Abstract
Keywords
1. Context
In the past few decades, our understanding has grown to recognize that, in addition to eukaryotic cells, the human body contains an abundant population of microorganisms. These microorganisms reside in various tissues and bodily fluids, with a significant concentration in the digestive tract, primarily within the colon (1). Healthy individuals exhibit a consistent continuity of skin microorganisms. The presence of a stable assembly of cooperative microorganisms has been linked to beneficial health effects. In contrast, a shift from cooperative microorganisms to pathogens can result in microbial imbalance and illness. By altering the composition and reducing the population of bacteria that make up the normal flora, the competitive environment on the skin is weakened, allowing pathogenic bacteria to grow. The transient or enduring occupants of the body's internal and external interfaces include bacteria, archaea, viruses, and fungi. Disparities in the composition of the microbiome have been observed in the intestinal tract and skin of individuals with atopic dermatitis compared to those who are healthy (2).
2. The Human Microbiome
For several decades, the coexistence of harmless or beneficial and harmful microorganisms in the human body has been recognized and studied. However, more recently, a deeper fascination has emerged, leading to extensive studies using innovative methodologies to identify microbial entities. This progress has led to the coining of the term 'human microbiome,' introduced by Joshua Lederberg (3). The microbiome includes entire microbial categories and their genetic material, encompassing both temporary and established bacteria, archaea, viruses, and fungi (4). Some authors even compare the human body and its symbiotic bacteria to a superorganism, similar to how insects like ants and honeybees colonize (5).
Microbes residing on the mucous membranes and skin can be categorized into distinct groups based on their positive or negative impact on the host, their persistence over time, and their optimal locations for colonization within the body. Favorable associations are marked by either commensalism or mutualism. Various regions of the human body serve as distinct environments, including dry regions, warm and moist regions like skin folds, and oxygen-deprived environments like the intestines (6) (Figure 1). Additionally, areas such as sebaceous follicles and sebum-rich regions on the face and back contribute to diverse microbial niches (7), further enriching the microbial landscape. This intricate ecosystem creates interaction opportunities between the host and microorganisms (8). A summary of some studies on the relationship between the microbiome and atopic dermatitis is listed in Table 1.
The most frequent normal flora on skin and gut

A Summary of Some Studies on the Relationship Between the Microbiome and Atopic Dermatitis
Authors | Findings | Ref |
---|---|---|
Paller et al. | The key role of the microbiome in the pathogenesis of atopic dermatitis (AD); use of antiseptics and antibiotics for AD treatment; use nonspecific immunomodulators with effect on microbiome for AD treatment; effects of infancy gut microbiome changes in AD development; effects of Staphylococcus aureus vaccines to manipulate AD skin microbiome | (2) |
Hu et al. | Observed that the diversity, relative abundance, and pathways derived from stool microbiota were associated with allergy, eczema, food allergy and asthma at school age; the role of stool microbiota on atopic diseases seems related to later childhood. | (9) |
Lee et al. | Staphylococcus aureus species of the skin microbiome play key roles in the development and establishment of AD; the role of S. aureus in gut and that’s effects on AD is unclear. | (10) |
Song et al. | Increase the number of active F. prausnitzii in the gut of AD infants; different nutrients released from the damaged gut epithelium can help the growth of F. prausnitzii in the gut of AD infants. | (11) |
Lee et al. | They didn't see the difference between the gut microbiome of AD patients and eosinophilic inflamed patients; they found the inverse correlation between Clostridia and blood eosinophil count and weak correlation between E. coli and blood eosinophils. | (12) |
Nylund et al. | They found the inverse correlation between eczema severity and microbiota diversity, as well as the load of butyrate-producing bacteria. | (13) |
Kim et al. | They found the increase of staphylococcus, pseudomonas and streptococci in AD lesions of AD compared to healthy controls with higher number of Sediminibacterium and Lactococcus. | (14) |
Shi et al. | They found that the skin microbiome is different in pediatric and adult AD. In children, they saw an increase of Gemella, Streptococcus, Haemophilus, Rothia, and Granulicatella, and in adults, they saw increase of Finegoldia, Corynebacterium, Propionibacterium, Anaerococcus, Lactobacillus, and Staphylococcus. | (15) |
3. Detection Methods
Before the advancements in contemporary sequencing methods, numerous inquiries utilized culture-dependent approaches to explore the microbiome of human skin across different habitats. Many investigations employing conventional techniques examined the impact of various external and internal materials, as well as environmental factors like cleansing agents and body washing. These studies also encompassed diverse ecological niches, such as sebum-rich areas and the infundibula of hair follicles (16). For instance, the effect of povidone-iodine was studied on the brow, a sebum-rich region, in contrast to 60% n-propanol on the outer skin and infundibulum of sebaceous glands (17). Furthermore, four additional skin disinfectants, including octenidine hydrochloride (18), were evaluated using the standardized forehead skin test (SFST) initially proposed in 1981 (19).
More extensive exploration has recently been conducted concerning antiseptic agents that induce personalized, short-term, and location-specific shifts in the native bacterial communities on the skin. Chlorhexidine, water, povidone-iodine, and ethanol were studied at two distinct skin sites: The forearm and the back (20). These findings offer valuable insights into the robustness and adaptability of the skin microbiome, concurrently revealing how topical antiseptic treatment affects the dynamics of skin bacteria and the overall community ecosystem. The methods employed to collect skin flora samples, including swabs, scrubs, cyanoacrylate (21), and skin biopsies, play a pivotal role in the outcomes. Since the skin constitutes a three-dimensional environment for bacteria, variations in niches and the bacterial strains accessed by each sampling technique are evident. These discrepancies necessitate careful consideration in microbiome studies. Compliance with protocols before sampling, the type of swabs used, and the skill of the sampler impact the results of microbiome investigations. Additionally, the site of bacterial colonization is important. Most bacteria are superficial colonizers, but some are deep colonizers, such as those in hair follicles, so different methods may yield different results.
Both modern sequencing techniques and culture-based methods bring distinct advantages, significantly shaping the outcomes of investigative studies. Conventional culture-based methods can identify about half of the skin's microbial diversity. The advantages of culture-oriented approaches lie in the validation of living microorganisms and the substantial production of cloned DNA. Metagenomics, which harnesses microbial DNA through techniques like polymerase chain reaction (PCR), DNA chips, and sequencing, opens up valuable avenues to reveal concealed diversity and interactions between microorganisms (14). The viability of collected microorganisms can be readily confirmed with culture-based methods, but some techniques also align with sequencing methods (22). In summary, culture-based methods are time-consuming and labor-intensive, while modern sequencing techniques are much faster and easier. On the other hand, culture-based methods are cheaper and more accessible than sequencing techniques such as next generation sequencing (NGS).
In the context of fungi, Kondori et al. compared duplex PCR employing a dermatophyte primer with traditional culture and microscopic techniques. Their findings demonstrated the potential of duplex PCR as a promising detection method, primarily due to its ability to deliver a diagnosis within a significantly reduced timeframe (23).
4. The Gut Microbiome
The gut harbors a diverse range of phyla by default. The specific makeup of microorganisms varies even among individuals in good health, and this diversity can be impacted by factors such as gender, age, or dietary choices. The phyla that constitute a well-balanced gut microbiome in adults include Bacteroidetes, firmicutes, proteobacteria, and actinobacteria (1). Distinctive patterns within the gut microbiome have been identified in connection with various illnesses, encompassing variations in diversity and the recurrent presence of particular bacterial strains. Associations have been observed among conditions such as hypertension, chronic kidney disease, obesity, and even breast cancer, and the microbiota within the individual's gut. For example, in cases of hypertension, researchers noted a reduction in the diversity of microorganisms and a decrease in the abundance of typically beneficial commensal microbes, characteristics typically found in a healthy individual's microbiome (24). In addition to above conditions, as a result of excessive use of some antibiotics, the balance of intestinal flora is disturbed. Also, by using probiotics, the composition of this population can be changed in a controlled way. Exploring mechanisms like the generation of advantageous metabolites, which could potentially shed light on whether particular patterns are a cause or an outcome of diseases, is an ongoing area of study that warrants further investigation.
5. Gut Commensals and Atopic Dermatitis
It is commonly believed that commensal microorganisms contribute positively to the development of the immune system, and certain types of human commensals have the capacity to trigger substantial immune reactions. Maintaining a harmonious balance between the host and the commensal gut microbiome depends significantly on the functioning of the innate immune system (25). Certain types of innate immune cells located in mucosal tissues, for instance, detect commensal bacteria using toll-like receptors (TLRs), thereby controlling the extent to which these microorganisms can breach the intestinal barrier (26). This interaction can initiate antigen presentation and play a pivotal role in the progression of the innate immune system.
In 1989, Strachan proposed that the emergence of atopic diseases might be attributed to either a lack of cross-contamination or the advancement of personal hygiene and living conditions. Subsequent research supports this hypothesis, particularly regarding the reduced diversity of the gut microbiome observed in infants with AD (15). Beyond its role in regulating the immune system's development, the gut microbiome also contributes to creating compounds like conjugated linoleic acid, which is suspected to have anti-inflammatory properties (27). Moreover, it might exert a beneficial impact by curbing the production of cytokines in the skin. Some experts refer to this interaction as the gut-skin axis (10). This concept refers to the bidirectional relationship between the gut microbiome and skin health. Several mechanisms, such as the intestinal barrier, inflammatory mediators, and metabolites, have been proposed for the gut-skin axis. For example, the gut-dwelling microbe Clostridium sporogenes generates metabolites derived from aromatic amino acids, which subsequently impact the functioning of the host's intestinal system. Some of these metabolites, including indole propionic acid, exhibit anti-inflammatory properties (28). Exploring the implications of these attributes and whether they influence AD is a compelling avenue for further investigation. This is particularly intriguing due to indications that the interplay between the gut microbiome and immune cells might play a role in influencing both the development and severity of AD (11).
Research findings indicate that the microorganisms inhabiting the intestinal tract change throughout childhood. The composition of gut microorganisms among children could potentially impact the development of AD and coincide with the onset of eczema (12). However, the means to foster a beneficial evolution in this regard, as well as whether the disparities in microbial composition are fundamental or consequential, require further exploration.
6. The Start and Impacts of Colonization
Numerous factors play a role in shaping an individual's microbiome composition. The conventional belief was that a fetus remains sterile before birth, with initial contact and colonization starting during the birthing process. However, recent evidence suggests that microbial colonization might commence before childbirth. In female mice capable of bearing offspring, which were administered labeled strains of Enterococcus faecium orally, identical bacteria were discovered in the amniotic fluid. Comparable outcomes were achieved in human subjects. When blood samples from the umbilical cords of women who underwent cesarean section (CS) deliveries were cultured, gram-positive cocci were identified in nine out of 20 samples from healthy newborns (29).
In addition to the mother's bacterial flora acting as a source of microbial inheritance during pregnancy, studies such as those conducted by Shin et al. have demonstrated that the method of delivery, whether vaginal or CS, also impacts the constitution of the newborn's microbiome (30). Backhed et al. conducted research involving fecal samples from children born via CS. They found that the gut microbiome consisted of Haemophilus spp., Enterobacter spp., Streptococcus spp., Veillonella spp., and Staphylococcus spp. (31). Since CS procedures are generally carried out under strictly sterile conditions, newborns acquire microorganisms from the hospital environment rather than their mother's vaginal or skin microflora. This dynamic could significantly influence the developing immune system of the infant.
A separate study, which included the collection of meconium samples, yielded similar outcomes. Bacteria were identified in over 66% of the samples, with Staphylococcus spp. being the most frequent. Even the meconium of infants delivered through CS was not entirely sterile. Furthermore, infants born vaginally exhibited a broader range of bacteria, while those born via CS experienced a delay in the colonization of Bacteroides fragilis (32). Microorganisms obtained during vaginal birth are considered crucial for the natural development of the infant's gut microbiome. Although being born via CS appears to heighten the likelihood of developing food allergies beyond 36 months, it does not seem to increase the risk of developing AD (33).
The establishment of an individual's gut microbiome also differs depending on whether an infant is breastfed or formula-fed. Breastfed infants tend to have a less varied gut microbiome. Among breastfed newborns, Bifidobacterium spp. is the prevailing microorganism. In breastfed children, the quantities of Bifidobacterium spp. were more than double those in their bottle-fed counterparts (34). Microorganisms commonly found in breast milk include Streptococci, Staphylococci, Micrococci, Enterococci, and Lactobacilli. However, it is possible that some of these may originate from the mother's skin and might be contaminants (35).
The impact of breastfeeding on the progression of atopic dermatitis is a matter of debate, involving several factors that require thorough examination. The complexity arises because a mother's milk, if she has experienced or is experiencing atopic dermatitis or related conditions (ADRD), might not yield the same beneficial outcomes for her infant as the milk from a non-atopic mother (36). This discrepancy could be due to the potential diminishment in the protective attributes of breast milk, which might occur as early as four weeks after breastfeeding initiation. A reduction in omega-6 and omega-3 fatty acids has been observed in mothers with atopic dermatitis who breastfeed. Following this, within the subsequent five months, alterations in the milk's composition occur at the expense of protective constituents, thereby increasing the child's susceptibility to developing atopic dermatitis (13). Nevertheless, women with atopic dermatitis should not discontinue breastfeeding, as the transfer of protective antibodies to the child via breast milk continues. Certain experts suggest the supplementation of fatty acids like omega-3 and omega-6 during the third trimester and continuing throughout the entire breastfeeding period (37). Research has indicated that breastfeeding among children from atopy-prone families acts as a safeguard against atopy. However, these outcomes were not observed in children without risk factors and were not as pronounced in the broader population (38).
The skin microbiome of a newborn shortly after birth is also affected by the delivery method and undergoes transformations as the child matures. Generally, neonates delivered vaginally tend to exhibit a microbial composition that closely resembles their mother's vaginal flora (39). In a systematic review of various studies, researchers suggested that there might be unexamined variables complicating the assessment of the impact of the delivery method versus the underlying reasons influencing the choice against natural birth (40). Additionally, during the early stages of life, the infant's skin microbiome appears relatively unstable and gradually achieves stability as time progresses (41). Approximately 1 to 2 months post-delivery, Capone and Paller observed no discernible disparities in the microbial composition among infants, regardless of the delivery mode (42).
7. The Relationship Between the Skin Microbiome and Atopic Dermatitis
Microorganisms that have been extensively investigated for their association with the worsening of atopic dermatitis include Staphylococcus aureus bacteria and fungi of the Malassezia genus. Furthermore, viruses such as the herpes simplex virus (HSV) might also contribute to the deterioration of the condition. Skin affected by atopic dermatitis tends to accommodate pathogenic microorganisms like S. aureus, while also exhibiting a diminished array of beneficial commensals (43). The dynamics among microorganisms play a pivotal role in inflammation, with inflamed skin exhibiting reduced diversity of microorganisms compared to healthy skin. Promoting the growth of commensals results in a reduction of both inflammation and the abundance of S. aureus (44) (Figure 2). Additionally, the interplay between microorganisms and the immune cells residing in the skin appears to influence the disease's progression. Thus, an intricate network of interactions between microorganisms, their fellow microbes, and the host contributes to an inflammatory cycle. Comprehending all constituents of this cycle remains an actively studied domain.
Crosstalk between skin and microbiome in healthy and atopic dermatitis conditions. The presence of normal flora on the skin interacts with the host to establish a functional immune response and prevents the overgrowth of pathogens. On healthy skin (Left), there is high microbial diversity. The skin of atopic dermatitis (AD) patients (Right) is characterized by the overgrowth of pathogenic microbes such as Staphylococcus aureus, and reduced microbial diversity. Staphylococcus aureus virulence factors including d-toxin and superantigens trigger IgE-mediated mast cell degranulation, and the resulting increase in Th2 cytokines perpetuates AD.
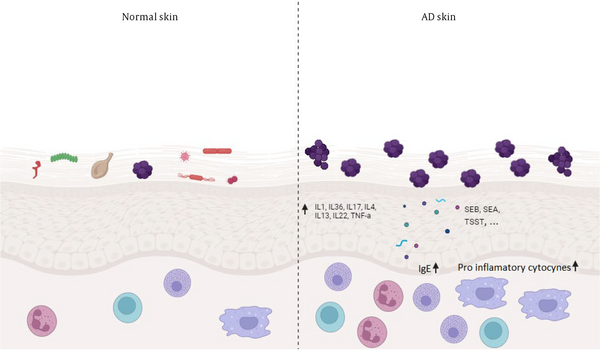
8. Staphylococcus aureus
Staphylococcus aureus is responsible for a range of infections, from minor pimples to conditions like erysipelas and staphylococcal scalded skin syndrome (SSSS) through the actions of its exfoliative toxins A and B. Staphylococcus aureus is known for producing stable superantigens and exoproteins that resist heat, dryness, and proteolysis (45). These proteins can bind to MHC-2 molecules and T-cells carrying specific T-cell receptors (TCR). Most individuals with exotoxin-producing S. aureus exhibit IgE sensitization (46). In chronic atopic dermatitis lesions, S. aureus has been identified in 90% of cases. Exudative lesions contained S. aureus in all sampled instances, exceeding a density of 14 × 106 organisms per cm2 (47). Higher-density colonization with S. aureus was linked to more severe flare-ups and elevated severity scoring of atopic dermatitis (SCORAD) scores compared to colonization by other Staphylococcus species like Staphylococcus epidermidis, which were related to milder disease courses (48). Conversely, S. aureus was found on about 5% of healthy individuals' skin using culture-based methods (49).
Varied distributions of colony clusters were identified in neonates and individuals over 80 years of age, and the observed virulence attributes can contribute to infection development. As a result, decolonization strategies could be considered for specific patients based on their infection risk. The amounts of certain defensins and cathelicidins, essential components of the skin's innate immune system, are observed to be lower in the inflamed skin of AD patients compared to psoriasis plaques (50). This deficiency in antimicrobial peptides, along with other disruptions in the skin barrier, such as abnormal levels of filaggrin, can render inflamed skin more susceptible to S. aureus infiltration and subsequent infection. Remarkably, S. aureus itself can initiate and worsen the inflammatory response in AD by generating factors that alter the host's immune response or compromise the integrity of the skin barrier.
Research indicates that certain strains of S. aureus can induce the production of kallikreins by keratinocytes to varying degrees (51). Kallikreins, a class of serine proteases, are responsible for the degradation of filaggrin and desmoglein-1 (52). This disruption in the normal desquamation process contributes to an impaired barrier function of the skin. Furthermore, S. aureus stimulates keratinocytes to release pro-inflammatory interleukins (IL)-1α and IL-36α, along with their respective receptors, IL-1R and IL-36R. This cascade, triggered by the induction of IL-17, leads to an inflammatory response (53).
Another noteworthy challenge is S. aureus's capability to create biofilms. These biofilms are intricate microbial communities that allow bacteria to evade the host's immune defense and resist antibiotics. Isolates obtained from AD patients demonstrated a strong capacity to generate biofilms. In a recent study conducted by Allen et al., it was discovered that S. aureus biofilms were widespread within AD lesions (54). Similarly, Tankersley et al. showed that exposure to S. aureus biofilm-conditioned media resulted in notably heightened inflammatory reactions in human keratinocytes in vitro, in contrast to media conditioned with planktonic bacteria. Following exposure, these keratinocytes rapidly experienced decreased viability and underwent apoptosis (55). This suggests that S. aureus biofilms can diminish the secretion of antimicrobial peptides by affecting their origin.
9. Other Bacteria
Although S. aureus is the bacterial species most commonly linked to exacerbations in atopic dermatitis, some scholars argue that other skin bacteria contributing to flares may not be receiving sufficient attention (56). Interactions among microorganisms within the skin's microbiota play a pivotal role in the development of AD. For instance, AD flares have been associated with reduced microbial diversity and the dominance of a specific microbe, such as S. aureus (56). This dysbiosis tends to resolve as lesions heal (56). Consequently, reinstating commensal bacteria could be an effective strategy for managing dysbiosis and alleviating AD symptoms.
For example, the experimental introduction of Staphylococcus hominis or S. epidermidis strains to the skin of two individuals with AD led to a notable reduction in S. aureus colonization (44). Moreover, skin commensals such as S. epidermidis play a vital role in IL-1 signaling, a fundamental pathway for the innate immune system. In one study, mice devoid of microorganisms were infected with Leishmania major, leading to the development of wounds. However, when these animals were treated with topically applied S. epidermidis strains, their skin healing significantly improved (44).
Staphylococcus epidermidis has also been associated with anti-inflammatory properties. A study demonstrated that S. epidermidis triggered non-inflammatory pathways following injury by suppressing TLR3 expression in keratinocytes. This effect was linked to staphylococcal teichoic acid (57). Immune responses to commensals, as considered earlier, differ from those toward pathogens. Studies have indicated that commensals promote the development of particular T-cells without inducing inflammation, displaying immunoregulatory and reparative markers (58).
10. Malassezia Species
Around a century ago, the discovery of Malassezia spp. marked a significant milestone. These microorganisms were initially labeled as Pityrosporum orbiculare/ovale. They are a component of the typical microbial community residing on human skin and are predominantly localized in regions characterized by substantial sebum production, such as the scalp, chest, and back. In these areas, they establish themselves within the outermost layer of the skin, known as the stratum corneum.
Presently, our understanding encompasses approximately 14 lipophilic yeasts within the Malassezia genus. Most of these yeasts lack the genetic machinery required for synthesizing fatty acids. This genetic trait explains their affinity for seborrheic sites, although an exception exists in the form of M. pachydermatis, which can flourish even without external sources of lipids. Other species compensate for this deficiency by secreting various lipases and phospholipases that facilitate the breakdown of oils present in the sebum produced by their host's skin. Although Malassezia spp. are usually present on healthy skin, they can also play a role in inciting or exacerbating various conditions such as seborrheic dermatitis, folliculitis, pityriasis versicolor, and AD (59).
The frequent occurrence of positive Malassezia spp. samples in both healthy individuals and AD patients underscores the prevalence of this species. Curiously, the rate of positive culture recoveries in AD patients was comparatively lower than in their healthy counterparts (60). The most prevalent species can be found in both controls and patients, varying depending on the geographical area. However, specific species appear to be more prevalent in skin regions with lesions than in non-lesional areas, prompting further investigation (61). Despite this, the true impact of Malassezia spp. remains somewhat unclear. Nevertheless, AD patients appear to benefit from antifungal treatments effective against Malassezia spp. (62).
Notably, during atopy scratch tests utilizing Malassezia extract, over 30% of patients displayed positive reactions. Additionally, a link between the severity of eczema and Malassezia antigens has been established (63). Apparent discrepancies in antibody production were evident; among healthy volunteers, IgG and IgM antibodies were detected, indicating a standard interaction between yeast and the immune system (64). Conversely, adults with AD exhibited specific IgE antibodies (62). In the case of children, there was no observable connection between IgE-mediated sensitization to Malassezia and SCORAD scores (65). Another study identified a link between gender and age regarding IgE-mediated sensitization, with a higher prevalence in adult males (66). Eczema incited by Malassezia generally manifests in seborrheic zones, such as the head and neck region, accounting for the shifting distribution pattern of AD during and after adolescence.
11. Other Fungi
Malassezia represents a typical example within the fungal realm. Other fungi coexist within the human body, including opportunistic molds like Aspergillus spp., dermatophytes such as Trichophyton, Microsporum, and Epidermophyton, as well as yeasts like Candidaalbicans and Malassezia spp. Candida spp. are part of the normal flora of mucous membranes and can be found in the gastrointestinal tract of symptomless carriers (67). Under certain circumstances, such as in patients with skin conditions, cancer patients, those with compromised immune systems, and individuals residing in humid and warm environments, C. albicans can even be cultured from non-hairy skin (68). Infections might then arise due to additional predisposing factors and the virulence of the infecting microorganism.
Notably, Th17 cells play a pivotal role in reacting to the yeast form of C. albicans, rather than the filamentous variety (69). Another study found no noteworthy contrast in Candida spp. skin colonization between individuals with AD and those without the condition (70). Conversely, other studies noted that C. albicans, in particular, can be isolated with greater frequency from both unaffected and affected skin areas in AD patients than in healthy individuals (71), as well as from the gastrointestinal tract (59). Furthermore, Savolainen et al. established a correlation between the severity of AD and the generation of C. albicans-specific IgE antibodies in individuals with AD (72). This suggests that Candida spp. could potentially activate the immune system by releasing various allergens, thereby triggering the onset or worsening of AD. Consequently, it has been proposed that Candida spp. colonization in AD patients should be evaluated and managed.
Persistent dermatophyte infections are believed to be more common in individuals with AD (70). According to Svejgaard et al., there is an elevated vulnerability to Trichophyton rubrum infections on the feet of atopic children and adolescents, along with a greater likelihood of enduring infections. Nonetheless, the findings present some inconsistencies, as they also revealed that acute infections can be entirely resolved in at least a subset of these patients (70).
12. Herpes Simplex Virus
HSV-1 and HSV-2 are widely prevalent DNA viruses known for their neuroinvasive and neurotoxic characteristics, capable of latent persistence. A significant proportion of individuals carry HSV; however, disparities in socioeconomic status have been noted, with a lower socioeconomic standing correlating with earlier infections. The virus gains entry through minor lesions on the skin or mucous membranes, leading to primary infection and subsequent antibody production. A few latent viruses persist in sensory neurons over time. In addition to causing cold sores and genital herpes, HSV can result in serious health complications like encephalitis and necrotizing hepatitis (73).
A particularly noteworthy dermatological condition associated with HSV is Kaposi's varicelliform eruption (KVE) or eczema herpeticum. Kaposi's varicelliform eruption exemplifies a secondary infection that arises over underlying skin conditions. A mortality rate of approximately 9% has been documented for KVE. This condition has been observed in various diseases that disrupt the skin's barrier function, including pemphigus, Darier’s disease, Grover's disease, cutaneous lymphomas, and AD. While perhaps not as numerous as S. aureus infections, a study examining 179 children with AD over 2.75 years in the 1980s revealed 21 symptomatic episodes of herpes simplex infection (74).
Comprehensive treatment, both topical and systemic, is essential for individuals with severe superinfections caused by HSV. Antiviral therapy involving medications like acyclovir, its derivatives, or foscarnet must be administered over several days. Particular attention is necessary to address the presence of keratitis (75).
13. Other Viruses
In individuals with AD, similar to widespread herpes infections, there is a greater prevalence of other skin diseases caused by viruses. Examples include human papillomavirus (HPV) and molluscum contagiosum virus. Molluscum contagiosum is frequently encountered in children with AD and individuals with compromised immune systems, often manifesting as a disseminated variant. The clinical appearance of molluscum contagiosum is characterized by skin-colored papules with a central depression, typically measuring 3 - 5 mm in diameter. Scratching can lead to self-inoculation of the poxvirus. Generally, this condition is self-limiting; using band-aids to prevent self-inoculation and employing careful removal techniques can accelerate the healing process (76).
14. Normalization and Treatment
The prevalence of atopic conditions is rising. Atopic dermatitis (AD), as a related disorder, is a complex ailment with varying degrees of severity, impacting not only the affected individuals but also posing a challenge for public health systems and healthcare services. Since approximately one in ten individuals is affected by AD, there is a substantial demand for appropriate treatment. However, the intricacies of this situation encompass various contributing factors, including the potential influence of the AD-specific microbiome and the restoration of dysbiosis. Dysbiosis within the gut could contribute to or be associated with exacerbations of the condition.
As a potential approach, the oral administration of synbiotics, which combine pro- and prebiotics, has been introduced for AD patients. Probiotics, primarily Lactobacillus and Bifidobacteria, are considered beneficial bacteria that can withstand the low pH of the stomach and induce positive health effects. Prebiotics, on the other hand, are substances that can induce alterations in the microbiome. Modern prebiotics include non-digestible oligosaccharides with bifidogenic properties. Most research studies on AD following the consumption of probiotics and synbiotics showed favorable effects, resulting in an improvement in SCORAD scores (77). For instance, a randomized placebo-controlled trial involving 53 children with moderate to severe AD supplemented with the probiotic Lactobacillusfermentum VRI-003 PCC indicated a statistically significant improvement in AD (P = 0.03) (78).
However, it is essential to acknowledge that not all published findings demonstrated an improvement in SCORAD scores or a substantial reduction in the likelihood of AD development. This discrepancy might indicate strain-specific attributes. Additionally, C. sporogenes and its associated metabolites hold promise for further exploration. Notably, its anti-inflammatory metabolite indole propionic acid has shown beneficial qualities in relation to the development of type 2 diabetes mellitus, yet its implications for AD remain uncharted. Given that compromised barrier function and elevated epidermal water loss are pivotal components of AD pathology, topical treatment involving emollients is typically recommended as a foundational therapeutic approach (79).
The application of the probiotic strain Lactococcus lactis onto the skin of a mouse model yielded promising outcomes, such as the alleviation of AD symptoms and the suppression of histamine levels (80). Established treatments have also impacted the microbiome composition. For patients displaying signs of bacterial superinfections, the use of intermittent bleach baths and topical mupirocin ointment in the nasal passages resulted in a reduction in AD severity (81). UVB phototherapy has long been an approach to AD treatment. A study conducted in 2006 demonstrated that UVB treatment contributed to a decrease in the microbial load of S. aureus (82). Atopic dermatitis localized to the head and neck, which often correlates with Malassezia, can be managed through the localized application of ketoconazole creams and shampoos.
15. Conclusions
Gathering clinical observations involves labor-intensive foundational research aimed at uncovering the underlying mechanisms, which we are only just beginning to unravel. Monitoring the interactions between microorganisms and between microorganisms and the host throughout the progression of the disease is a process that requires additional time and resources. Based on some evidence, the effects of metabolites produced by gut bacteria on AD have been demonstrated in mice. However, further investigations are necessary to evaluate the effectiveness of this approach in the development and progression of AD in humans. Given that AD is a multifaceted chronic ailment, its treatment must also encompass other interrelated pathways. Addressing dysbiosis, for instance, necessitates tackling impaired skin barrier function through adequate rehydration or even the topical supplementation of components like ceramides. The skin's pH level might also warrant attention; adjusting the pH below five could potentially favor the physiological flora, particularly benefiting S. epidermidis. Another area to explore would involve investigating the long-term effects of focused systemic therapies, like dupilumab, on the microbial composition within AD patients.
Finally, it should be noted that the findings collected in this review are not yet proven for clinical use, and more research is needed to justify any clinical practices aimed at the microbiome in AD patients.
References
-
1.
Gensollen T, Blumberg RS. Correlation between early-life regulation of the immune system by microbiota and allergy development. J Allergy Clin Immunol. 2017;139(4):1084-91. [PubMed ID: 28390575]. [PubMed Central ID: PMC5402752]. https://doi.org/10.1016/j.jaci.2017.02.011.
-
2.
Paller AS, Kong HH, Seed P, Naik S, Scharschmidt TC, Gallo RL, et al. The microbiome in patients with atopic dermatitis. J Allergy Clin Immunol. 2019;143(1):26-35. [PubMed ID: 30476499]. [PubMed Central ID: PMC7163929]. https://doi.org/10.1016/j.jaci.2018.11.015.
-
3.
Dreno B, Araviiskaia E, Berardesca E, Gontijo G, Sanchez Viera M, Xiang LF, et al. Microbiome in healthy skin, update for dermatologists. J Eur Acad Dermatol Venereol. 2016;30(12):2038-47. [PubMed ID: 27735094]. [PubMed Central ID: PMC6084363]. https://doi.org/10.1111/jdv.13965.
-
4.
Ursell LK, Metcalf JL, Parfrey LW, Knight R. Defining the human microbiome. Nutr Rev. 2012;70 Suppl 1(Suppl 1):S38-44. [PubMed ID: 22861806]. [PubMed Central ID: PMC3426293]. https://doi.org/10.1111/j.1753-4887.2012.00493.x.
-
5.
Glendinning L, Free A. Supra-organismal interactions in the human intestine. Front Cell Infect Microbiol. 2014;4:47. [PubMed ID: 24795867]. [PubMed Central ID: PMC4005949]. https://doi.org/10.3389/fcimb.2014.00047.
-
6.
Byrd AL, Belkaid Y, Segre JA. The human skin microbiome. Nat Rev Microbiol. 2018;16(3):143-55. [PubMed ID: 29332945]. https://doi.org/10.1038/nrmicro.2017.157.
-
7.
Gupta A. Clinical effectiveness of antimicrobial dressings. Antimicrobial Dressings. Elsevier; 2023. p. 203-27.
-
8.
Chen YE, Fischbach MA, Belkaid Y. Skin microbiota-host interactions. Nature. 2018;553(7689):427-36. [PubMed ID: 29364286]. [PubMed Central ID: PMC6075667]. https://doi.org/10.1038/nature25177.
-
9.
Hu C, van Meel ER, Medina-Gomez C, Kraaij R, Barroso M, Kiefte-de Jong J, et al. A population-based study on associations of stool microbiota with atopic diseases in school-age children. J Allergy Clin Immunol. 2021;148(2):612-20. [PubMed ID: 33862008]. https://doi.org/10.1016/j.jaci.2021.04.001.
-
10.
Lee SY, Lee E, Park YM, Hong SJ. Microbiome in the Gut-Skin Axis in Atopic Dermatitis. Allergy Asthma Immunol Res. 2018;10(4):354-62. [PubMed ID: 29949831]. [PubMed Central ID: PMC6021588]. https://doi.org/10.4168/aair.2018.10.4.354.
-
11.
Song H, Yoo Y, Hwang J, Na YC, Kim HS. Faecalibacterium prausnitzii subspecies-level dysbiosis in the human gut microbiome underlying atopic dermatitis. J Allergy Clin Immunol. 2016;137(3):852-60. [PubMed ID: 26431583]. https://doi.org/10.1016/j.jaci.2015.08.021.
-
12.
Lee E, Lee SY, Kang MJ, Kim K, Won S, Kim BJ, et al. Clostridia in the gut and onset of atopic dermatitis via eosinophilic inflammation. Ann Allergy Asthma Immunol. 2016;117(1):91-92 e1. [PubMed ID: 27179583]. https://doi.org/10.1016/j.anai.2016.04.019.
-
13.
Nylund L, Nermes M, Isolauri E, Salminen S, de Vos WM, Satokari R. Severity of atopic disease inversely correlates with intestinal microbiota diversity and butyrate-producing bacteria. Allergy. 2015;70(2):241-4. [PubMed ID: 25413686]. https://doi.org/10.1111/all.12549.
-
14.
Kim MH, Rho M, Choi JP, Choi HI, Park HK, Song WJ, et al. A Metagenomic Analysis Provides a Culture-Independent Pathogen Detection for Atopic Dermatitis. Allergy Asthma Immunol Res. 2017;9(5):453-61. [PubMed ID: 28677360]. [PubMed Central ID: PMC5500701]. https://doi.org/10.4168/aair.2017.9.5.453.
-
15.
Shi B, Bangayan NJ, Curd E, Taylor PA, Gallo RL, Leung DYM, et al. The skin microbiome is different in pediatric versus adult atopic dermatitis. J Allergy Clin Immunol. 2016;138(4):1233-6. [PubMed ID: 27474122]. [PubMed Central ID: PMC5235385]. https://doi.org/10.1016/j.jaci.2016.04.053.
-
16.
Skowron K, Bauza-Kaszewska J, Kraszewska Z, Wiktorczyk-Kapischke N, Grudlewska-Buda K, Kwiecinska-Pirog J, et al. Human Skin Microbiome: Impact of Intrinsic and Extrinsic Factors on Skin Microbiota. Microorganisms. 2021;9(3). [PubMed ID: 33808031]. [PubMed Central ID: PMC7998121]. https://doi.org/10.3390/microorganisms9030543.
-
17.
Jing JLJ, Pei Yi T, Bose RJC, McCarthy JR, Tharmalingam N, Madheswaran T. Hand Sanitizers: A Review on Formulation Aspects, Adverse Effects, and Regulations. Int J Environ Res Public Health. 2020;17(9). [PubMed ID: 32403261]. [PubMed Central ID: PMC7246736]. https://doi.org/10.3390/ijerph17093326.
-
18.
Bandyopadhyay D. Topical Antibacterials in Dermatology. Indian J Dermatol. 2021;66(2):117-25. [PubMed ID: 34188265]. [PubMed Central ID: PMC8208253]. https://doi.org/10.4103/ijd.IJD_99_18.
-
19.
Hartmann AA. Zur in vitro-und in vivo-Untersuchung der Wirkung von Hautreinigungsmitteln auf die Residentflora der Haut des Menschen. Arch Dermatological Res. 1981;275:251-4.
-
20.
SanMiguel AJ, Meisel JS, Horwinski J, Zheng Q, Bradley CW, Grice EA. Antiseptic Agents Elicit Short-Term, Personalized, and Body Site-Specific Shifts in Resident Skin Bacterial Communities. J Invest Dermatol. 2018;138(10):2234-43. [PubMed ID: 29753031]. [PubMed Central ID: PMC6326167]. https://doi.org/10.1016/j.jid.2018.04.022.
-
21.
Bjerre RD, Hugerth LW, Boulund F, Seifert M, Johansen JD, Engstrand L. Effects of sampling strategy and DNA extraction on human skin microbiome investigations. Sci Rep. 2019;9(1):17287.
-
22.
Emerson JB, Adams RI, Roman CMB, Brooks B, Coil DA, Dahlhausen K, et al. Schrodinger's microbes: Tools for distinguishing the living from the dead in microbial ecosystems. Microbiome. 2017;5(1):86. [PubMed ID: 28810907]. [PubMed Central ID: PMC5558654]. https://doi.org/10.1186/s40168-017-0285-3.
-
23.
Kondori N, Tehrani PA, Strombeck L, Faergemann J. Comparison of dermatophyte PCR kit with conventional methods for detection of dermatophytes in skin specimens. Mycopathologia. 2013;176(3-4):237-41. [PubMed ID: 23948965]. https://doi.org/10.1007/s11046-013-9691-7.
-
24.
Yan Q, Gu Y, Li X, Yang W, Jia L, Chen C, et al. Alterations of the Gut Microbiome in Hypertension. Front Cell Infect Microbiol. 2017;7:381. [PubMed ID: 28884091]. [PubMed Central ID: PMC5573791]. https://doi.org/10.3389/fcimb.2017.00381.
-
25.
Artis D, Spits H. The biology of innate lymphoid cells. Nature. 2015;517(7534):293-301. [PubMed ID: 25592534]. https://doi.org/10.1038/nature14189.
-
26.
Cray P, Sheahan BJ, Dekaney CM. Secretory Sorcery: Paneth Cell Control of Intestinal Repair and Homeostasis. Cell Mol Gastroenterol Hepatol. 2021;12(4):1239-50. [PubMed ID: 34153524]. [PubMed Central ID: PMC8446800]. https://doi.org/10.1016/j.jcmgh.2021.06.006.
-
27.
Kaikiri H, Miyamoto J, Kawakami T, Park SB, Kitamura N, Kishino S, et al. Supplemental feeding of a gut microbial metabolite of linoleic acid, 10-hydroxy-cis-12-octadecenoic acid, alleviates spontaneous atopic dermatitis and modulates intestinal microbiota in NC/nga mice. Int J Food Sci Nutr. 2017;68(8):941-51. [PubMed ID: 28438083]. https://doi.org/10.1080/09637486.2017.1318116.
-
28.
Dodd D, Spitzer MH, Van Treuren W, Merrill BD, Hryckowian AJ, Higginbottom SK, et al. A gut bacterial pathway metabolizes aromatic amino acids into nine circulating metabolites. Nature. 2017;551(7682):648-52. [PubMed ID: 29168502]. [PubMed Central ID: PMC5850949]. https://doi.org/10.1038/nature24661.
-
29.
Vandenplas Y, Carnielli VP, Ksiazyk J, Luna MS, Migacheva N, Mosselmans JM, et al. Factors affecting early-life intestinal microbiota development. Nutrition. 2020;78:110812. [PubMed ID: 32464473]. https://doi.org/10.1016/j.nut.2020.110812.
-
30.
Shin H, Pei Z, Martinez K2, Rivera-Vinas JI, Mendez K, Cavallin H, et al. The first microbial environment of infants born by C-section: the operating room microbes. Microbiome. 2015;3:59. [PubMed ID: 26620712]. [PubMed Central ID: PMC4665759]. https://doi.org/10.1186/s40168-015-0126-1.
-
31.
Backhed F, Roswall J, Peng Y, Feng Q, Jia H, Kovatcheva-Datchary P, et al. Dynamics and Stabilization of the Human Gut Microbiome during the First Year of Life. Cell Host Microbe. 2015;17(5):690-703. [PubMed ID: 25974306]. https://doi.org/10.1016/j.chom.2015.04.004.
-
32.
Martin R, Makino H, Cetinyurek Yavuz A, Ben-Amor K, Roelofs M, Ishikawa E, et al. Early-Life Events, Including Mode of Delivery and Type of Feeding, Siblings and Gender, Shape the Developing Gut Microbiota. PLoS One. 2016;11(6). e0158498. [PubMed ID: 27362264]. [PubMed Central ID: PMC4928817]. https://doi.org/10.1371/journal.pone.0158498.
-
33.
Papathoma E, Triga M, Fouzas S, Dimitriou G. Cesarean section delivery and development of food allergy and atopic dermatitis in early childhood. Pediatr Allergy Immunol. 2016;27(4):419-24. [PubMed ID: 26888069]. https://doi.org/10.1111/pai.12552.
-
34.
Bezirtzoglou E, Tsiotsias A, Welling GW. Microbiota profile in feces of breast- and formula-fed newborns by using fluorescence in situ hybridization (FISH). Anaerobe. 2011;17(6):478-82. [PubMed ID: 21497661]. https://doi.org/10.1016/j.anaerobe.2011.03.009.
-
35.
Yi DY, Kim SY. Human Breast Milk Composition and Function in Human Health: From Nutritional Components to Microbiome and MicroRNAs. Nutrients. 2021;13(9). [PubMed ID: 34578971]. [PubMed Central ID: PMC8471419]. https://doi.org/10.3390/nu13093094.
-
36.
Kong WS, Tsuyama N, Inoue H, Guo Y, Mokuda S, Nobukiyo A, et al. Long-chain saturated fatty acids in breast milk are associated with the pathogenesis of atopic dermatitis via induction of inflammatory ILC3s. Sci Rep. 2021;11(1):13109. [PubMed ID: 34162906]. [PubMed Central ID: PMC8222289]. https://doi.org/10.1038/s41598-021-92282-0.
-
37.
Hartmann AA, Feil W, Helling A. Allergieprävention in Schwangerschaft und Stillzeit. Erfahrungsheilkunde. 2008;57(2):77-83.
-
38.
Rosas-Salazar C, Shilts MH, Tang ZZ, Hong Q, Turi KN, Snyder BM, et al. Exclusive breast-feeding, the early-life microbiome and immune response, and common childhood respiratory illnesses. J Allergy Clin Immunol. 2022;150(3):612-21. [PubMed ID: 35283139]. [PubMed Central ID: PMC9463089]. https://doi.org/10.1016/j.jaci.2022.02.023.
-
39.
Dominguez-Bello MG, Costello EK, Contreras M, Magris M, Hidalgo G, Fierer N, et al. Delivery mode shapes the acquisition and structure of the initial microbiota across multiple body habitats in newborns. Proc Natl Acad Sci USA. 2010;107(26):11971-5. [PubMed ID: 20566857]. [PubMed Central ID: PMC2900693]. https://doi.org/10.1073/pnas.1002601107.
-
40.
Chu DM, Ma J, Prince AL, Antony KM, Seferovic MD, Aagaard KM. Maturation of the infant microbiome community structure and function across multiple body sites and in relation to mode of delivery. Nat Med. 2017;23(3):314-26. [PubMed ID: 28112736]. [PubMed Central ID: PMC5345907]. https://doi.org/10.1038/nm.4272.
-
41.
Oh J, Byrd AL, Park M, Kong HH, Segre JA; Nisc Comparative Sequencing Program. Temporal Stability of the Human Skin Microbiome. Cell. 2016;165(4):854-66. [PubMed ID: 27153496]. [PubMed Central ID: PMC4860256]. https://doi.org/10.1016/j.cell.2016.04.008.
-
42.
Casterline BW, Paller AS. Early development of the skin microbiome: therapeutic opportunities. Pediatr Res. 2021;90(4):731-7. [PubMed ID: 32919387]. [PubMed Central ID: PMC7952468]. https://doi.org/10.1038/s41390-020-01146-2.
-
43.
Towell AM, Feuillie C, Vitry P, Da Costa TM, Mathelie-Guinlet M, Kezic S, et al. Staphylococcus aureus binds to the N-terminal region of corneodesmosin to adhere to the stratum corneum in atopic dermatitis. Proc Natl Acad Sci USA. 2021;118(1). [PubMed ID: 33361150]. [PubMed Central ID: PMC7817190]. https://doi.org/10.1073/pnas.2014444118.
-
44.
Nakatsuji T, Chen TH, Narala S, Chun KA, Two AM, Yun T, et al. Antimicrobials from human skin commensal bacteria protect against Staphylococcus aureus and are deficient in atopic dermatitis. Sci Transl Med. 2017;9(378). [PubMed ID: 28228596]. [PubMed Central ID: PMC5600545]. https://doi.org/10.1126/scitranslmed.aah4680.
-
45.
Spaulding AR, Salgado-Pabon W, Kohler PL, Horswill AR, Leung DY, Schlievert PM. Staphylococcal and streptococcal superantigen exotoxins. Clin Microbiol Rev. 2013;26(3):422-47. [PubMed ID: 23824366]. [PubMed Central ID: PMC3719495]. https://doi.org/10.1128/CMR.00104-12.
-
46.
Ott H, Weissmantel S, Kennes LN, Merk HF, Baron JM, Folster-Holst R. Molecular microarray analysis reveals allergen- and exotoxin-specific IgE repertoires in children with atopic dermatitis. J Eur Acad Dermatol Venereol. 2014;28(1):100-7. [PubMed ID: 23305076]. https://doi.org/10.1111/jdv.12083.
-
47.
Blicharz L, Rudnicka L, Samochocki Z. Staphylococcus aureus: an underestimated factor in the pathogenesis of atopic dermatitis? Postepy Dermatol Alergol. 2019;36(1):11-7. [PubMed ID: 30858773]. [PubMed Central ID: PMC6409874]. https://doi.org/10.5114/ada.2019.82821.
-
48.
Byrd AL, Deming C, Cassidy SKB, Harrison OJ, Ng WI, Conlan S, et al. Staphylococcus aureus and Staphylococcus epidermidis strain diversity underlying pediatric atopic dermatitis. Sci Transl Med. 2017;9(397). [PubMed ID: 28679656]. [PubMed Central ID: PMC5706545]. https://doi.org/10.1126/scitranslmed.aal4651.
-
49.
Nowicka D, Grywalska E. The Role of Immune Defects and Colonization of Staphylococcus aureus in the Pathogenesis of Atopic Dermatitis. Anal Cell Pathol (Amst). 2018;2018:1956403. [PubMed ID: 29854575]. [PubMed Central ID: PMC5954962]. https://doi.org/10.1155/2018/1956403.
-
50.
Ong PY, Ohtake T, Brandt C, Strickland I, Boguniewicz M, Ganz T, et al. Endogenous antimicrobial peptides and skin infections in atopic dermatitis. N Engl J Med. 2002;347(15):1151-60. [PubMed ID: 12374875]. https://doi.org/10.1056/NEJMoa021481.
-
51.
Williams MR, Nakatsuji T, Sanford JA, Vrbanac AF, Gallo RL. Staphylococcus aureus Induces Increased Serine Protease Activity in Keratinocytes. J Invest Dermatol. 2017;137(2):377-84. [PubMed ID: 27765722]. [PubMed Central ID: PMC5258850]. https://doi.org/10.1016/j.jid.2016.10.008.
-
52.
Fischer J, Meyer-Hoffert U. Regulation of kallikrein-related peptidases in the skin - from physiology to diseases to therapeutic options. Thromb Haemost. 2013;110(3):442-9. [PubMed ID: 23446429]. https://doi.org/10.1160/TH12-11-0836.
-
53.
Nakagawa S, Matsumoto M, Katayama Y, Oguma R, Wakabayashi S, Nygaard T, et al. Staphylococcus aureus Virulent PSMalpha Peptides Induce Keratinocyte Alarmin Release to Orchestrate IL-17-Dependent Skin Inflammation. Cell Host Microbe. 2017;22(5):667-677 e5. [PubMed ID: 29120744]. [PubMed Central ID: PMC5728420]. https://doi.org/10.1016/j.chom.2017.10.008.
-
54.
Allen HB, Vaze ND, Choi C, Hailu T, Tulbert BH, Cusack CA, et al. The presence and impact of biofilm-producing staphylococci in atopic dermatitis. JAMA Dermatol. 2014;150(3):260-5. [PubMed ID: 24452476]. https://doi.org/10.1001/jamadermatol.2013.8627.
-
55.
Tankersley A, Frank MB, Bebak M, Brennan R. Early effects of Staphylococcus aureus biofilm secreted products on inflammatory responses of human epithelial keratinocytes. J Inflamm (Lond). 2014;11:17. [PubMed ID: 24936153]. [PubMed Central ID: PMC4059087]. https://doi.org/10.1186/1476-9255-11-17.
-
56.
Kong HH, Oh J, Deming C, Conlan S, Grice EA, Beatson MA, et al. Temporal shifts in the skin microbiome associated with disease flares and treatment in children with atopic dermatitis. Genome Res. 2012;22(5):850-9. [PubMed ID: 22310478]. [PubMed Central ID: PMC3337431]. https://doi.org/10.1101/gr.131029.111.
-
57.
Shang L, Deng D, Buskermolen JK, Roffel S, Janus MM, Krom BP, et al. Commensal and Pathogenic Biofilms Alter Toll-Like Receptor Signaling in Reconstructed Human Gingiva. Front Cell Infect Microbiol. 2019;9:282. [PubMed ID: 31448244]. [PubMed Central ID: PMC6692492]. https://doi.org/10.3389/fcimb.2019.00282.
-
58.
Linehan JL, Harrison OJ, Han SJ, Byrd AL, Vujkovic-Cvijin I, Villarino AV, et al. Non-classical Immunity Controls Microbiota Impact on Skin Immunity and Tissue Repair. Cell. 2018;172(4):784-796 e18. [PubMed ID: 29358051]. [PubMed Central ID: PMC6034182]. https://doi.org/10.1016/j.cell.2017.12.033.
-
59.
Thammahong A, Kiatsurayanon C, Edwards SW, Rerknimitr P, Chiewchengchol D. The clinical significance of fungi in atopic dermatitis. Int J Dermatol. 2020;59(8):926-35. [PubMed ID: 32441807]. https://doi.org/10.1111/ijd.14941.
-
60.
Hamdino M, Saudy AA, El-Shahed LH, Taha M. Identification of Malassezia species isolated from some Malassezia associated skin diseases. J Mycol Med. 2022;32(4):101301. [PubMed ID: 35700659]. https://doi.org/10.1016/j.mycmed.2022.101301.
-
61.
Nowicka D, Nawrot U. Contribution of Malassezia spp. to the development of atopic dermatitis. Mycoses. 2019;62(7):588-96. [PubMed ID: 30908750]. https://doi.org/10.1111/myc.12913.
-
62.
Glatz M, Bosshard PP, Hoetzenecker W, Schmid-Grendelmeier P. The Role of Malassezia spp. in Atopic Dermatitis. J Clin Med. 2015;4(6):1217-28. [PubMed ID: 26239555]. [PubMed Central ID: PMC4484996]. https://doi.org/10.3390/jcm4061217.
-
63.
Mittermann I, Wikberg G, Johansson C, Lupinek C, Lundeberg L, Crameri R, et al. IgE Sensitization Profiles Differ between Adult Patients with Severe and Moderate Atopic Dermatitis. PLoS One. 2016;11(5). e0156077. [PubMed ID: 27228091]. [PubMed Central ID: PMC4881900]. https://doi.org/10.1371/journal.pone.0156077.
-
64.
Chen TA, Hill PB. The biology of Malassezia organisms and their ability to induce immune responses and skin disease. Vet Dermatol. 2005;16(1):4-26. [PubMed ID: 15725101]. https://doi.org/10.1111/j.1365-3164.2005.00424.x.
-
65.
Glatz M, Buchner M, von Bartenwerffer W, Schmid-Grendelmeier P, Worm M, Hedderich J, et al. Malassezia spp.-specific immunoglobulin E level is a marker for severity of atopic dermatitis in adults. Acta Derm Venereol. 2015;95(2):191-6. [PubMed ID: 24696225]. https://doi.org/10.2340/00015555-1864.
-
66.
Ivert L. Atopic Dermatitis in Adults: Epidemiological Studies of Comorbidity and Studies of Patients on Systemic Treatment |[thesis]. Sweden: Karolinska Institutet; 2021.
-
67.
Hu L, Zhou M, Young A, Zhao W, Yan Z. In vivo effectiveness and safety of probiotics on prophylaxis and treatment of oral candidiasis: a systematic review and meta-analysis. BMC Oral Health. 2019;19(1):140. [PubMed ID: 31291932]. [PubMed Central ID: PMC6621984]. https://doi.org/10.1186/s12903-019-0841-2.
-
68.
Szczepanska M, Blicharz L, Nowaczyk J, Makowska K, Goldust M, Waskiel-Burnat A, et al. The Role of the Cutaneous Mycobiome in Atopic Dermatitis. J Fungi (Basel). 2022;8(11). [PubMed ID: 36354920]. [PubMed Central ID: PMC9695942]. https://doi.org/10.3390/jof8111153.
-
69.
Kashem SW, Igyarto BZ, Gerami-Nejad M, Kumamoto Y, Mohammed JA, Jarrett E, et al. Candida albicans morphology and dendritic cell subsets determine T helper cell differentiation. Immunity. 2015;42(2):356-66. [PubMed ID: 25680275]. [PubMed Central ID: PMC4343045]. https://doi.org/10.1016/j.immuni.2015.01.008.
-
70.
Svejgaard E, Faergemann J, Jemic G, Kieffer M, Ottevanger V. Recent investigations on the relationship between fungal skin diseases and atopic dermatitis. . Acta Dermato-Venereol. 1989;144:140-2. https://doi.org/10.2340/00015555144140142.
-
71.
Gangneux JP, Godet C, Denning DW. Allergic diseases and fungal exposome: Prevention is better than cure. Allergy. 2022;77(11):3182-4. [PubMed ID: 35822920]. https://doi.org/10.1111/all.15436.
-
72.
Savolainen J, Lammintausta K, Kalimo K, Viander M. Candida albicans and atopic dermatitis. Clin Experimental Allergy. 1993;23(4):332-9. [PubMed ID: 8319131]. https://doi.org/10.1111/j.1365-2222.1993.tb00331.x.
-
73.
Whitley R, Baines J. Clinical management of herpes simplex virus infections: past, present, and future. F1000Res. 2018;7. [PubMed ID: 30443341]. [PubMed Central ID: PMC6213787]. https://doi.org/10.12688/f1000research.16157.1.
-
74.
Ong PY, Leung DY. Bacterial and Viral Infections in Atopic Dermatitis: a Comprehensive Review. Clin Rev Allergy Immunol. 2016;51(3):329-37. [PubMed ID: 27377298]. https://doi.org/10.1007/s12016-016-8548-5.
-
75.
Damour A, Garcia M, Seneschal J, Leveque N, Bodet C. Eczema Herpeticum: Clinical and Pathophysiological Aspects. Clin Rev Allergy Immunol. 2020;59(1):1-18. [PubMed ID: 31836943]. https://doi.org/10.1007/s12016-019-08768-3.
-
76.
Li H, Zhang Z, Zhang H, Guo Y, Yao Z. Update on the Pathogenesis and Therapy of Atopic Dermatitis. Clin Rev Allergy Immunol. 2021;61(3):324-38. [PubMed ID: 34338977]. https://doi.org/10.1007/s12016-021-08880-3.
-
77.
Li L, Han Z, Niu X, Zhang G, Jia Y, Zhang S, et al. Probiotic Supplementation for Prevention of Atopic Dermatitis in Infants and Children: A Systematic Review and Meta-analysis. Am J Clin Dermatol. 2019;20(3):367-77. [PubMed ID: 30465329]. https://doi.org/10.1007/s40257-018-0404-3.
-
78.
Kim K, Lee E, Kim M, Lee KS, Sol IS, Min TK, et al. Therapeutic effectiveness of probiotics for atopic dermatitis: A systematic review and meta-analysis of randomized controlled trials with subgroup analysis. Asian Pac J Allergy Immunol. 2023. [PubMed ID: 37578483]. https://doi.org/10.12932/AP-280323-1576.
-
79.
Sala-Cunill A, Lazaro M, Herraez L, Quinones MD, Moro-Moro M, Sanchez I, et al. Basic Skin Care and Topical Therapies for Atopic Dermatitis: Essential Approaches and Beyond. J Investig Allergol Clin Immunol. 2018;28(6):379-91. [PubMed ID: 30004024]. https://doi.org/10.18176/jiaci.0293.
-
80.
Jin M, Lee S, Choi YA, Jang HJ, Lee SW, Park PH, et al. Lactococcus lactis KR-050L extract suppresses house dust mite induced-atopic skin inflammation through inhibition of keratinocyte and mast cell activation. J Appl Microbiol. 2019;126(1):230-41. [PubMed ID: 30267608]. https://doi.org/10.1111/jam.14116.
-
81.
Harkins CP, Pettigrew KA, Oravcova K, Gardner J, Hearn RMR, Rice D, et al. The Microevolution and Epidemiology of Staphylococcus aureus Colonization during Atopic Eczema Disease Flare. J Invest Dermatol. 2018;138(2):336-43. [PubMed ID: 28951239]. [PubMed Central ID: PMC5780352]. https://doi.org/10.1016/j.jid.2017.09.023.
-
82.
Lossius AH, Sundnes O, Ingham AC, Edslev SM, Bjornholt JV, Lilje B, et al. Shifts in the Skin Microbiota after UVB Treatment in Adult Atopic Dermatitis. Dermatol. 2022;238(1):109-20. [PubMed ID: 33887725]. https://doi.org/10.1159/000515236.