective activities in vivo. J Ethnopharmacol. 2018;213:38-47. [PubMed ID: 29126990]. https://doi.org/10.1016/j.jep.2017.11.007.
-
51.
Weiss J, Loeffler M, Terjung N. The antimicrobial paradox: why preservatives lose activity in foods. Current Opinion in Food Science. 2015;4:69-75. https://doi.org/10.1016/j.cofs.2015.05.008.