Abstract
Background:
Exposure to different frequencies would have a profound effect on the brain tissue extract supernatant’s optical density and hexakis-2-methoxyisobutylisonitrile (99mTc-MIBI) build-up.Objectives:
This study aimed to investigate the blood-brain barrier permeability after electromagnetic field irradiation.Methods:
In this study, 150 male albino rats were randomly divided into 25 groups. They had an intraperitoneal injection of Evans blue 24 hours before experiments. Before 15 or 30-min Electromagnetic Field (EMF) irradiation (900, 1700, and 1900 MHz), 500 μCi (18.5 MBq) of 99mTc -MIBI was injected via the caudal vein. The rats decapitated 15, 30, 45, or 60 min after radiotracer injection to evaluate the brain-specific activity and brain tissue extract supernatant’s optical density.Results:
No significant difference was found between the groups in 60-min post-injection activity after 15-min EMF radiation at 900, 1700, and 1900 MHz. The brain-specific activity with 30-min EMF radiation showed a significant increase in the 900 MHz group compared to others. Brain/whole body-specific activity in all experimental groups after 15-min EMF radiation showed no significant differences. On the other hand, the brain/whole body-specific activity ratio with 30-min EMF radiation showed a significant increase in the 900 MHz group compared to other groups. A comparison between the brain tissue extract supernatant’s optical density in the experimental groups after 15 and 30-min EMF radiation showed no significant difference in Evans blue accumulations in brains.Conclusions:
It may be concluded that mobile phone electromagnetic field radiation may increase the blood-brain barrier permeability. As most humans use mobile phones for daily communications, all users around the world need to be advised of the effects of modern call devices.Keywords
1. Background
Mobile phones were introduced into the market in the 1970s. The popular average mobile phone call expected to six minutes as a safe time with no increase in brain temperature (1). Although the dependence on mobile phones is growing at an alarming rate, the biological effects of exposure to Electromagnetic Field (EMF) have become a global issue. Non-ionizing radiation of mobile phones can lead to the movement of electric charges and the conversion of energy into heat in the environment. The amount of energy absorbed by the brain depends on many factors such as the time of conversation, the location of calls, the number of calls, the frequency band, and the type of mobile device (2). The EMF of mobile phones can cause headaches, changes in sleep patterns, modifications in the electroencephalogram, and increases in the activities of nitric oxide, malondialdehyde, xanthine oxidase, and adenosine deaminase (3).
One of the biological effects of EMF is the permeability change in the Blood-Brain Barrier (BBB), which was discovered by E.E. Goldman in 1919. The BBB is crucial for maintaining the homeostasis of the brain in the human. The key functioning site is the tight junction that eliminates nearly all exogenous materials from the brain for its regular function (4). Several studies have explored the effect of EMF on the permeability of BBB. In some studies, researchers found increased BBB permeability to albumin in the brain with exposure to the 900 MHz band (5-7). Several studies have used Evans blue as a low molecular mass indicator to evaluate BBB permeability following EMF exposure (8-12), as its leakage into the brain parenchyma indicates BBB disruption.
99mTc Hexakis 2-Methoxyisobutyl Isonitrile (99mTc-MIBI) was developed as an agent for myocardial perfusion studies. According to bio-distribution studies, 99mTc-MIBI also can accumulate in negative plasma membrane tissues through high mitochondrial contents, including the heart, liver, kidney, and skeletal muscle tissue (13). MIBI uptake in brain tumors affected by the defect of the BBB (14). but it was not absorbed in the normal brain due to the presence of BBB (15).
2. Objectives
In this study, we examined the role of EMF radiation (frequency bands of 900, 1700, and 1900 MHz) on the blood-brain barrier permeability with two methods of 99mTc-MIBI radiotracer and Evans blue injection.
3. Methods
3.1. Subjects and Radiopharmaceutical Preparation
In this study, 150 male Wistar Albino rats (200 - 250 g, 10 -12-weeks-old) were selected and kept inside an animal room at 22 ± 2ºC with a 12-h light: 12-h dark cycle. Food and water were available ad libitum until the administration of 99mTc-MIBI. The animals were divided into four non-exposed (control) groups and 21 EMF-exposed groups (Table 1) and were housed six per cage randomly. All rats had an intraperitoneal injection (2 mL of Evans blue 1%, Merck) 24 hours before radiotracer administration. A freeze-dried kit (MIBI, Pars Isotope, Iran) was used to prepare 99mTc-MIBI by adding 2 ml of sterile Pertechnetate-Tc solution (150 mCi = 5.55 GBq). The tracer was heated at 100ºC for 10 min. Based on the rat’s weight, about 500 μCi (18.5 MBq) of 99mTc-MIBI was injected through the caudal vein (16). As the best time of quantitative analysis of the left ventricular uptake after tail vein injection of MIBI is 10 to 60 min post-injection (17), the animal brain activity quantitative analysis was done 15, 30, 45, and 60 min after the radiotracer injection. To confirm radiotracer activity, a dose calibrator (RAMS 88, Iran, sensitivity 0.01 μCi) was used in all parts of the experiments.
The Time of EMF Radiation and Rat Brain Activity Evaluation in four Control (Non-Exposed) Groups and 21 EMF-Exposed Groups
Group/Brain Activity (Post Tc-MIBI Injection) | EMF Frequency (MHz) | ||
---|---|---|---|
900 | 1700 | 1900 | |
Non-exposed | |||
15 min | |||
30 min | |||
45 min | |||
60 min | |||
EMF (15 min) | |||
15 min | * | * | * |
30 min | * | * | * |
45 min | * | * | * |
60 min | * | * | * |
EMF (30 min) | |||
30 min | * | * | * |
45 min | * | * | * |
60 min | * | * | * |
3.2. Electromagnetic Field Radiation
After injections, rats were transferred to a Plexiglas cage for whole-body exposure. The cage was placed directly above a mobile phone (Nokia X, China, Human SAR EU: 1.14 W/kg-head, 0.90 W/kg-body), and rats were exposed to either 15 or 30 min of mobile phone radiation (Figure 1). To ensure a stable call mode, a piece of definite music transmitted from another terminal was played by the Nokia X mobile phone so that the mobile phone device was continuously active for the period of emitting. The strength of the electric field (E) in the middle of the exposure cage was assessed by an Electrosmog meter (TES-92, Taiwan) in the mode of X, Y, and Z-axis. The background strength of the electric field was 18.7 ± 1.95 mV/m that increased to 477.1 ± 139.7 mV/m through the call mode. Consistent with available networks, three up-link mobile phone frequencies of 900, 1700, and 1900 MHz were used with changing SIM card input handsets. For the non-exposed groups, the mobile phone was left in the position but switched off. The animals were allowed to move freely in the cage during the exposure. Rats were anesthetized with ether 15, 30, 45, and 60 min after radiotracer injection, and then decapitated. The skull bones were removed carefully, and the complete brain was ablated from the rat skull. Each brain was weighed and kept in a plastic bag to prevent the radiotracer contamination of the dose calibrator. The Research Ethics Committee of Semnan University of Medical Sciences approved that all procedures (IR.SEMUMS.RES: 93/529095) were under the National Institutes of Health (NIH) guidelines for the care and use of laboratory animals (NIH Publications No. 8023, Revised Edition, 1978).
The position of mobile phone under the Plexiglas cage for whole-body exposure of rats.
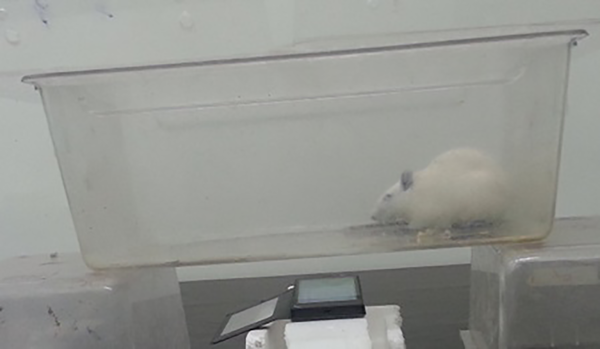
3.3. Data Acquisition
Brain activity with the plastic cover and the whole body activity (after blood extraction) were evaluated by a dose calibrator. The brain-specific activity and brain-to-whole body-specific activity were defined by Equations (1) (1) and (2).
(1) Brain-specific activity = Brain activity (µCi)/Brain weight (g)
(2) Brain/Whole body-specific activity = Brain-specific activity/Whole body-specific activity
One brain in each group was fully inspected, and any Evans blue extravasation was evaluated microscopically. The weight of each brain was used for the quantitative measurement of EB extravasation. Then, samples were homogenized in 2.5 mL of phosphate-buffered saline and mixed with a vortex for 2 min after the addition of 2.5 mL of 60% Trichloroacetic acid to precipitate proteins. Samples were later cooled for 30 min and centrifuged for 30 min at 3,500 rpm. The supernatant was measured at 610 nm for absorbance of EB using a spectrophotometer (18). The Evans blue dye was assessed by a standard curve and expressed as mg % brain tissue (19).
3.4. Statistical Analysis
The Tukey test was used to evaluate the normal distribution of data. The statistical differences between the groups were examined using one-way ANOVA (organ radiation activity) and Kruskal-Wallis (Evans blue OD level) tests with SPSS 16.0 software. Statistical differences were considered significant at the P < 0.05 level. The data are expressed as means ± SD.
4. Results
The microscopic inspection of samples showed no sign of Evans blue extravasation in brain tissues (Figure 2). As shown in Figure 3A, the brain uptake decreased in the non-exposed group with the values of 0.156 ± 0.01 µCi/g and 0.128 ± 0.03 µCi/g at 15 and 60 min, respectively; but there was no significant difference between the times of sampling (P > 0.05). Meanwhile, similar to the radiotracer accumulation in the non-exposed group, after 15 min of EMF radiation at 900, 1700, and 1900 MHz, there were no significant differences between the groups in 60-min post-injection activity (P > 0.05). As shown in Figure 3B, the brain-specific activity with 30-min EMF irradiation showed a significant increase in the 900 MHz group (0.523 ± 0.05 µCi/g) compared to other groups (P = 0.02). In both groups of 1700 and 1900 MHz, brain-specific activity had no change during 60 min post-injection compared to the non-exposed group (P > 0.05).
Microscopic inspection of samples showing no sign of Evans blue extravasation in brain tissues; brain parenchyma (A), hippocampus (B) (Magnification ×40).
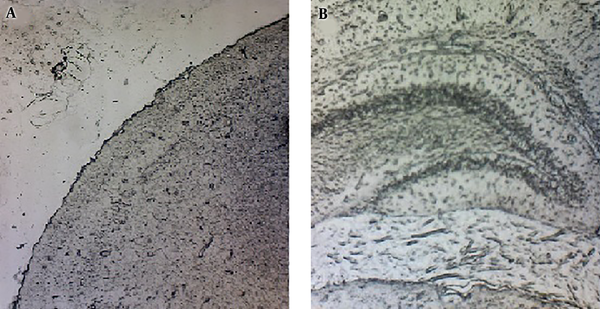
Brain-specific activity during 60 min post-injection of 99mTc-MIBI after EMF irradiation (900, 1700, and 1900 MHz). After 15 min of EMF radiation (A), there were no significant differences between non-exposed and EMF groups (P > 0.05). After 30 min of EMF radiation (B), there was a significant permanent increase in brain-specific activity of the 900 MHz group. The data represent the mean ± SD. (*P < 0.05).
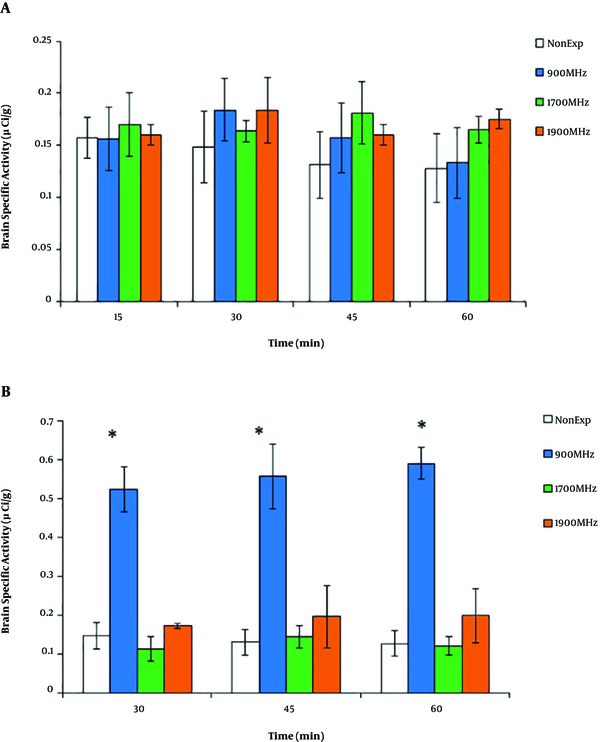
A comparison between the brain/whole body-specific activity in all experimental groups after 15 min of EMF radiation showed no significant differences between 15, 30, 45, and 60-min 99mTc-MIBI activity (P > 0.05) (Figure 4A). On the other hand, as shown in Figure 4B, the brain/whole body-specific activity with 30-min EMF irradiation showed a significant increase in the 900 MHz group (0.3 ± 0.03) compared to others (P = 0.01). In both groups of 1700 and 1900 MHz, brain/whole body-specific activity had no change during 60 min post-injection compared to the non-exposed group (P > 0.05).
Brain/whole body-specific activity during 60 min post-injection of 99mTc-MIBI after EMF irradiation (900, 1700, and 1900 MHz). After 15 min of EMF radiation (A), there were no significant differences between non-exposed and EMF groups (P > 0.05). After 30 min of EMF radiation (B), there was a significant permanent increase in the ratio of the 900 MHz group. The data represent the mean ± SD. (*P < 0.05).
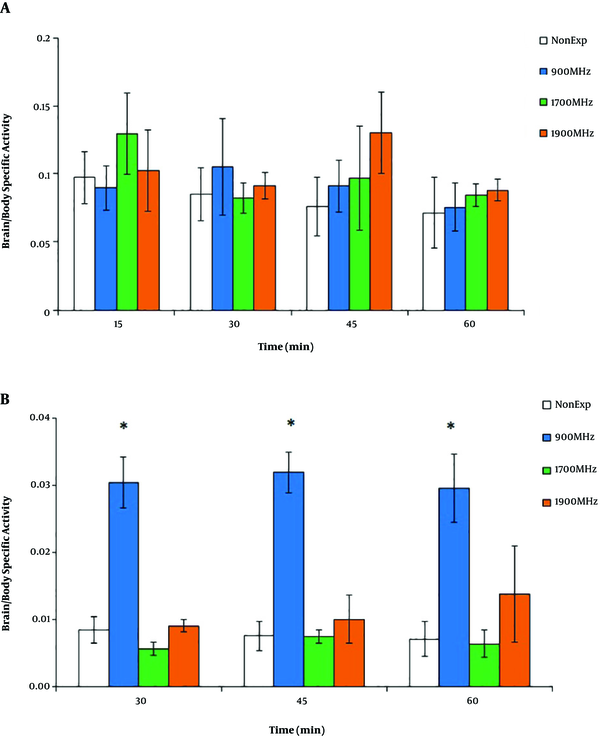
As shown in Figure 5A, similar to Optical Density (OD) of brain tissue extract supernatants in the non-exposed group, there were no significant differences between 15, 30, 45, and 60-min Evans blue accumulation in the brain in the 900, 1700, and 1900 MHz trial groups after 15-min EMF radiation (P > 0.05). A comparison between the OD of brain tissue extract supernatants in all experiment groups after 30-min EMF radiation showed no significant differences in Evans blue accumulation in brains (P > 0.05), with a non-significant increase in the 900 MHz group at the 30-min post-irradiation time (Figure 5B).
The brain tissue extract supernatants optical density after 15 min (A) and 30 min (B) of EMF irradiation (900, 1700, and 1900 MHz). There were no significant differences between non-exposed and EMF groups (P > 0.05). The data represent the mean ± SD.
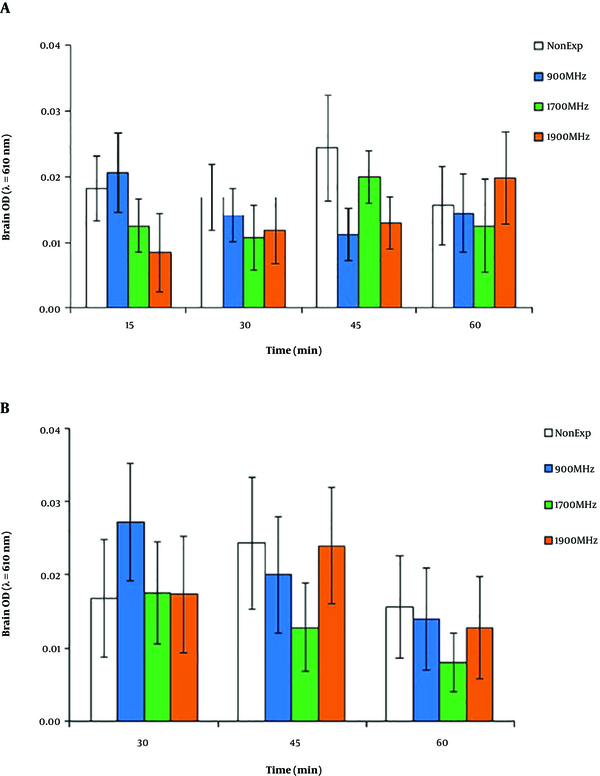
5. Discussion
This study aimed to inspect the distribution of 99mTc-MIBI in the brain to examine the effect of 900, 1700, and 1900 MHz EMF radiation on the brain 99mTc-MIBI build-up. The results showed that 30-min irradiation at 900 MHz increased the penetration of 99mTc-MIBI into the brain. 99mTc-MIBI can accumulate in tissues such as the heart, liver, kidney, and skeletal muscle tissue (13), but it is not absorbed in the normal brain due to the presence of BBB (15). The presence of BBB is crucial for homeostasis of the brain.
Nearly all endogenous materials are excluded from the brain by endothelial cell’s tight junction (4). The experimental evidence of non-thermal EMF exposure shows increases in the permeability of the erythrocyte membrane, effects on the BBB, and increases in calcium ion efflux (20). The 99mTc-MIBI uptake consists of radiotracer passive distribution across plasma and mitochondrial membranes (21, 22). On the other hand, the uptake of 99mTc-MIBI also changes with the intracellular concentration of Na+, indicating that 99mTc-MIBI behaves like Na+ during its uptake (23). Moreover, increases in K+ begin to fall in the 99mTc-MIBI uptake. The cellular radioactivity of 99mTc-MIBI is not dependent on the medium albumin concentration, possibly reproducing a lack in binding of 99mTc-MIBI to albumin (24).
A comparison between the optical density of brain tissue extract supernatants in experimental groups after 15 and 30 min of EMF radiation showed no significant difference between Evans blue accumulations in the samples. These findings show a discrepancy between our results and many of the tests with exposure to the 900 MHz band in which researchers found the increased albumin permeability of BBB (5, 6) and significantly impaired spatial memory (11). The results showed that 20-min exposure to 900 MHz and 1700 MHz radiations surged the permeability of BBB in male rats, but the radiation at 900 MHz could affect females. This result may be due to the physiological differences between female and male animals (12). Eberhardt et al. investigated the effects of 900 MHz EMF on the permeability of the BBB and signs of neuronal damage in rats. Albumin extravasation and its approval into neurons increased after two weeks (7). These results are consistent with the increased penetration of 99mTc-MIBI into the brain after 30 min of 900 MHz radiation in the present study, but this change was not observed with Evans blue injections in any experimental groups.
In other explorations using EMF irradiation, in agreement with our results, no evidence of improved permeability of the BBB was shown. In the De-Gannes study, no effect was found on albumin extravasation or other neurodegenerative markers in the brain after rats were exposed to 900 MHz EMF radiation (25). On the other hand, in young male Wistar rats exposed to the same EMF, no degenerative change and leakage of the BBB were detected (9). Similarly, EMF at 1439 MHz did not induce noticeable variations in the permeability of the BBB and cell morphology in the cerebellum of rats (26-29). Franke et al. also found no change in the permeability of the BBB in an in vitro study with 1966 MHz EMF (30). It is also notable that 2450 MHz exposure did not lead to the extravasation of the Evans blue-albumin compound, confirming the lack of BBB permeability changes in irradiated rats (8). In addition, no adverse impact in terms of BBB leakage or rat brain neuron degeneration was observed after single exposures to 1800 and 1950 MHz EMF (31). The time between the last irradiation and sacrificing animals is the significance of the discovery of drug leakage since the rapid diffusion of extravasated albumin Similarly, the initial albumin leakage into the brain tissue may, along with the delay in BBB opening even 8 weeks after the exposure (6). The results attained in the Mausset-Bonnefont study showed that acute exposure to 900MHz EMF induced changes in the rat brain at both molecular and cellular levels (32).
It has been found that EMF can induce changes in nerve cells, the function of the nerve myelin, and ion channels; additionally, EMF can act as a stress source (33). There is a construction between EMF and neurodegenerative diseases such as headaches, seizures, nausea, and chronic pain (34). The EMF of mobile phones can cause changes in sleep patterns, modifications in the electroencephalogram, and increases in the activities of nitric oxide, malondialdehyde, xanthine oxidase, and adenosine deaminase (3). The human brain is the closest organ to the mobile phone during the call mode. Despite biological, physiological, epidemiological, and toxicological studies to assess the adverse outcomes, the potential effects of mobile phone EMF exposure on the BBB, and the possible involved biochemical pathways are still controversial. Likewise, experimental conditions (time of exposure, frequency, intensity, and type of the field) and tissue parameters are critical factors. As most humans use mobile phones for daily communications, all users around the world need to be advised of the effects of modern call devices.
5.1. Study Limitations
One of the most important limitations of this study was associated with its methodology. The present study used 15 or 30 min single exposures to EMF radiation and found only measurable changes in the 900MHz band. In contrast, chronic long-term irradiation may cause greater BBB permeability at other mobile phone EMF frequencies.
5.2. Conclusion
The irradiation with a mobile phone at 900 MHz EMF could affect 99mTc-MIBI brain extravasation in the rat brain, suggesting that acute short-term irradiation is associated with blood-brain barrier permeability. Due to the increasing number of mobile phone users in the world, advanced investigations and further experiments should be performed to explain the mechanism of this consequence.
Acknowledgements
References
-
1.
Altun G, Kaplan S, Deniz OG, Kocacan SE, Canan S, Davis D, et al. Protective effects of melatonin and omega-3 on the hippocampus and the cerebellum of adult Wistar albino rats exposed to electromagnetic fields. J Microsc Ultrastruct. 2017;5(4):230-41. [PubMed ID: 30023259]. [PubMed Central ID: PMC6025784]. https://doi.org/10.1016/j.jmau.2017.05.006.
-
2.
Deltour I, Wiart J, Taki M, Wake K, Varsier N, Mann S, et al. Analysis of three-dimensional SAR distributions emitted by mobile phones in an epidemiological perspective. Bioelectromagnetics. 2011;32(8):634-43. [PubMed ID: 21695709]. https://doi.org/10.1002/bem.20684.
-
3.
Ilhan A, Gurel A, Armutcu F, Kamisli S, Iraz M, Akyol O, et al. Ginkgo biloba prevents mobile phone-induced oxidative stress in rat brain. Clin Chim Acta. 2004;340(1-2):153-62. [PubMed ID: 14734207]. https://doi.org/10.1016/j.cccn.2003.10.012.
-
4.
Zhou JX, Ding GR, Zhang J, Zhou YC, Zhang YJ, Guo GZ. Detrimental effect of electromagnetic pulse exposure on permeability of in vitro blood-brain-barrier model. Biomed Environ Sci. 2013;26(2):128-37. [PubMed ID: 23336136]. https://doi.org/10.3967/0895-3988.2013.02.007.
-
5.
Persson BR, Salford LG, Brun A. Blood-brain barrier permeability in rats exposed to electromagnetic fields used in wireless communication. Wireless Networks. 1997;3(6):455-61. https://doi.org/10.1023/a:1019150510840.
-
6.
Salford LG, Brun AE, Eberhardt JL, Malmgren L, Persson BR. Nerve cell damage in mammalian brain after exposure to microwaves from GSM mobile phones. Environ Health Perspect. 2003;111(7):881-3. discussion A408. [PubMed ID: 12782486]. [PubMed Central ID: PMC1241519]. https://doi.org/10.1289/ehp.6039.
-
7.
Eberhardt JL, Persson BR, Brun AE, Salford LG, Malmgren LO. Blood-brain barrier permeability and nerve cell damage in rat brain 14 and 28 days after exposure to microwaves from GSM mobile phones. Electromagn Biol Med. 2008;27(3):215-29. [PubMed ID: 18821198]. https://doi.org/10.1080/15368370802344037.
-
8.
Cosquer B, Galani R, Kuster N, Cassel JC. Whole-body exposure to 2.45 GHz electromagnetic fields does not alter anxiety responses in rats: a plus-maze study including test validation. Behav Brain Res. 2005;156(1):65-74. [PubMed ID: 15474651]. https://doi.org/10.1016/j.bbr.2004.05.007.
-
9.
Kumlin T, Iivonen H, Miettinen P, Juvonen A, van Groen T, Puranen L, et al. Mobile phone radiation and the developing brain: behavioral and morphological effects in juvenile rats. Radiat Res. 2007;168(4):471-9. [PubMed ID: 17903040]. https://doi.org/10.1667/RR1002.1.
-
10.
Ding GR, Qiu LB, Wang XW, Li KC, Zhou YC, Zhou Y, et al. EMP-induced alterations of tight junction protein expression and disruption of the blood-brain barrier. Toxicol Lett. 2010;196(3):154-60. [PubMed ID: 20412840]. https://doi.org/10.1016/j.toxlet.2010.04.011.
-
11.
Tang J, Zhang Y, Yang L, Chen Q, Tan L, Zuo S, et al. Exposure to 900 MHz electromagnetic fields activates the mkp-1/ERK pathway and causes blood-brain barrier damage and cognitive impairment in rats. Brain Res. 2015;1601:92-101. [PubMed ID: 25598203]. https://doi.org/10.1016/j.brainres.2015.01.019.
-
12.
Sirav B, Seyhan N. Effects of GSM modulated radio-frequency electromagnetic radiation on permeability of blood-brain barrier in male & female rats. J Chem Neuroanat. 2016;75(Pt B):123-7. [PubMed ID: 26723545]. https://doi.org/10.1016/j.jchemneu.2015.12.010.
-
13.
Chang C, Huang W, Yang S, Yeh H, Kao C, Chen G. Effect of P-glycoprotein and multidrug resistance associated protein gene expression on Tc-99m MIBI imaging in hepatocellular carcinoma. Nuclear Medicine and Biology. 2003;30(2):111-7. https://doi.org/10.1016/s0969-8051(02)00414-6.
-
14.
Staudenherz A, Fazeny B, Marosi C, Nasel C, Hoffmann M, Puig S, et al. Does (99m)Tc-Sestamibi in high-grade malignant brain tumors reflect blood-brain barrier damage only? Neuroimage. 2000;12(1):109-11. [PubMed ID: 10875907]. https://doi.org/10.1006/nimg.2000.0594.
-
15.
Packard AB, Kronauge JF, Limpa-Amara N, Lampson L, O'Tuama LA, Jones AG. Tumor uptake of 99mTc-MIBI and 201T1 by a 9L gliosarcoma brain tumor model in rats. Nuclear Medicine and Biology. 1993;20(6):773-6. https://doi.org/10.1016/0969-8051(93)90164-p.
-
16.
Jadidi M, Bokharaeian M, Esmaili MH, Hasanzadeh H, Ghorbani R. Cell Phone EMF Affects Rat Pulmonary Tc-MIBI Uptake and Oxidative Stress. Iranian Journal of Science and Technology, Transactions A: Science. 2019;43(4):1491-7. https://doi.org/10.1007/s40995-019-00679-4.
-
17.
Vrachimis A, Hermann S, Mathe D, Schober O, Schafers M. Systematic evaluation of 99mTc-tetrofosmin versus 99mTc-sestamibi to study murine myocardial perfusion in small animal SPECT/CT. EJNMMI Res. 2012;2(1):21. [PubMed ID: 22626255]. [PubMed Central ID: PMC3413527]. https://doi.org/10.1186/2191-219X-2-21.
-
18.
Kaya M, Kalayci R, Kucuk M, Arican N, Elmas I, Kudat H, et al. Effect of losartan on the blood-brain barrier permeability in diabetic hypertensive rats. Life Sci. 2003;73(25):3235-44. [PubMed ID: 14561528]. https://doi.org/10.1016/j.lfs.2003.06.014.
-
19.
Oztas B, Kalkan T, Tuncel H. Influence of 50 Hz frequency sinusoidal magnetic field on the blood-brain barrier permeability of diabetic rats. Bioelectromagnetics. 2004;25(5):400-2. [PubMed ID: 15197766]. https://doi.org/10.1002/bem.20030.
-
20.
Đinđić B, Sokolović D, Krstić D, Petković D, Jovanović J, Muratović M. Biochemical and histopathological effects of mobile phone exposure on rat hepatocytes and brain. Acta Medica Medianae. 2010;49(1):37-42.
-
21.
Chiu ML, Kronauge JF, Piwnica-Worms D. Effect of mitochondrial and plasma membrane potentials on accumulation of hexakis (2-methoxyisobutylisonitrile) technetium(I) in cultured mouse fibroblasts. J Nucl Med. 1990;31(10):1646-53. [PubMed ID: 2213187].
-
22.
Metaye T, Rosenberg T, Guilhot J, Bouin-Pineau MH, Perdrisot R. The presence of sodium nitrate in generator eluate decreases the radiochemical purity of 99mTc-sestamibi. J Nucl Med Technol. 2012;40(3):187-93. [PubMed ID: 22872664]. https://doi.org/10.2967/jnmt.111.101246.
-
23.
Arbab AS, Koizumi K, Toyama K, Arai T, Araki T. Technetium-99m-tetrofosmin, technetium-99m-MIBI and thallium-201 uptake in rat myocardial cells. J Nucl Med. 1998;39(2):266-71. [PubMed ID: 9476934].
-
24.
Bernard BF, Krenning EP, Breeman WA, Ensing G, Benjamins H, Bakker WH, et al. 99mTc-MIBI, 99mTc-Tetrofosmin and 99mTc-Q12 In Vitro and In Vivo. Nucl Med Biol. 1998;25(3):233-40. https://doi.org/10.1016/s0969-8051(97)00201-1.
-
25.
de Gannes FP, Billaudel B, Taxile M, Haro E, Ruffie G, Leveque P, et al. Effects of head-only exposure of rats to GSM-900 on blood-brain barrier permeability and neuronal degeneration. Radiat Res. 2009;172(3):359-67. [PubMed ID: 19708785]. https://doi.org/10.1667/RR1578.1.
-
26.
Tsurita G, Nagawa H, Ueno S, Watanabe S, Taki M. Biological and morphological effects on the brain after exposure of rats to a 1439 MHz TDMA field. Bioelectromagnetics. 2000;21(5):364-71. https://doi.org/10.1002/1521-186x(200007)21:5<364::aid-bem5>3.0.co;2-q.
-
27.
Kuribayashi M, Wang J, Fujiwara O, Doi Y, Nabae K, Tamano S, et al. Lack of effects of 1439 MHz electromagnetic near field exposure on the blood-brain barrier in immature and young rats. Bioelectromagnetics. 2005;26(7):578-88. [PubMed ID: 16142770]. https://doi.org/10.1002/bem.20138.
-
28.
Masuda H, Ushiyama A, Hirota S, Wake K, Watanabe S, Yamanaka Y, et al. Effects of acute exposure to a 1439 MHz electromagnetic field on the microcirculatory parameters in rat brain. In Vivo. 2007;21(4):555-62. [PubMed ID: 17708346].
-
29.
Masuda H, Ushiyama A, Hirota S, Wake K, Watanabe S, Yamanaka Y, et al. Effects of subchronic exposure to a 1439 MHz electromagnetic field on the microcirculatory parameters in rat brain. In Vivo. 2007;21(4):563-70. [PubMed ID: 17708347].
-
30.
Franke H, Ringelstein EB, Stogbauer F. Electromagnetic fields (GSM 1800) do not alter blood-brain barrier permeability to sucrose in models in vitro with high barrier tightness. Bioelectromagnetics. 2005;26(7):529-35. [PubMed ID: 16142784]. https://doi.org/10.1002/bem.20123.
-
31.
Poulletier de Gannes F, Masuda H, Billaudel B, Poque-Haro E, Hurtier A, Leveque P, et al. Effects of GSM and UMTS mobile telephony signals on neuron degeneration and blood-brain barrier permeation in the rat brain. Sci Rep. 2017;7(1):15496. [PubMed ID: 29138435]. [PubMed Central ID: PMC5686211]. https://doi.org/10.1038/s41598-017-15690-1.
-
32.
Mausset-Bonnefont AL, Hirbec H, Bonnefont X, Privat A, Vignon J, de Seze R. Acute exposure to GSM 900-MHz electromagnetic fields induces glial reactivity and biochemical modifications in the rat brain. Neurobiol Dis. 2004;17(3):445-54. [PubMed ID: 15571980]. https://doi.org/10.1016/j.nbd.2004.07.004.
-
33.
Kim JH, Lee JK, Kim HG, Kim KB, Kim HR. Possible Effects of Radiofrequency Electromagnetic Field Exposure on Central Nerve System. Biomol Ther (Seoul). 2019;27(3):265-75. [PubMed ID: 30481957]. [PubMed Central ID: PMC6513191]. https://doi.org/10.4062/biomolther.2018.152.
-
34.
Terzi M, Ozberk B, Deniz OG, Kaplan S. The role of electromagnetic fields in neurological disorders. J Chem Neuroanat. 2016;75(Pt B):77-84. [PubMed ID: 27083321]. https://doi.org/10.1016/j.jchemneu.2016.04.003.