Keywords
Melanoma Cancer Extracellular Vesicles Tumor Microenvironment
Dear Editor,
Melanoma is a malignant tumor that originates from melanocytes, constituting roughly 5% of the total tumor count (1). Melanoma primarily affects the skin but can also manifest in the mucosal surface, eyes, and meninges. Melanoma is a form of skin cancer characterized by the uncontrolled division of melanocytes, which are cells responsible for producing pigment. Melanomas can occur anywhere on the skin, with some areas being more susceptible to this type of cancer. Melanoma tends to primarily impact the chest and back in men, the legs in women, and the face, which is another common location for this type of skin cancer.
Melanoma manifests in four primary forms: Superficial spreading, nodular, lentigo maligna, and acral lentiginous melanomas. The superficial extension form is the most common, representing about 70% of melanomas, followed by the nodular form, accounting for 15 - 30% of cases. The less common forms of melanoma, including lentigo maligna and acral lentiginous, make up less than 10% of melanoma cases (2).
Although melanoma is relatively uncommon, it is the primary reason for skin cancer-related deaths due to its aggressive metastatic nature. Although the sentinel lymph node (SLN) is commonly identified as the primary site of initial metastasis, melanoma cells also tend to spread to distant regions and organs in the body (3). This dissemination to distant sites contributes to the advanced and often fatal stages of the disease.
Melanoma incidence has increased in fair-skinned countries over the past 70 years; nevertheless, mortality rates have remained stable. Improved awareness, early detection, and advancements in treatment have contributed to better outcomes and reduced mortality. However, prevention efforts and regular screenings are still essential in combating this form of skin cancer (4).
The likelihood of survival greatly improves with early diagnosis and advancements in treatment. When melanoma is detected at an early stage and remains localized, the 5-year survival probability is quite high. However, as the disease progresses to advanced stages with distant metastasis, the survival probability significantly decreases. The National Cancer Institute’s 5-year survival rate is 99.5% for localized cases and 31.9% for cases that have metastasized to distant sites.
Surgery is the main treatment for melanoma. Radiotherapy and chemotherapy are used for advanced cases; however, they have limitations and potential side effects. Newer treatments, such as immunotherapy and targeted therapies, offer promising alternatives. It is important to consult with healthcare providers for the best treatment option.
In recent years, targeted therapies and immunotherapy have emerged as alternative treatments for melanoma. Targeted therapies address molecular defects; however, resistance often develops.
Extracellular vesicles (EVs) play crucial roles in melanoma biology by enabling intercellular communication. They provide insights for diagnosis, prognosis, and potential therapies. The cargo of EVs, including proteins and ribonucleic acid (RNA), can act as diagnostic biomarkers and targets for therapy. Therefore, they have become a significant focus in melanoma research for advancing personalized medicine (5).
Extracellular vesicles are vesicles released by cells into the extracellular space. They can be categorized into three major types: Microvesicles, exosomes, and apoptotic bodies. These EVs are lipid membrane-bound nanoparticles produced by cells. They possess distinct characteristics and play crucial roles in facilitating communication between cells, and they can be categorized into subtypes based on size, origin, biochemical components, and physiological conditions. These diverse EV subtypes contribute to the complexity of intercellular communication and have potential diagnostic and therapeutic applications (6).
Recent technological advances have improved the classification of EVs using different markers, enhancing our understanding of their heterogeneity and their role in intercellular communication. Extracellular vesicles contain various biomolecules emitted from cells, such as nucleic acids, proteins, and lipids. By transferring this cargo to recipient cells, EVs can induce malignant phenotype changes, such as enhanced invasion, angiogenesis, tumor cell migration, and impaired tumor immunity (7, 8).
Extracellular vesicles, particularly exosomes, show immense promise as carriers for drug delivery. Their inherent properties, such as efficient material transportation, circulation stability, extended half-lives, and excellent biocompatibility, make them well-suited for delivering therapeutic agents (9).
Extracellular vesicle-based drug formulations offer a potent and innovative delivery platform for anti-cancer and anti-inflammatory therapies (10). Extracellular vesicles have shown the ability to both suppress and activate the immune response in various studies.
In advanced cancer, tumor cells develop strategies to evade the immune system, and these immune suppressive factors are also present in EVs. Nevertheless, during the early stages of cancer, EVs express tumor antigens capable of stimulating and activating the immune response. However, it is important to consider that the variability in these findings could be influenced by the specific experimental conditions employed in each study and the developmental stage of the chosen cells (11).
The tumor microenvironment (TME) is composed of a variety of cells, such as immune cells, tumor cells, and stromal cells. It plays a vital role in melanoma development, influencing both tumor immunity and prognosis. The interaction between melanoma cells and other cells within the TME plays a crucial role in facilitating these effects (12).
Emerging evidence suggests a close relationship between EVs and the TME in melanoma and other cancers. Extracellular vesicles play a significant role in driving tumor progression within the TME by influencing various processes involved in cancer development and metastasis (Figure 1) (8).
Extracellular vesicles (EVs) originating from cancerous cells, such as exosomes, microvesicles, and apoptotic bodies, can transport diverse cargoes to the tumor microenvironment and impact the immune system’s response involving T, B, and NK cells.
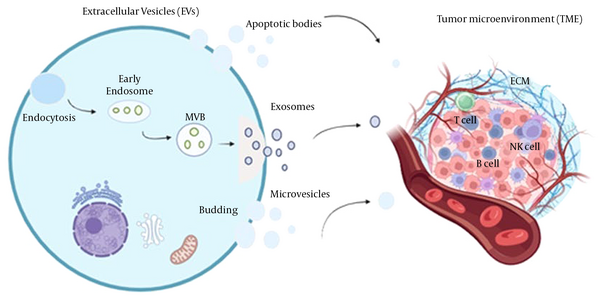
In a study by Palmulli et al. (13), it was discovered that melanoma-derived EVs can physically interact with collagen I, a key component of the extracellular matrix (ECM). This interaction enhances the action of surface metalloproteinases on the EVs, leading to ECM remodeling and promoting tumor progression.
Inhibiting the linking process between EVs derived from tumors and collagen I could be a potential remedial approach for melanoma. Nevertheless, further research is needed to understand if EVs can bind to other ECM components. Matrix metalloproteinases (MMPs) are enzymes that rely on zinc and are implicated in tissue remodeling and tumor progression (14, 15).
Mesenchymal stem cells (MSCs) are highly versatile cells that possess self-renewal abilities and can differentiate into different cell types in response to specific signals (16). Exosomes obtained from MSCs have been identified as potential carriers for diagnostic biomarkers. Mesenchymal stem cells have also shown promise in promoting therapies in both clinical and pre-clinical studies. The release of exosomes from MSCs plays a vital role in their paracrine impact, which is responsible for the trophic effects observed in regenerative medicine.
Previous studies have shown that human cord blood-derived MSCs can reduce inflammatory disorders and localized cerebral ischemia. Recent research also suggests that human embryonic stem cells have the potential to transform the tumor-promoting microenvironment of melanoma cells into a less malignant state by inhibiting cell proliferation and inducing apoptosis (17). Treatment with exosomes derived from cord blood stem cells (CBSC) resulted in a dose- and time-dependent decrease in cell survival for cancer cells (CHL-1 cells) and melanoma patient lymphocytes. These exosomes demonstrated genotoxicity in cancer cells and melanoma patient lymphocytes while showing no cytotoxicity in healthy volunteer lymphocytes. Cord blood exosomes have the potential to inhibit tumor formation by containing specific microRNAs that can reduce the tumorigenicity and growth of cancer cells.
In addition to the main types of EVs, there are other variations, such as amphisomes, exomeres, ectosomes, and oncosomes. Melanoma cells also release melanosomes, structures similar to EVs, which help transport pigment to the skin’s outer layer (18).
The population of EVs is incredibly diverse, just like their roles. Extracellular vesicle research has flourished in recent years, expanding our knowledge extensively. The classification of EVs continuously adapts to keep up with this growing field. Cancer cells release a high number of EVs due to their increased metabolic activity. These EVs, reflecting their parent cells, have varying functions depending on the stage of cancer, making them particularly captivating in cancer immunotherapy. Tumor-derived EVs contain tumor-associated antigens (TAA) and tumor-specific antigens (TSA). T cells recognize these antigens, and dendritic cells (DCs) help activate cytotoxic T lymphocytes (CTLs). These cells have shown promise as cancer vaccines in both laboratory studies and living organisms. Cancer cells utilize EVs to create an immunosuppressive environment, both at the tumor site and potential metastatic sites. Finding ways to block this inhibition and enhance immune-stimulatory effects is critical for the development of effective tumor-specific cancer vaccines (19, 20).
Extracellular vesicles are valuable for studying and diagnosing cancer, evaluating treatment responses, and developing new therapies. Their dual ability to promote both pro- and anti-tumor effects makes them a powerful tool in cancer research and treatment. Due to the characteristics of EVs, they can be used as nanosized carriers designed for drug delivery and regenerative medicine. Extracellular vesicles can be a suitable alternative as a new treatment option.
References
-
1.
Falcone I, Conciatori F, Bazzichetto C, Ferretti G, Cognetti F, Ciuffreda L, et al. Tumor Microenvironment: Implications in Melanoma Resistance to Targeted Therapy and Immunotherapy. Cancers (Basel). 2020;12(10). [PubMed ID: 33036192]. [PubMed Central ID: PMC7601592]. https://doi.org/10.3390/cancers12102870.
-
2.
Liu Y, Sheikh MS. Melanoma: Molecular pathogenesis and therapeutic management. Mol Cell Pharmacol. 2014;6(3):228. [PubMed ID: 25745537]. [PubMed Central ID: PMC4346328].
-
3.
Garcia-Silva S, Benito-Martin A, Nogues L, Hernandez-Barranco A, Mazariegos MS, Santos V, et al. Melanoma-derived small extracellular vesicles induce lymphangiogenesis and metastasis through an NGFR-dependent mechanism. Nat Cancer. 2021;2(12):1387-405. [PubMed ID: 34957415]. [PubMed Central ID: PMC8697753]. https://doi.org/10.1038/s43018-021-00272-y.
-
4.
Saginala K, Barsouk A, Aluru JS, Rawla P, Barsouk A. Epidemiology of Melanoma. Med Sci (Basel). 2021;9(4). [PubMed ID: 34698235]. [PubMed Central ID: PMC8544364]. https://doi.org/10.3390/medsci9040063.
-
5.
van Niel G, Carter DRF, Clayton A, Lambert DW, Raposo G, Vader P. Challenges and directions in studying cell-cell communication by extracellular vesicles. Nat Rev Mol Cell Biol. 2022;23(5):369-82. [PubMed ID: 35260831]. https://doi.org/10.1038/s41580-022-00460-3.
-
6.
Doyle LM, Wang MZ. Overview of extracellular vesicles, their origin, composition, purpose, and methods for exosome isolation and analysis. Cells. 2019;8(7). [PubMed ID: 31311206]. [PubMed Central ID: PMC6678302]. https://doi.org/10.3390/cells8070727.
-
7.
Sheehan C, D'Souza-Schorey C. Tumor-derived extracellular vesicles: molecular parcels that enable regulation of the immune response in cancer. J Cell Sci. 2019;132(20). [PubMed ID: 31615844]. [PubMed Central ID: PMC6826013]. https://doi.org/10.1242/jcs.235085.
-
8.
Marar C, Starich B, Wirtz D. Extracellular vesicles in immunomodulation and tumor progression. Nat Immunol. 2021;22(5):560-70. [PubMed ID: 33753940]. [PubMed Central ID: PMC9389600]. https://doi.org/10.1038/s41590-021-00899-0.
-
9.
Elsharkasy OM, Nordin JZ, Hagey DW, de Jong OG, Schiffelers RM, Andaloussi SE, et al. Extracellular vesicles as drug delivery systems: Why and how? Adv Drug Deliv Rev. 2020;159:332-43. [PubMed ID: 32305351]. https://doi.org/10.1016/j.addr.2020.04.004.
-
10.
Kim MS, Haney MJ, Zhao Y, Yuan D, Deygen I, Klyachko NL, et al. Engineering macrophage-derived exosomes for targeted paclitaxel delivery to pulmonary metastases: in vitro and in vivo evaluations. Nanomedicine. 2018;14(1):195-204. [PubMed ID: 28982587]. https://doi.org/10.1016/j.nano.2017.09.011.
-
11.
Wang L, Sun Z, Wang H. Extracellular vesicles and the regulation of tumor immunity: Current progress and future directions. J Cell Biochem. 2021;122(7):760-9. [PubMed ID: 33594754]. https://doi.org/10.1002/jcb.29904.
-
12.
Alvarez-Breckenridge C, Markson SC, Stocking JH, Nayyar N, Lastrapes M, Strickland MR, et al. Microenvironmental landscape of human melanoma brain metastases in response to immune checkpoint inhibition. Cancer Immunol Res. 2022;10(8):996-1012. [PubMed ID: 35706413]. [PubMed Central ID: PMC10201927]. https://doi.org/10.1158/2326-6066.CIR-21-0870.
-
13.
Palmulli R, Bresteau E, Raposo G, Montagnac G, van Niel G. In vitro interaction of melanoma-derived extracellular vesicles with collagen. Int J Mol Sci. 2023;24(4). [PubMed ID: 36835115]. [PubMed Central ID: PMC9964759]. https://doi.org/10.3390/ijms24043703.
-
14.
Carey P, Low E, Harper E, Stack MS. Metalloproteinases in ovarian cancer. Int J Mol Sci. 2021;22(7). [PubMed ID: 33810259]. [PubMed Central ID: PMC8036623]. https://doi.org/10.3390/ijms22073403.
-
15.
Scheau C, Badarau IA, Costache R, Caruntu C, Mihai GL, Didilescu AC, et al. The role of matrix metalloproteinases in the epithelial-mesenchymal transition of hepatocellular carcinoma. Anal Cell Pathol (Amst). 2019;2019:9423907. [PubMed ID: 31886121]. [PubMed Central ID: PMC6899323]. https://doi.org/10.1155/2019/9423907.
-
16.
Kim YJ, Yoo SM, Park HH, Lim HJ, Kim YL, Lee S, et al. Exosomes derived from human umbilical cord blood mesenchymal stem cells stimulates rejuvenation of human skin. Biochem Biophys Res Commun. 2017;493(2):1102-8. [PubMed ID: 28919421]. https://doi.org/10.1016/j.bbrc.2017.09.056.
-
17.
Li X, Liu L, Yang J, Yu Y, Chai J, Wang L, et al. Exosome derived from human umbilical cord mesenchymal stem cell mediates mir-181c attenuating burn-induced excessive inflammation. EBioMedicine. 2016;8:72-82. [PubMed ID: 27428420]. [PubMed Central ID: PMC4919539]. https://doi.org/10.1016/j.ebiom.2016.04.030.
-
18.
Lattmann E, Levesque MP. The role of extracellular vesicles in melanoma progression. Cancers (Basel). 2022;14(13). [PubMed ID: 35804857]. [PubMed Central ID: PMC9264817]. https://doi.org/10.3390/cancers14133086.
-
19.
Giacobino C, Canta M, Fornaguera C, Borros S, Cauda V. Extracellular vesicles and their current role in cancer immunotherapy. Cancers (Basel). 2021;13(9). [PubMed ID: 34068657]. [PubMed Central ID: PMC8126043]. https://doi.org/10.3390/cancers13092280.
-
20.
Wolfers J, Lozier A, Raposo G, Regnault A, Thery C, Masurier C, et al. Tumor-derived exosomes are a source of shared tumor rejection antigens for CTL cross-priming. Nat Med. 2001;7(3):297-303. [PubMed ID: 11231627]. https://doi.org/10.1038/85438.