Abstract
Background:
The better performance of elite athletes than others may be due to their better mental and psychological advantages. Transcranial direct current stimulation (tDCS) is an attractive technique to improve the physical and mental functions in recent years.Objectives:
This study aimed to examine the effects of single and dual tDCS of M1 and DLPFC regions on motor and cognitive functions in male gymnasts.Methods:
Sixteen male gymnasts (age mean = 21.70 ± 0.66 years) who were selected randomly, exposed to 4 different intervention conditions in 4 separate sessions with a one-week interval between the sessions, including (1) anodal stimulation of the M1 area; (2) anodal stimulation of the left DLPFC area; (3) simultaneous stimulation of M1 and DLPFC areas; (4) sham stimulation. After ensuring the normal distribution using shapiro-whilk test, gathered data were analyzed with two- way repeated measures ANOVA.Results:
The results showed that single and dual tDCS of M1 and DLPFC regions had a significant effect on improving bimanual coordination, eye-hand coordination, reaction time, working memory, and selective attention of male gymnasts. Also, considering the observed effect size, the M1& DLPFC stimulation condition had a greater effect than other stimulation conditions.Conclusions:
Based on present findings coaches and sports physiologists can improve the performance of their athletes with inclusion single and dual tDCS into their training routines.Keywords
Coordination Reaction Time Selective Attention tDCS Working Memory
1. Background
The exceptional advancement of professional sports in recent years and the unprecedented pressure on athletes to maintain readiness for extended periods and achieve optimal results have sparked an interest in utilizing novel and effective methods and strategies to improve athletic performance (1). The physical characteristics of individuals participating in a particular sport are influenced by many physical, physiological and psychological factors. With the advancement in neuroscience, researchers have started unraveling brain mechanisms (2).
In recent decades, the use of technology in designing training sessions to improve learning capacity and enhance cognitive and motor abilities that affect sports skills and motor tasks, has been increasing (3). In this regard, the use of brain wave electroencephalography (EEG) techniques (4), electromyography (EMG) of neuromuscular activity (5), or transcranial direct current stimulation (tDCS) of the brain has increased (6). The tDCS technique is one of the non-invasive, low-cost methods. Transcranial direct current stimulation passes a direct current in the range of 0.5 to 2 milliamps through the skull using electrodes measuring 25 to 35 square centimeters and modulates the activity of different areas of the brain (7-10). The history of tDCS use dates back to the elucidation of the biological effects of this type of brain stimulation (11). For two centuries, direct current has been used to treat mental disorders (12), and studies in humans and animals have shown that anodal tDCS increases cortical excitability (13). Although the mechanism of tDCS is not clear, this method can increase cortical activity and lead to spontaneous neural firing (3). However, the mechanism of tDCS is not limited to changing the membrane potential of nerve cells and spontaneous neural firing. In fact, some studies have shown that tDCS can cause changes in synaptic function, which is achieved by changing the synaptic power of membrane receptors (14). Also, tDCS affects cortical neurons and corticospinal pathways (15). On the other hand, investigations into the effects of tDCS on motor function have primarily centered on the primary motor cortex, revealing its crucial role in this area (14). Anodal tDCS has been shown to induce long-term potentiation (LTP) in the primary motor cortex, leading to changes in brain plasticity (16). M1 has also been shown to play a role in postural control, as it is part of the prefrontal-basal ganglia network (6). Furthermore, in 2012, Dutta and Chugh demonstrated that 10 minutes of anodal tDCS over M1 can improve static postural stability with eyes closed in young adults (13, 17). Overall, it can be stated that different brain regions play roles in various behavioral and cognitive functions and may even have overlapping effects when these different regions are stimulated simultaneously.
Past studies have reported positive effects of tDCS, but primarily on brain excitability or specific diseases, not athletic performance. Despite the importance of skilled motor and cognitive performance in sports, there is limited research on the effectiveness of tDCS for improving athletic performance and the underlying mechanisms.
2. Objectives
This study aims to address this gap by investigating the immediate effects of single and dual tDCS interventions on the motor and cognitive performance of gymnasts, a sport that demands complex skill acquisition and learning.
3. Methods
3.1. Subjects
The present research was an experimental study within-group design with four conditions. The sample size was calculated using software version 3.1 with 80% statistical power, 35% effect size (Cohen's d), and a significance level of 0.05, and taking into account the statistical test. Working memory (WM) was the primary variable in this research, with the anticipated effect size for determining the sample size based on a recent study conducted in this field (18). According to G*Power output, 13 subjects were deemed adequate for this research; however, considering a 20% dropout rate, 16 male (age mean = 21.70 ± 0.66 years) who attended sports clubs in Kermanshah in 2023, participated voluntarily in this study. None of the subjects had a history of chronic diseases such as cardiovascular diseases, diabetes, various cancers, kidney and digestive disorders, or any injury or problem that would prevent them from participating in physical activities.
3.2. Procedure
The subjects attended the laboratory in 5 separate sessions. The first session was considered to familiarize the subjects with the research process, how to apply brain stimulation, and also the method of measuring the research variables. Following the familiarization session, participants underwent a randomization process, allocating them to four distinct intervention conditions. The research design employed a staggered approach, with subjects exposed to these varying conditions during four separate sessions, each spaced one week apart, including:
- Condition 1: Anodal tDCS of the M1 (a-tDCS-M1) area
- Condition 2: Anodal tDCS of the left DLPFC area (a-tDCS-DLPFC)
- Condition 3: Anodal tDCS of M1 and DLPFC areas (a-tDCS-M1& DLPFC)
- Condition 4: Sham tDCS (s-tDCS).
In each session, the desired variables were measured before and after the application of brain stimulation. During the intervention period, the subjects should not participate in activities that affect the results of the research, and the necessary explanations were given to them in this regard. Participants who received both real and s-tDCS reported only slight itching, mainly due to mild burning.
In the current study, to target specific areas (M1 and DLPFC areas), the 10 - 20 international brain mapping method and a specialized EEG cap were utilized for stimulation. Participants were instructed to sit on a comfortable chair positioned in the predetermined location. Depending on the stimulation type for the session, the target electrode areas were marked using the specialized EEG cap and electrodes were then positioned accordingly. For stimulating the left M1 area, the anode electrode was placed in the C3 area and the cathode electrode on the left shoulder. To stimulate the left DLPFC area, the anode electrode was placed on the F3 area and the cathode electrode on the AF8 area (19). For simultaneous stimulation, the anode electrodes were placed on the C3 (M1) and F3 (DLPFC) areas of the left hemisphere with the cathode electrodes on the supraorbital area (Fp2) of the right hemisphere. During sham stimulation state, the electrode placement method mirrored that of simultaneous stimulation (20).
Participants underwent 20 minutes of 2-milliamperes anodal electrical stimulation, seated, in silence. This double-blind study kept both subjects and primary researchers unaware of stimulation type until completion. A non-team member randomized stimulation orders, and measures hid the tDCS device and procedure from participants' view to ensure they remained unaware of the stimulation protocol. The primary researcher was absent during electrode application and removal, further ensuring participant blinding.
3.3. Data Analysis
Based on the Shapiro-wilk test results, the data for two-handed coordination (THC), eye-hand coordination (EHC), reaction time (RT), WM, and selective attention (SA) were normally distributed (P > 0.05), a two-way (2 time × 4 condition) repeated measures ANOVA was used to analyze the these variables. For pairwaise comparision a one-way ANOVA with repeated measures and paired t-test was used. The significance level of P ≤ 0.05 was considered in all statistical tests. Statistical analysis was performed using SPSS version 26.
4. Results
Two-way ANOVA with repeated measures revealed the interaction effect of conditions × time was significant for all varibles (Table 1). One-way ANOVA with repeated measure results for all variables showed no significant differences between the conditions at the pre-test (P > 0.05). However, during the post-test EHC scores were significantly higher in the condition 2 compared to the condition 3 (P = 0.004) and 1 (P = 0.002). Additionally, RT was significantly lower in the condition 3 compared to the condition 1 (P = 0.030). Furthermore, WM and SA scores in the post-test were significantly better in the condition 3 compared to the conditions 1 (WM: P = 0.026; SA: P=0.005) and 4 (WM: P= 0.021; SA: P < 0.001).
Results of Two-Way ANOVA with Repeated Measures
Variables and Factor | F | DF | P | Effect Size |
---|---|---|---|---|
THC | ||||
Conditions × time | 2.90 | 1.64 | 0.048 | 0.195 |
Time | 0.170 | 1 | 0.688 | 0.014 |
Conditions | 7.512 | 1.678 | 0.005 | 0.385 |
EHC | ||||
Conditions × time | 3.948 | 3 | 0.016 | 0.248 |
Time | 1.634 | 1 | 0.225 | 0.120 |
Conditions | 19.101 | 1.595 | 0.000 | 0.614 |
RT | ||||
Conditions × time | 6.373 | 1.596 | 0.011 | 0.347 |
Time | 2.439 | 1 | 0.144 | 0.169 |
Conditions | 5.517 | 1.316 | 0.025 | 0.315 |
WM | ||||
Conditions × time | 7.782 | 1.878 | 0.003 | 0.393 |
Time | 0.387 | 1 | 0.546 | 0.031 |
Conditions | 10.611 | 1.745 | 0.001 | 0.469 |
SA | ||||
Conditions × time | 14.924 | 1.652 | 0.000 | 0.554 |
Time | 0.773 | 1 | 0.397 | 0.061 |
Conditions | 19.219 | 2.141 | 0.000 | 0.616 |
Paired t-test results showed significant improvements from pre-test to post-test of condition 1 on THC (P < 0.001, Δ = 27.88%, Cohen's d = 1.16), EHC (P = 0.000, Δ = 53.48%, Cohen's d = 1.74), and RT (P = 0.026, Δ = -1.98%, Cohen's d = 0.16). Condition 3 also displayed improvement from pre-test to post-test for THC (P = 0.030, Δ = 7.19%, Cohen's d = 0.44), EHC (P < 0.001, Δ = 20%, Cohen's d = 1.03), and RT (P < 0.001, Δ = -9.98%, Cohen's d = 0.70). The results indicated significant differences in WM from pre-test to post-test for condition 1 (P = 0.030, Δ= 5.23%, d=0.26), condition 2 (P < 0.001, Δ = 16.59%, d = 1.27), condition 3 (P < 0.001, Δ = 19.83%, d = 1.08), and condition 4 (P = 0.028, Δ = 6.33%, d = 0.43). Similarly, a significant difference in SA was observed between the pre-test and post-test for condition 1 (P < 0.001, Δ = -16.75%, d = 0.59), condition 2 (P < 0.001, Δ=-35.61%, d = 0.77), condition 3 (P < 0.001, Δ = -58.31%, d = 1.66), and condition 4 (P < 0.001, Δ = -21.84%, d = 0.67) (Figure 1).
Mean values of the eye-hand coordination (EHC); two-handed coordination (THC); reaction time (RT); working memory (WM) and selective attention (SA) at the specified time points. * = Significant difference with DLPFC; & = Significant difference with M1& DLPFC; # = Significant difference with Sham.
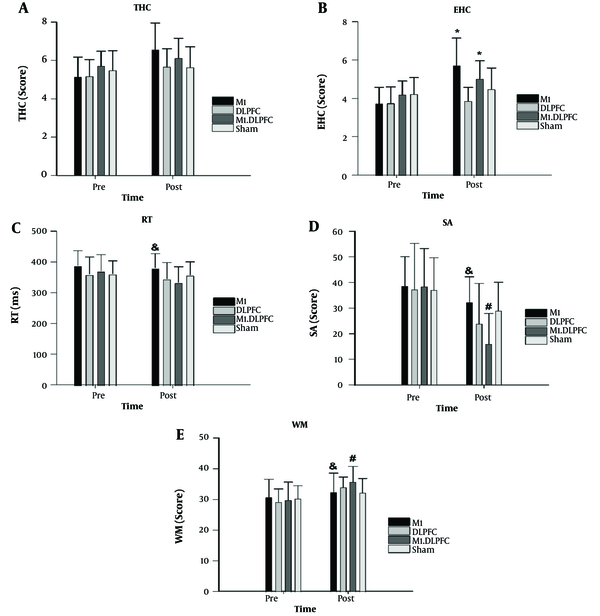
5. Discussion
The present study aimed to investigate the effectiveness of single and dual tDCS of the M1 and DLPFC regions on the motor and cognitive performance of male gymnasts. The findings of this study can be examined from two perspectives. First, the results of this study demonstrated that single and dual tDCS of the M1 and DLPFC regions had a significant impact on improving THC, EHC, RT, WM and SA, leading to enhanced performance and learning among participants. Second, the results of this study indicated that the M1& DLPFC stimulation condition had a greater effect than the other stimulation conditions.
These research findings are in direct agreement with those of Shahbazi et al. (21), Rezaei Sharif et al. (22), and Jin et al. (23), However, they are inconsistent with the results of Seidel and Ragert (24) and Gomes-Osman and Field-Fote (25). The number of stimulation sessions and the research protocol could be among the reasons for this inconsistency. For instance, in the study by Seidel et al. the effect of tDCS on simple RT and tapping was examined among trained football players, handball players, and non-athletes. The purpose of this study was to determine whether trained athletes, compared to non-athletes, demonstrate more effective tDCS-related gains. The findings revealed that tDCS had no effect on simple RT and tapping at various time points examined among athletes and non-athletes (24). However, indirect studies in this field have shown that DLPFC stimulation maintains alertness and attention (26). Transcranial direct current stimulation of the DLPFC region has also been linked to improvements in visual search (27). In this regard, McIntire et al. (28) suggest that tDCS stimulation initially increases and then maintains alertness and attention performance, which is consistent with the findings of the present study.
In terms of the effectiveness of these exercises, it can be argued that electrical brain stimulation of the cerebellum and motor cortex increases the excitability of brain cells, accelerates the process of neural plasticity, and leads to improved motor learning (29). Additionally, during motor learning, functional and structural changes occur in certain neural networks, such as the M1 and DLPFC, altering the behavioral and neural relationships associated with motor learning.
It is proposed that the beneficial effects of tDCS on motor learning may be related to the strengthening of these neural networks and the enhancement of physiological and cellular changes that occur with practice, particularly since it can reduce the levels of inhibitory neurotransmitters (such as gamma-aminobutyric acid) or increase the levels of excitatory neurotransmitters and facilitate motor performance and learning by improving parameters that enhance motor cortex excitability (30). Another possible effect of tDCS is the increase in BDNF levels as a result of stimulation, which can aid in neural potential storage and enhance motor learning (31).
The findings of the present study have significant implications. First, gymnasts can achieve better THC, EHC, RT, WM, SA and overall performance and learning, which can directly compensate for training condition-related inconsistencies. Second, the guidelines for single and dual tDCS of the M1 and DLPFC regions improve the motor and cognitive performance of male gymnasts. Therefore, these findings have specific applications in the abilities of gymnasts and other sports disciplines where skilled cognitive and motor performance are reciprocally influenced by psychological and physiological stresses. Consequently, it is suggested that sports coaches and physiologists further enhance the athletic performance and learning of their athletes through the application of single and dual tDCS.
The study had limitations, including a small sample size, which prevents conclusions about the effectiveness of single-site or dual-site conditions on motor learning. Future research should focus on long-term effects and investigate learning outcomes over a longer period. The variation in tDCS stimulation features has also made it challenging to design standardized protocols.
Generally, the results suggest that single-area stimulation of the M1 region is the most effective method for enhancing motor performance. Transcranial direct current stimulation may reduce inhibitory neurotransmitters or increase stimulated neurotransmitters, improving excitability and facilitating motor skills (32). Stimulating the primary motor cortex increases output and improves performance. Additionally, stimulating both the M1 and DLPFC has a positive impact on athletes' cognitive abilities, modulating a range of functions from attention to WM (33, 34).
Acknowledgements
References
-
1.
Gazerani P. Performance Enhancement by Brain Stimulation. J Sports Sci Med. 2017;16(3):438-9. [PubMed ID: 28912663]. [PubMed Central ID: PMC5592297].
-
2.
Hu K, Chen Y, Guo F, Wang X. Effects of Transcranial Direct Current Stimulation on Upper Limb Muscle Strength and Endurance in Healthy Individuals: A Systematic Review and Meta-Analysis. Front Physiol. 2022;13:834397. [PubMed ID: 35356085]. [PubMed Central ID: PMC8959826]. https://doi.org/10.3389/fphys.2022.834397.
-
3.
Wing VC, Barr MS, Wass CE, Lipsman N, Lozano AM, Daskalakis ZJ, et al. Brain stimulation methods to treat tobacco addiction. Brain Stimul. 2013;6(3):221-30. [PubMed ID: 22809824]. https://doi.org/10.1016/j.brs.2012.06.008.
-
4.
Miniussi C, Cappa SF, Cohen LG, Floel A, Fregni F, Nitsche MA, et al. Efficacy of repetitive transcranial magnetic stimulation/transcranial direct current stimulation in cognitive neurorehabilitation. Brain Stimul. 2008;1(4):326-36. [PubMed ID: 20633391]. https://doi.org/10.1016/j.brs.2008.07.002.
-
5.
Ko MH, Han SH, Park SH, Seo JH, Kim YH. Improvement of visual scanning after DC brain polarization of parietal cortex in stroke patients with spatial neglect. Neurosci Lett. 2008;448(2):171-4. [PubMed ID: 18952147]. https://doi.org/10.1016/j.neulet.2008.10.050.
-
6.
Demain A, Westby GW, Fernandez-Vidal S, Karachi C, Bonneville F, Do MC, et al. High-level gait and balance disorders in the elderly: a midbrain disease? J Neurol. 2014;261(1):196-206. [PubMed ID: 24202784]. [PubMed Central ID: PMC3895186]. https://doi.org/10.1007/s00415-013-7174-x.
-
7.
Rajji TK, Rogasch NC, Daskalakis ZJ, Fitzgerald PB. Neuroplasticity-based brain stimulation interventions in the study and treatment of schizophrenia: a review. Can J Psychiatry. 2013;58(2):93-8. [PubMed ID: 23442896]. https://doi.org/10.1177/070674371305800206.
-
8.
Guleyupoglu B, Schestatsky P, Edwards D, Fregni F, Bikson M. Classification of methods in transcranial electrical stimulation (tES) and evolving strategy from historical approaches to contemporary innovations. J Neurosci Methods. 2013;219(2):297-311. [PubMed ID: 23954780]. [PubMed Central ID: PMC3833074]. https://doi.org/10.1016/j.jneumeth.2013.07.016.
-
9.
Brunoni AR, Nitsche MA, Bolognini N, Bikson M, Wagner T, Merabet L, et al. Clinical research with transcranial direct current stimulation (tDCS): challenges and future directions. Brain Stimul. 2012;5(3):175-95. [PubMed ID: 22037126]. [PubMed Central ID: PMC3270156]. https://doi.org/10.1016/j.brs.2011.03.002.
-
10.
Nitsche MA, Cohen LG, Wassermann EM, Priori A, Lang N, Antal A, et al. Transcranial direct current stimulation: State of the art 2008. Brain Stimul. 2008;1(3):206-23. [PubMed ID: 20633386]. https://doi.org/10.1016/j.brs.2008.06.004.
-
11.
Giordano J, Bikson M, Kappenman ES, Clark VP, Coslett HB, Hamblin MR, et al. Mechanisms and Effects of Transcranial Direct Current Stimulation. Dose Response. 2017;15(1):1559325816685470. [PubMed ID: 28210202]. [PubMed Central ID: PMC5302097]. https://doi.org/10.1177/1559325816685467.
-
12.
Agarwal SM, Shivakumar V, Bose A, Subramaniam A, Nawani H, Chhabra H, et al. Transcranial direct current stimulation in schizophrenia. Clin Psychopharmacol Neurosci. 2013;11(3):118-25. [PubMed ID: 24465247]. [PubMed Central ID: PMC3897759]. https://doi.org/10.9758/cpn.2013.11.3.118.
-
13.
Xiao S, Wang B, Zhang X, Zhou J, Fu W. Acute Effects of High-Definition Transcranial Direct Current Stimulation on Foot Muscle Strength, Passive Ankle Kinesthesia, and Static Balance: A Pilot Study. Brain Sci. 2020;10(4). [PubMed ID: 32326228]. [PubMed Central ID: PMC7226500]. https://doi.org/10.3390/brainsci10040246.
-
14.
Nitsche MA, Paulus W. Excitability changes induced in the human motor cortex by weak transcranial direct current stimulation. J Physiol. 2000;527 Pt 3(Pt 3):633-9. [PubMed ID: 10990547]. [PubMed Central ID: PMC2270099]. https://doi.org/10.1111/j.1469-7793.2000.t01-1-00633.x.
-
15.
Clark VP, Coffman BA, Trumbo MC, Gasparovic C. Transcranial direct current stimulation (tDCS) produces localized and specific alterations in neurochemistry: a (1)H magnetic resonance spectroscopy study. Neurosci Lett. 2011;500(1):67-71. [PubMed ID: 21683766]. https://doi.org/10.1016/j.neulet.2011.05.244.
-
16.
Goodwill AM, Reynolds J, Daly RM, Kidgell DJ. Formation of cortical plasticity in older adults following tDCS and motor training. Front Aging Neurosci. 2013;5:87. [PubMed ID: 24367333]. [PubMed Central ID: PMC3854104]. https://doi.org/10.3389/fnagi.2013.00087.
-
17.
Dutta A, Chugh S. Effect of transcranial direct current stimulation on cortico-muscular coherence and standing postural steadiness. The 2nd IASTED International Conference on Assistive Technologies. Cambridge, USA. ACTA Press Innsbruck; 2012.
-
18.
Baumert A, Buchholz N, Zinkernagel A, Clarke P, MacLeod C, Osinsky R, et al. Causal underpinnings of working memory and Stroop interference control: Testing the effects of anodal and cathodal tDCS over the left DLPFC. Cogn Affect Behav Neurosci. 2020;20(1):34-48. [PubMed ID: 31183619]. [PubMed Central ID: PMC7012981]. https://doi.org/10.3758/s13415-019-00726-y.
-
19.
Cowan N. Working Memory Underpins Cognitive Development, Learning, and Education. Educ Psychol Rev. 2014;26(2):197-223. [PubMed ID: 25346585]. [PubMed Central ID: PMC4207727]. https://doi.org/10.1007/s10648-013-9246-y.
-
20.
Leclercq M, Zimmerman P. Applied neuropsychology of attention: theory, diagnosis, and rehabilitation. New York, USA: Psychology Press; 2002.
-
21.
Shahbazi N, Heyrani A, Amiri E, Gomez Da Silva Machado D. The effect of virtual reality with and without transcranial direct current stimulation on the balance performance of sedentary adolescent girls. Monthly J Sport Psychol Stud. 2023;15(3):113-32.
-
22.
Rezaei Sharif A, Taghizadeh Hir S, Fattahzadeh Ardalani G. The Effectiveness of Transcranial Direct Current Stimulation (tDCS) on Improving Cognitive Abilities in Martial Athletes. J Police Med. 2023;12(1):1-13. eng. https://doi.org/10.30505/12.1.3.
-
23.
Jin Y, Lee J, Kim S, Yoon B. Noninvasive brain stimulation over M1 and DLPFC cortex enhances the learning of bimanual isometric force control. Hum Movement Sci. 2019;1(66):73-83.
-
24.
Seidel O, Ragert P. Effects of Transcranial Direct Current Stimulation of Primary Motor Cortex on Reaction Time and Tapping Performance: A Comparison Between Athletes and Non-athletes. Front Hum Neurosci. 2019;13:103. [PubMed ID: 31024275]. [PubMed Central ID: PMC6460944]. https://doi.org/10.3389/fnhum.2019.00103.
-
25.
Gomes-Osman J, Field-Fote EC. Bihemispheric anodal corticomotor stimulation using transcranial direct current stimulation improves bimanual typing task performance. J Mot Behav. 2013;45(4):361-7. [PubMed ID: 23796102]. https://doi.org/10.1080/00222895.2013.808604.
-
26.
McKinley RA, McIntire L, Bridges N, Goodyear C, Bangera NB, Weisend MP. Acceleration of image analyst training with transcranial direct current stimulation. Behav Neurosci. 2013;127(6):936-46. [PubMed ID: 24341718]. https://doi.org/10.1037/a0034975.
-
27.
Bolognini N, Fregni F, Casati C, Olgiati E, Vallar G. Brain polarization of parietal cortex augments training-induced improvement of visual exploratory and attentional skills. Brain Res. 2010;1349:76-89. [PubMed ID: 20599813]. https://doi.org/10.1016/j.brainres.2010.06.053.
-
28.
McIntire LK, McKinley RA, Goodyear C, Nelson J. A comparison of the effects of transcranial direct current stimulation and caffeine on vigilance and cognitive performance during extended wakefulness. Brain Stimul. 2014;7(4):499-507. [PubMed ID: 25047826]. https://doi.org/10.1016/j.brs.2014.04.008.
-
29.
Stagg CJ, Jayaram G, Pastor D, Kincses ZT, Matthews PM, Johansen-Berg H. Polarity and timing-dependent effects of transcranial direct current stimulation in explicit motor learning. Neuropsychol. 2011;49(5):800-4. [PubMed ID: 21335013]. [PubMed Central ID: PMC3083512]. https://doi.org/10.1016/j.neuropsychologia.2011.02.009.
-
30.
Honarmand P, Gharayagh Zandi H, Moghadam Zadeh A, Rostami R. The effect of direct transcranial electrical stimulation (tDCS) in the DLPFC area on performance and visual attention of sitting volleyball players under stressor. J Psychol Sci. 2022;21(112):647-62. eng. https://doi.org/10.52547/jps.21.112.647.
-
31.
Butefisch CM, Khurana V, Kopylev L, Cohen LG. Enhancing encoding of a motor memory in the primary motor cortex by cortical stimulation. J Neurophysiol. 2004;91(5):2110-6. [PubMed ID: 14711974]. https://doi.org/10.1152/jn.01038.2003.
-
32.
Ungerleider LG, Doyon J, Karni A. Imaging brain plasticity during motor skill learning. Neurobiol Learn Mem. 2002;78(3):553-64. [PubMed ID: 12559834]. https://doi.org/10.1006/nlme.2002.4091.
-
33.
Ahissar E, Sosnik R, Bagdasarian K, Haidarliu S. Temporal frequency of whisker movement. II. Laminar organization of cortical representations. J Neurophysiol. 2001;86(1):354-67. [PubMed ID: 11431516]. https://doi.org/10.1152/jn.2001.86.1.354.
-
34.
Hunter MA, Coffman BA, Gasparovic C, Calhoun VD, Trumbo MC, Clark VP. Baseline effects of transcranial direct current stimulation on glutamatergic neurotransmission and large-scale network connectivity. Brain Res. 2015;1594:92-107. [PubMed ID: 25312829]. [PubMed Central ID: PMC4358793]. https://doi.org/10.1016/j.brainres.2014.09.066.