Abstract
Background:
Regular exercise can alleviate oxidative stress. There are no studies examining changes in this variable as a result of an intense acute taekwondo (TKD) exercise.Objectives:
We aimed to investigate the effect of one bout of intense TKD exercise on superoxide dismutase (SOD), glutathione peroxidase (GPx), catalase (CAT), total antioxidant capacity (TAC), and malondialdehyde (MDA) in adolescent female taekwondokas.Methods:
Ten adolescent female taekwondokas (age: 13.71 ± 2.05 years; body mass index: 19.75 ± 2.83 kg/m2) voluntarily participated in the present study. They performed one bout of intense TKD protocol. Blood samples were obtained before and immediately after exercise. Then, SOD, GPx, CAT, TAC, and MDA were measured using the spectrophotometric method. Plasma volume was corrected using the Dill and Costill equation. Data were analyzed using a paired-sample t test. The significance level was set at P < 0.05.Results:
The results revealed that CAT activity (P = 0.043) and serum TAC level (P = 0.039) significantly increased after TKD exercise. However, no significant change was found in SOD (P = 0.414), GPx activity (P = 0.196), and MDA levels (P = 0.377) immediately after exercise.Conclusions:
Collectively, lipid peroxidation did not occur after one bout of intense TKD exercise in adolescent girls, which may be due to increased TAC levels and the activity of the CAT enzyme.Keywords
Taekwondo Superoxide Dismutase Glutathione Peroxidase Catalase Total Antioxidant Capacity Malondialdehyde Adolescent
1. Background
Oxygen is essential for the life of all aerobic organisms. However, there is growing evidence that 2% to 5% of the oxygen consumed is converted to reactive oxygen species (ROS) during respiration in the mitochondrial electron transport chain (1). Oxygen intake during exercise is 10 to 15 times the normal condition. Besides, the flow of oxygen to active tissues increases up to 100 times (2) so that the ROS generation rate is expected to be higher during exercise than in normal conditions. Reactive oxygen species contain one or more unpaired electrons that are continuously produced in all living cells and are unable to exist independently. Hence, they react with other molecules to reach stability. In this context, an increase in lipid peroxidation has been revealed after acute exercise in various studies (1, 2). Aldehydes, especially malondialdehyde (MDA), have been frequently used as the by-products of lipid peroxidation in response to exercise (3). Based on such evidence, it is thought that exercise is destructive because it generates ROS. This supposition is not necessarily true because a living body is equipped with a well-organized antioxidant system consisting of enzymes such as superoxide dismutase (SOD), glutathione peroxidase (GPx), catalase (CAT), and numerous non-enzymatic antioxidants, which neutralize ROS (3).
Exercise can produce a disturbance in the prooxidant-antioxidant balance depending on its intensity and duration (1-3). In this context, it has been shown that a moderate weight reduction program resulted in elevated levels of SOD and total antioxidant capacity (TAC) when compared to low and high reduction programs (4). The short-term cycle sprint test (5) and incremental rowing ergometer (6) may result in a dramatic increase in SOD and MDA in sprinter and rower males, while no significant change in serum GPx occurs (5, 6). Other research findings indicate a significant increase in serum GPx, CAT activity, and TAC concentration after a high-intensity cycling test (7). Moreover, eccentric exercise induces a significant increase in the plasma TAC and MDA levels in sedentary women (8). In contrast, one study showed that 20-s maximal cycling sprint anaerobic exercise evoked a significant reduction in SOD, GPx, and CAT activity 15 min after exercise in non-trained participants (9). Other studies have not shown any significant changes in MDA levels and antioxidant enzyme activities in sera and lymphocytes after acute swimming (10), prolong cycling exercise (11), and graded exercise test (12) in trained athletes.
There has been a considerably increasing interest in the scientific research of taekwondo (TKD) in the last few decades (13). As a popular combat sport, TKD has been found as a useful exercise regimen for the physical education of students. In this regard, several study groups have suggested that TKD is effective for enhancing physical fitness and growth development and improving body composition in growing participants (14). The TKD athletes, on the other hand, perform intensified technical training and physical fitness in the pre-competition training period. In this regard, it has been concluded that intense bouts of exercise have critical effects on physiological functions in elite players who perform intense exercise events (15). Therefore, this issue has raised concerns among parents and educators.
2. Objectives
To the best of our knowledge, no study has examined the effect of TKD on oxidant-antioxidant balance in adolescent female taekwondokas. For better clarification of this issue, the purpose of the present study was to investigate the effect of one bout of intense TKD exercise session on oxidative stress levels in adolescent female taekwondokas.
3. Methods
3.1. Participants
Ten healthy adolescent female taekwondokas (aged 13.71 ± 2.05 years) from Mashhad city (Razavi Khorasan province, Iran) volunteered to participate in the study, as notified via advertisement. Identification card information was the basis for determining the age of the participants. They had at least four years of athletic activity in TKD. They performed TKD training regularly for three sessions per week in the past four years. Subjects were examined by a general physician two weeks before the protocol started, and none of them had any acute or chronic illness. The subjects had a history of participating in provincial competitions. Moreover, no subjects were receiving any drugs, antioxidants, or anabolic-steroid supplements at the time of the study. All subjects had normal biphasic menstrual cycles (9). The study took place in the off-season of competitions. Subjects refrained from participating in any exercise programs two weeks before the onset of the protocol. The research protocol was approved by the Institutional Review Board (University of Bojnord, Iran) and the Institutional Ethics Committee for Human Use (IR.IAU.BOJNOURD.REC.1399.005). Besides, all the procedures followed the 1975 Declaration of Helsinki, as revised in 1996. Moreover, they were encouraged to participate voluntarily and allowed to leave the study without any wrongs, resentment, or discontent. Finally, subjects’ guardians signed a consent form after being informed orally about the program, its benefits, and potential risks.
3.2. Determination of Body Composition
One week before the test day, the body composition of the subjects was determined. Body Fat Mass (BFM), Body Mass Index (BMI), Basal Metabolic Rate (BMR), Fat-free Mass (FFM), Percentage of Body Fat (PBF), Skeletal Muscle Mass (SMM), and Waist-to-Hip Ratio (WHR) were measured using a Bioimpendence body composition analyzer (X-Contact 356 model, Jawon Medical, South Korea) at 9:00 a.m. For this purpose, participants were asked to empty their bladder before the test. They were instructed to stand on metal footpads with bare feet and grasp a pair of electrodes fixed on a handle. The anthropometric characteristics are depicted in Table 1.
Anthropometric Characteristics (n = 10)a
Variables | Mean ± SD |
---|---|
Weight (kg) | 46.60 ± 10.08 |
Height (cm) | 153.41 ± 13.67 |
BMI (kg/m2) | 19.75 ± 2.83 |
WHR | 0.76 ± 0.03 |
BFP (%) | 21.63 ± 5.88 |
BFM (kg) | 11.45 ± 4.97 |
SMM (kg) | 18.81 ± 4.57 |
FFM (kg) | 33.43 ± 6.87 |
BMR | 360.68 ± 78.03 |
3.3. Determination of Aerobic and Anaerobic Power
The aerobic and anaerobic power of the taekwondokas was evaluated one-week before performing the TKD exercise. Aerobic power (as measured by peak oxygen uptake) was determined using the 12-min Run Cooper Test (16). The anaerobic power of taekwondokas was determined using the Running-based Anaerobic Sprint Test (RAST) (17). In brief, the subject stood at one end of the 35-m track and started a maximal sprint on the command 'go'. Subjects sprinted maximally through the line each time. After 10 seconds, the next sprint started from the opposite end of the 35-m track. This procedure was repeated until six sprints were completed. Power for each sprint was calculated according to the following equation: Power = bodyweight of the subject × Distance2 ÷ Time3 (17). The highest and lowest power outputs were considered as maximum and minimum power values, respectively. Besides, the average power of subjects was calculated by dividing the sum of all the six obtained powers by 6. Finally, the fatigue index value of the subjects was determined by dividing the difference between maximal and minimal anaerobic power by the total time for the six sprints (17). Moreover, the upper-body explosive power was assayed by a seated medicine ball chest throw. The lower-body explosive power was assayed by the Sargent jump test. The Sargent jump height was subsequently used to determine the peak power according to the Sayers Equation. Each Each subject had four attempts interspersed with approximately a 1.5-min rest between each trial, and the best record from each subject was used in data analysis. Table 2 summarizes the aerobic and anaerobic power information of the subjects.
Aerobic and Anaerobic Power Characteristics of the Subjects (n = 10)a
Variables | Mean ± SD |
---|---|
Peak VO2 (ml/kg/min) | 27.25 ± 4.05 |
Minimum power (W) | 90 ± 45.50 |
Maximum power (W) | 1950 ± 72.05 |
Average power (W) | 1352 ± 58.80 |
Fatigue index | 2.34 ± 1.15 |
Upper-body explosive power (cm) | 185.57 ± 45.12 |
Lower-body explosive power (W) | 5383 ± 678.25 |
3.4. Intense TKD Protocol
The current study took place under the supervision of a qualified and experienced TKD coach at the Mashhad Taekwondo Home (Razavi Khorasan province, Iran) one month after the end of the provincial TKD season (14). The study began at 11:00 a.m. and lasted for about 1.5 h. The protocol started by jogging and plyometric and stretching exercises for 20 min as a warm-up. Then, they performed high-intensity, short-duration tactical TKD drills for 30 min. Subsequently, the participants were divided into two groups of 5 with one subject in each group designated as the iron man. The iron man section lasted for 20 min. Then, pairs with similar weights and abilities participated in 10-min matches. During this phase, each athlete competed for three 120-second rounds with a one-minute break between the rounds. Finally, the protocol ended with slow jogging and stretch exercise for 10 min as a cold-down. The participants were encouraged to have a short rest when exhausted (14).
3.5. Sample Collection and Biochemical Assays
For the biochemical assays, 7 ml blood was collected from the participants’ antecubital vein by standard phlebotomy before and immediately after the acute exercise. The collected samples were stored under cold conditions and sent to the Arad medical laboratory (Mashhad, Iran). The samples were centrifuged (Universal Model, Behdad Corporation, Iran) at 3,000 rpm for 10 min at 4°C to separate sera. Serum SOD (#SD125, Randox Laboratories Ltd., Crumlin, United Kingdom), GPX (#RS504, Randox Laboratories Ltd., Crumlin, United Kingdom), and CAT (#ZB-CAT-96A, ZellBio GmbH Corporation, Germany) activities were calorimetrically assayed according to the manufacturer's instructions. The sensitivity of the CAT kit was 0.5 U/L. The intra- and inter-assay coefficients of variation for the CAT kit were claimed to be 6.3% and 7.9%, respectively. Moreover, serum TAC and MDA concentrations were determined by a colorimetric TAC assay kit (#ZB-TAC-96A, ZellBio GmbH Corporation, Germany) and MAD colorimetric assay kit (#ZB-MDA-96A, ZellBio GmbH Corporation, Germany), respectively. The sensitivities of the TAC and MDA kits were 0.1 mM and 0.1 µM, respectively. The intra- and inter-assay variation coefficients for TAC were claimed to be less than 3.4% and 4.2%, respectively. The intra- and inter-assay coefficients of variation for MDA were claimed to be 5.8% and 7.6%, respectively.
3.6. Diet Control
One week before the study, the subjects were asked to record their diets for three consecutive days. The nutritional analysis showed that all participants had a normal diet regimen. They were prohibited from any intense training and asked to consume a carbohydrate-rich diet (glycogen loading) for three days before the acute intense TKD session. In addition, they were instructed not to consume any antioxidants and anabolic-androgenic supplements before the study. Moreover, a similar light breakfast was provided on the morning of the test. They were permitted to have free access to water and drinks when thirsty (18).
3.7. Statistical Analyses
The obtained data were analyzed using the Statistical Package for the Social Science version 16.0 (SPSS, Inc., Chicago, IL). Initially, the normal data distribution was checked and confirmed by the Shapiro-Wilk normality test. Subsequently, a paired sample t-test was used to compare the mean differences between the pretest and posttest. Besides, the significance level was set at P < 0.05.
4. Results
Concerning antioxidant enzyme activity, the CAT activity significantly increased from baseline (1.88 ± 0.56 kU/L) to the post-intervention phase (3.25 ± 1.14 kU/L) (P = 0.043) (Figure 1A). However, SOD (0.30 ± 0.13 and 0.26 ± 0.06 U/L for pre- and post-intervention phases, respectively) (P = 0.414) and GPX activity (42.47 ± 9.12 and 45.84 ± 11.76 U/L for pre-and post-intervention phases, respectively) (P = 0.196) did not change significantly after the intervention (Figure 1B and C).
Effect of acute taekwondo exercise on serum catalase (A), superoxide dismutase (B), and glutathione peroxidase (C) in adolescent female taekwondokas. *Significant difference compared to pre-exercise.
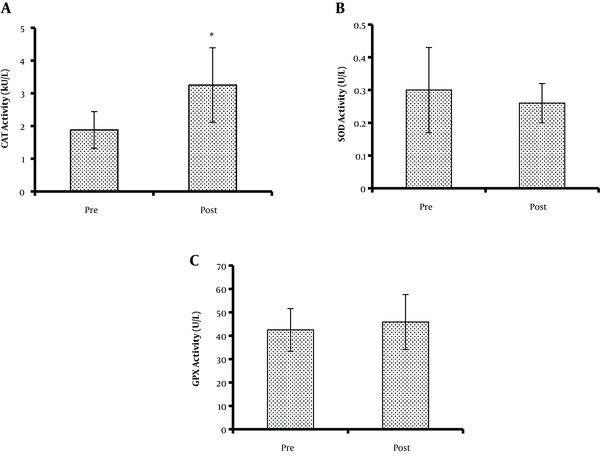
Concerning the total oxidant status, the serum TAC level (4,738 ± 176 and 6,114 ± 276 µM/L before and after exercise, respectively) significantly increased after the protocol (P = 0.039) (Figure 2). Besides, MDA, as a lipid peroxidation marker, did not change significantly after one bout of acute TKD exercise (1,564 ± 398 and 1,670 ± 371 nM for pre- and post-intervention phases, respectively) (P = 0.377) (Figure 3).
Effect of acute taekwondo exercise on serum total antioxidant capacity in adolescent female taekwondokas. *Significant difference compared to pre-exercise.
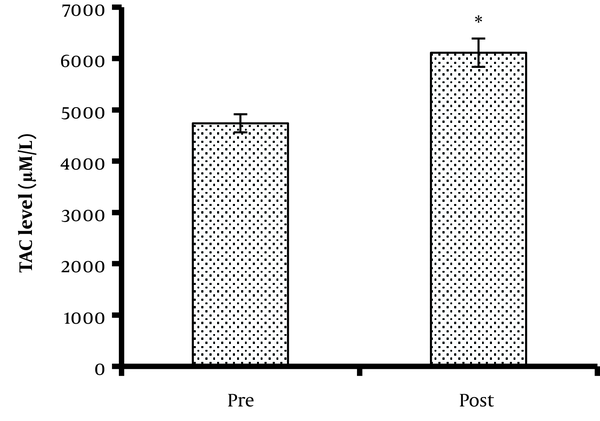
Effect of acute taekwondo exercise on serum malondialdehyde in adolescent female taekwondokas.
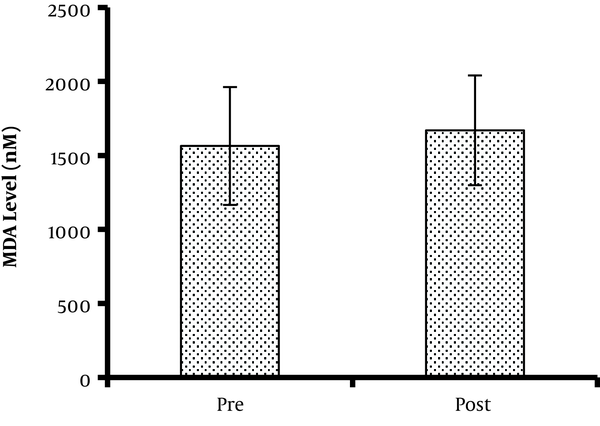
5. Discussion
To assay exercise-induced oxidative stress, most researchers have evaluated various stress markers in the blood (1). To the best of our knowledge, this is the first study of its kind to examine changes in oxidative stress parameters following one bout of intense TKD exercise in adolescent female taekwondokas. The main findings were that after the acute exercise, CAT activity and TAC levels increased, whereas the MDA levels and SOD and GPx activity remained unchanged.
Antioxidant defense systems resist redox disturbances in the cell and protect cells from oxidative injury during intense or prolonged exercise (3). Superoxide, as a prominent ROS, is generated at significant amounts intracellularly, both in the cytosol via flavin-containing enzymes and in mitochondria, prominently due to the escape of electrons from the respiratory chain (2). It has been documented that the superoxide anion is dismutated rapidly via SOD to oxygen and hydrogen peroxide in cellular compartments. Hydrogen peroxide can be enzymatically metabolized to oxygen and water by GPx and CAT (1-3). Our finding is consistent with a study that showed a significant increase in serum CAT activity immediately to 48 h after a sprint test (7). One study has reported no significant changes in SOD, GPx, and CAT enzyme activity in erythrocytes and lymphocytes in response to 30-min swimming exercise in female swimmers (10). In contrast, Cases et al. found significant increases in lymphocyte total SOD activity and levels of CuZn-SOD and Mn-SOD isoenzymes in response to prolonged exercise (11). Two studies have reported that antioxidant enzyme activity differs overtime after the end of the exercise (7, 9). Wiecek et al. showed no significant changes in SOD, GPx, and CAT activity immediately after anaerobic exercise; however, antioxidant enzyme activity was induced 15 min after exercise (9). Besides, Bogdanis et al. have reported a significant increase in serum GPx 24 to 48 h after short-term, high-intensity cycling, while no dramatic changes were observed immediately after the exercise (7). Therefore, part of the discrepancy in results may be because of the differences in the assay time. Recently, it has been reported that there are no gender-related differences to attribute to changes in SOD, GPx, and CAT activity after exercise. It has been concluded that estradiol concentrations correlate negatively with post-exercise changes in SOD activity in women with normal biphasic menstrual cycles (19). Joo et al. (2004) report that free radicals generated during exercise are more easily scavenged when the estradiol concentration is higher. Moreover, no correlations have been observed between the activity of antioxidant enzymes and progesterone levels (19). Therefore, the current study was conducted with females who had normal biphasic menstrual cycles during the follicular phase to eliminate the potential effects of estradiol levels (9).
Total antioxidant capacity is an analyte frequently used to assess the antioxidant status of biological samples and can assay the antioxidant response to the free radicals produced during exercise (20-23). In this regard, it has been noticed that a substantial increase occurs at the level of serum TAC during one bout of intense TKD exercise. These findings have been confirmed in other studies. Consistent with the current study, Babaei et al. reported elevations in the serum TAC level in untrained males who conducted 30 min of aerobic exercise on a treadmill at 75% maximal oxygen consumption, while serum TAC returned to baseline values 2 and 24 h after exercise (20). In addition, it has been exhibited that four to six 30-s bouts of high-intensity cycling can induce a significant increase in the concentration of TAC immediately to 48 h after the test (7). Skenderi et al. also reported an increase in the serum TAC level in elite sportsmen subject to the ultramarathon race Spartathlon (21). A similar increase in plasma TAC in response to eccentric exercise (Ellestad test) has been reported in sedentary female students (8). In contrast, no significant changes in plasma TAC have been found after one bout of incremental rowing ergometer in Polish rowing athletes (6).
Total antioxidant capacity reflects the cumulative effect of all antioxidants present in body fluids and is used to assess a range of diseases in humans and animals (20-23). In other words, TAC is influenced by all antioxidants in the blood, such as ascorbate, alpha-tocopherol, beta-carotene, glutathione, bilirubin, and uric acid (20-23). Studies have revealed a significant positive correlation between uric acid and TAC (5, 21-25). In reality, an increase in uric acid increases blood TAC by one-third (20). Uric acid supplementation also increases serum urate and TAC concentrations in men and women (22). In this regard, a concurrent increase in serum TAC, uric acid, and bilirubin has been reported immediately after a repeated cycle sprint test (5), long-distance endurance exercise (21), and long-distance aerobic exercise (23). Besides, alpha-tocopherol (24) and ascorbic acid (25) are responsible for up to 10% and 24% increases in plasma TAC, respectively. A 90-min soccer game has been shown to induce an acute increase in uric acid, alpha-tocopherol, and ascorbic acid in well-trained female athletes (26). Peake’s review has pointed out a transient increase in circulating ascorbic acid in the hours following prolonged exercise (25). It has also been suggested that oxidative stress following prolonged endurance exercise is a stimulus for the release of ascorbic acid from the adrenal gland (25). Another contributor to the increased plasma TAC level after exercise is an increase in the reduced glutathione levels of plasma, as proven by one study (8). Collectively, the observed increase in the TAC level after acute exercise may be rooted in the cumulative effect of serum non-enzymatic antioxidants.
In the absence of an antioxidant system, hydrogen peroxide can be converted to the hydroxyl radical, which is extremely reactive and results in lipid peroxidation. Malondialdehyde reflects lipid peroxidation induced by oxidative stress (1-3). The findings concerning the MDA response to exercise are controversial in the literature. In line with our findings, a study addressing oxidative stress in soccer players showed no significant changes in MDA levels (27). Babaei et al. found no significant changes in serum MDA levels after running on a treadmill in sedentary male subjects (20). Additionally, one bout of graded exercise test increased the serum MDA level in untrained individuals. However, no significant changes occurred in serum MDA levels in aerobically and anaerobically trained athletes (12). Therefore, the level of physical fitness of individuals can affect the response of oxidative stress to exercise (12). Moreover, no significant changes were found in the serum and lymphocyte MDA levels in response to a five-day cycling competition in professional cyclists (11). Nonetheless, the MDA levels increased two hours after the end of exercise in their study. In contrast, the MDA/cholesterol ratio considerably increased immediately at the end of the ultramarathon Spartathlon race in one study (21). Four consecutive cycle sprint tests on a cycle ergometer also induced a significant increase in blood MDA in male sprinters (5). Moreover, both incremental rowing ergometer at 40 to 90% of maximal aerobic power (6) and four to six 30-s bout of high-intensity cycling (7) induced a significant increase in the concentration of lipid peroxidation product levels immediately to 48 h after exercise. Furthermore, a study by Norouziyan et al. also demonstrated an increase in plasma MDA induced by eccentric exercise (8). Malondialdehyde increases depending on the duration and intensity of exercise. Gonzalez et al. concluded that aerobic exercise-induced increment in both uric acid and TAC seems to inhibit lipid hydroperoxide generation (23). Therefore, the non-significant changes in serum MDA in the current study may be associated with an increase in serum TAC.
Acknowledgements
References
-
1.
Urso ML, Clarkson PM. Oxidative stress, exercise, and antioxidant supplementation. Toxicology. 2003;189(1-2):41-54. [PubMed ID: 12821281]. https://doi.org/10.1016/s0300-483x(03)00151-3.
-
2.
Masuda K, Tanabe K, Kuno S. Exercise and reactive oxygen species in elderly-exercise as prevention of oxidative stress. Int J Sport Stud Hlth. 2006;4:348-59. https://doi.org/10.5432/ijshs.4.348.
-
3.
Powers SK, Jackson MJ. Exercise-induced oxidative stress: cellular mechanisms and impact on muscle force production. Physiol Rev. 2008;88(4):1243-76. [PubMed ID: 18923182]. [PubMed Central ID: PMC2909187]. https://doi.org/10.1152/physrev.00031.2007.
-
4.
Knapik K, Sieroń K, Wojtyna E, Onik G, Romuk E, Birkner E, et al. Precompetitional weight reduction modifies prooxidative-antioxidative status in judokas. Oxid Med Cell Longev. 2019;2019:2164698. [PubMed ID: 31428221]. [PubMed Central ID: PMC6679850]. https://doi.org/10.1155/2019/2164698.
-
5.
Jówko E, Długołęcka B, Makaruk B, Cieśliński I. The effect of green tea extract supplementation on exercise-induced oxidative stress parameters in male sprinters. Eur J Nutr. 2015;54(5):783-91. [PubMed ID: 25120110]. [PubMed Central ID: PMC4500852]. https://doi.org/10.1007/s00394-014-0757-1.
-
6.
Skarpańska-Stejnborn A, Basta P, Pilaczyńska-Szcześniak Ł, Horoszkiewicz-Hassan M. Black grape extract supplementation attenuates blood oxidative stress in response to acute exercise. Biol Sport. 2010;27(1):41-6. https://doi.org/10.5604/20831862.907791.
-
7.
Bogdanis GC, Stavrinou P, Fatouros IG, Philippou A, Chatzinikolaou A, Draganidis D, et al. Short-term high-intensity interval exercise training attenuates oxidative stress responses and improves antioxidant status in healthy humans. Food Chem Toxicol. 2013;61:171-7. [PubMed ID: 23747717]. https://doi.org/10.1016/j.fct.2013.05.046.
-
8.
Hanachi P, Shemshaki A, Norouziyan S. The effect of eccentric exercise on total anti-oxidant capacity, reduced glutathione and malondialdehyde levels in active women. Zahedan J Res Med Sci. 2014;16(6):47-52.
-
9.
Wiecek M, Szymura J, Maciejczyk M, Kantorowicz M, Szygula Z. Anaerobic exercise-induced activation of antioxidant enzymes in the blood of women and men. Front Physiol. 2018;9:1006. [PubMed ID: 30140236]. [PubMed Central ID: PMC6094974]. https://doi.org/10.3389/fphys.2018.01006.
-
10.
Mestre-Alfaro A, Ferrer MD, Sureda A, Tauler P, Martínez E, Bibiloni MM, et al. Phytoestrogens enhance antioxidant enzymes after swimming exercise and modulate sex hormone plasma levels in female swimmers. Eur J Appl Physiol. 2011;111(9):2281-94. [PubMed ID: 21331628]. https://doi.org/10.1007/s00421-011-1862-y.
-
11.
Cases N, Sureda A, Maestre I, Tauler P, Aguiló A, Córdova A, et al. Response of antioxidant defences to oxidative stress induced by prolonged exercise: antioxidant enzyme gene expression in lymphocytes. Eur J Appl Physiol. 2006;98(3):263-9. [PubMed ID: 16896722]. https://doi.org/10.1007/s00421-006-0273-y.
-
12.
Park SY, Kwak YS. Impact of aerobic and anaerobic exercise training on oxidative stress and antioxidant defense in athletes. J Exerc Rehabil. 2016;12(2):113-7. [PubMed ID: 27162773]. [PubMed Central ID: PMC4849490]. https://doi.org/10.12965/jer.1632598.299.
-
13.
Bridge CA, Ferreira da Silva Santos J, Chaabène H, Pieter W, Franchini E. Physical and physiological profiles of taekwondo athletes. Sports Med. 2014;44(6):713-33. [PubMed ID: 24549477]. https://doi.org/10.1007/s40279-014-0159-9.
-
14.
Roh HT, Cho SY, So WY. Effects of regular taekwondo intervention on oxidative stress biomarkers and myokines in overweight and obese adolescents. Int J Environ Res Public Health. 2020;17(7). [PubMed ID: 32268592]. [PubMed Central ID: PMC7177505]. https://doi.org/10.3390/ijerph17072505.
-
15.
Laursen PB. Training for intense exercise performance: high-intensity or high-volume training? Scand J Med Sci Sports. 2010;20 Suppl 2:1-10. [PubMed ID: 20840557]. https://doi.org/10.1111/j.1600-0838.2010.01184.x.
-
16.
Penry JT, Wilcox AR, Yun J. Validity and reliability analysis of Cooper's 12-minute run and the multistage shuttle run in healthy adults. J Strength Cond Res. 2011;25(3):597-605. [PubMed ID: 20647946]. https://doi.org/10.1519/JSC.0b013e3181cc2423.
-
17.
Andrade VL, Zagatto AM, Kalva-Filho CA, Mendes OC, Gobatto CA, Campos EZ, et al. Running-based anaerobic sprint test as a procedure to evaluate anaerobic power. Int J Sports Med. 2015;36(14):1156-62. [PubMed ID: 26422055]. https://doi.org/10.1055/s-0035-1555935.
-
18.
Hazratian M, Taheri-Chadorneshin H, Rashidi A. The effect of one bout of intensive judo exercise on select hematological and immunological parameters in adolescent elite judo athletes. Asian J Sports Med. 2020;11(2). https://doi.org/10.5812/asjsm.101364.
-
19.
Joo MH, Maehata E, Adachi T, Ishida A, Murai F, Mesaki N. The relationship between exercise-induced oxidative stress and the menstrual cycle. Eur J Appl Physiol. 2004;93(1-2):82-6. [PubMed ID: 15243748]. https://doi.org/10.1007/s00421-004-1168-4.
-
20.
Babaei P, Rahmani-Nia F, Nakhostin B, Bohlooli S. The effect of VC on immunoendocrine and oxidative stress responses to exercise. J clin diagn res. 2009;3:1627-32.
-
21.
Skenderi KP, Tsironi M, Lazaropoulou C, Anastasiou CA, Matalas AL, Kanavaki I, et al. Changes in free radical generation and antioxidant capacity during ultramarathon foot race. Eur J Clin Invest. 2008;38(3):159-65. [PubMed ID: 18257778]. https://doi.org/10.1111/j.1365-2362.2007.01917.x.
-
22.
Waring WS, Convery A, Mishra V, Shenkin A, Webb DJ, Maxwell SR. Uric acid reduces exercise-induced oxidative stress in healthy adults. Clin Sci (Lond). 2003;105(4):425-30. [PubMed ID: 12801243]. https://doi.org/10.1042/CS20030149.
-
23.
González D, Marquina R, Rondón N, Rodríguez-Malaver AJ, Reyes R. Effects of aerobic exercise on uric acid, total antioxidant activity, oxidative stress, and nitric oxide in human saliva. Res Sports Med. 2008;16(2):128-37. [PubMed ID: 18569946]. https://doi.org/10.1080/15438620802103700.
-
24.
Wayner DD, Burton GW, Ingold KU, Barclay LR, Locke SJ. The relative contributions of vitamin E, urate, ascorbate and proteins to the total peroxyl radical-trapping antioxidant activity of human blood plasma. Biochim Biophys Acta. 1987;924(3):408-19. [PubMed ID: 3593759]. https://doi.org/10.1016/0304-4165(87)90155-3.
-
25.
Peake JM. Vitamin C: effects of exercise and requirements with training. Int J Sport Nutr Exerc Metab. 2003;13(2):125-51. [PubMed ID: 12945825]. https://doi.org/10.1123/ijsnem.13.2.125.
-
26.
Andersson H, Karlsen A, Blomhoff R, Raastad T, Kadi F. Plasma antioxidant responses and oxidative stress following a soccer game in elite female players. Scand J Med Sci Sports. 2010;20(4):600-8. [PubMed ID: 19706000]. https://doi.org/10.1111/j.1600-0838.2009.00987.x.
-
27.
Kiyici F, Kishali NF. Acute effect of intense exercises on serum superoxide dismutase, catalase and malondialdehyde levels in soccer players. J Sports Med Phys Fitness. 2012;52(1):107-11. [PubMed ID: 22327094].