Abstract
Background:
Autism spectrum is a type of developmental-neurodegenerative disease commonly reflected in social communications and associated with oxidative stress in the brain. Vitamins D and E, as strong antioxidants, may improve autistic-like behavioral disorders.Objectives:
The aim of this study was to determine the effects of vitamins D and E on pain, threshold, motor coordination, and anxiety behavior, as well as oxidative stress parameters in an autistic model in rats.Methods:
We induced an experimental model of congenital autism in the rat by injection of valproic acid (500 mg/kg) into pregnant rats on the day 12.5th of gestation. From postnatal day 30 to 60, male offspring received daily injections of vitamins D (5 µg/kg) and/or E (10 mg/kg) or vehicle. Next, their anxiety levels were assessed with the elevated plus maze and open field, pain thresholds with hot plate, motor and balance with rotarod, and spatial learning and memory with T-maze. The rat brain was then removed to measure oxidative markers, including Malondialdehyde, Glutathione, and Catalase.Results:
Results indicated that injection of valproic acid-induced autistic-like behaviors, including increased pain threshold, increased anxiety, decreased exploring behaviors, reduced balance power, and motor learning, and produced oxidative stress. Administration of vitamins D and E significantly ameliorated these symptoms.Conclusions:
These findings may indicate that vitamins D and E improved sensory, motor, anxiety, and cognitive deficits in an experimental model of autism, probably by reducing the oxidative stress and inflammatory process or maintaining balance in the neurotransmitter system.Keywords
1. Background
Autism is a neurodevelopmental disorder associated with impaired social communication and often repetitive and stereotyped behaviors (1). These conditions in rats are characterized by five main symptoms: increased irritability, high pain threshold, motor hyperactivity, repetitive motions, increased stress, and altered social behavior (2). Post-mortem brain studies of patients with autism have shown anatomical changes in areas such as the frontal cortex, limbic system, hippocampus, amygdala, and olive nucleus (3). From a pathophysiological point of view, genetic factors, immune system disorders, and maternal infectious diseases (4, 5) are involved in the occurrence of this disorder; also, elevated levels of lipid oxidation, as a marker of oxidative stress, are found in many people with autism (6).
Valproic acid (VPA)-induced autism is one of the common models in laboratory animals. Previous studies documented the similarity between this model and autism in humans in terms of impairment of the cranial nerve motor nuclei and a decrease in the total number of Purkinje cells in the cerebellum (7). The VPA serves as a standard antiepileptic drug by affecting the GABA receptor and the sodium channel, and reportedly shortens the hyperacetylation in the fetal brain, increases apoptosis, impairs nerve growth, leads to increased density of glutamatergic neurons (8-10) and decreased cell proliferation in specific areas of the brain (11, 12). On the other hand, the VPA prevents serotonergic neuronal maturation from embryonic stem cells and possibly leads to altered serotonin levels in the brain (13, 14). Children exposed to VPA during the embryonic period have been reported to have an 8-fold higher chance of being diagnosed with autism (5). No definitive cure has been recognized for autistic spectrum disorders to date. However, the most common therapies include drug therapy, behavior therapy, and sometimes polytherapy (15). The use of antioxidants has been the focus of attention in recent years. The purpose of this study was to determine the effects of vitamins D and E in reducing the symptoms of VPA-induced-autistic-like behavior in rat.
Gumpricht and Rockway reported increased oxidative stress and altered antioxidant and neuroinflammatory defense factors in children with neurodevelopmental disorders. Based on that, they suggested that omega-3 fatty acids and vitamin E could be prescribed as supplements to alleviate the motor and cognitive symptoms of diseases such as autism and Alzheimer's disease (16). On the other hand, many studies have found a decrease in vitamin E levels (16) and the capacity of glutathione (GLU), as a defense against oxidative stress, in children with autism (17).
Recent studies have also reported a decrease in vitamin D in children with autism (18, 19). This vitamin plays an important role in bone formation and development; also, its deficiency reduces cortical thickness in the brain of children with autism (20). On the other hand, vitamin D inhibits γ-glutamyl transpeptidase in the brain, the major enzyme in glutamate metabolism. Accordingly, vitamin D strengthens the brain's antioxidant defense through increased glutamate (21). Humble et al. measured the vitamin D levels in adults with schizophrenia and autism and found a decrease in vitamin D levels in these patients, a decrease in depression, and an improvement in symptoms following treatment with vitamin D (22). Evidence has shown that all vitamin supplements are likely to improve metabolic and nutritional status in autistic patients and modulate oxidative stress. Treatment with vitamins can probably maintain the antioxidant capacity against the oxidant markers (23).
Based on the above evidence, administering vitamin compounds may modify oxidative stress markers and may eventually control the symptoms of autism.
2. Objectives
This study aimed to determine the effects of vitamins D and E alone and in combination on the alleviation of motor imbalance, pain sensation, emotional and cognitive disorders, and oxidative stress markers in rat offspring induced by maternal VPA consumption as a rat model of autism.
3. Methods
3.1. Animals
This study was conducted using 120 male rat pups. The animals were kept 5-rat per cage with a 12/12 h light/dark cycle and at a temperature of 22 - 24°C with free access to adequate water and food. This study was approved by the Ethical Review Board of Semnan University of Medical Sciences, Semnan, Iran (ethical approval code: IR.SEMUMS.REC.1397.144). All experiments were conducted in accordance with the National Institutes of Health Guide for the Care and Use of Laboratory Animals.
3.2. The Procedure of VPA-Induced Autism in the Rat Offspring
Female rats (n = 20, weight 180 - 200g) were divided into two groups of normal saline (control) and those that received Valproic acid, VPA, and then allowed to mate. Pregnancy was confirmed by observation of vaginal plaque and was marked as the first day of pregnancy. Pregnant rats received intraperitoneal VPA (500 mg/kg), and control rats received an injection of normal saline on the day 12th of the pregnancy (24). We performed behavioral tests, including hotplate, rotarod, open field, elevated plus maze, and T-maze, on the 30th day after birth in order to confirm that VPA has caused autistic-like changes in offspring (VPA-offspring).
3.3. Drugs
Vitamin D (kindly provided by Iran Hormone Co., Tehran, Iran) was dissolved in 100% ethanol (1μg/μL, stock) and diluted in 5% ethanol in distilled water for intraperitoneal injection. Vitamin D was injected at a concentration of 5 µg/kg/day. Vitamin E (Sigma Co. USA) was primarily dissolved in vegetable oil. The stock solution was prepared as 1ml/1ml of ethanol 100% and then diluted in 5% ethanol in normal saline 0.9%. Vitamin E was injected at 10 mg/kg/day. The vehicle groups for vitamin D or E treatment received the same volume of ethanol 5% vehicle solution as the vitamin groups.
3.4. Behavioral Tests
All postnatal rats (saline and VPA-offspring, n = 8) underwent the following behavioral tests on days 30th and 62nd after the birth; during four weeks in between, they received treatments with vitamins D and E or their vehicle (Figure 1).
Timeline of pregnancy delivery, behavioral tests, and drug injections in a model of VPA-induced autistic-like behaviors (GD, gestation day; PND, postnatal day).
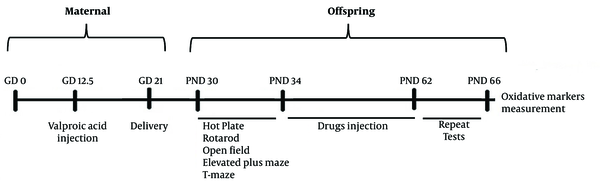
3.4.1. Hotplate Test (Armaghan Co. Mashhad, Iran)
The plate temperature was set at 52 ± 0.1°C. The time required for the animal to respond to the pain stimuli (latency) was recorded in seconds. The cut-off point was 20 seconds to prevent any tissue damage.
3.4.2. Rotarod Test (Armaghan Co. Mashhad, Iran)
Rotarod was used to examine balance, motor coordination, and skilled movement (motor learning). Through the use of this device, the animal was placed on a horizontal bar for some time before the experiment in order to assess the animal's activity balance. Then the device was set at a speed of 10 rpm, and rat motor coordination and balance were recorded.
3.4.3. Open Field Test (Ethovision, Noldus, The Netherlands, XT-7)
This device is a square open-roof box with the dimensions of 70 × 70 × 45 made of Plexiglas with a black floor, in which the rat behavior is analyzed using a camera video tracking system over a five-minute course. The field is divided into two central and peripheral areas. Animal's movement and the period spent by the animal in each area, total distance traveled, number of crossings between areas, and the average speed was recorded and measured by a CCD camera (Panasonic Inc., Japan) placed over the device and analyzed by behavior analyzing software (Ethovision, version XT7, The Netherlands). The increased time spent by the rat in the peripheral area and the decrease in the number of crossings to the central field were considered anxiety-like behavior.
3.4.4. Elevated Plus Maze Test (Borjeh-Sanaat, Tehran, Iran)
The EPM device was used to measure anxiety. The device consists of four wooden arms in the form of a cross with a central platform. The two open arms (50 × 10 cm) have no walls, but the other two enclosed arms (50 × 40 × 10 cm) have dark walls on either side. The height of this device is 50 cm from the ground. The animal was placed in the center of the cross, facing one of the open arms, and allowed to move freely in the device. A CCD camera recorded the animal’s movement (Panasonic Inc., Japan) placed over the device and measured by video tracking for automated analysis of behavior software (Ethovision, version XT7, The Netherlands). The number of crossings to open and enclosed arms and the total time spent in open and enclosed arms were measured. The entry to the open or enclosed arm was accounted when all four legs of the animal were placed inside each arm.
3.4.5. T-Maze Test (Borjeh-Sanaat, Tehran, Iran)
T-maze is made of black Plexiglas with a base and T-shaped arms with dimensions of 35 cm in length, 10 cm wide, and 15 cm in height. Horizontal sliding doors separated the arms and the start box from the central alley. A lamp positioned 2 m above provides about 50-lux illumination inside the device. Initially, the animal was made familiar with the device by being placed twice in the same arm in order to find the reward at the end of that arm. Twenty-four hours later, a memory test was performed by placing the animal in the start box for 30 s. Then, doors were opened, and the animal was free to enter any of the two arms. The time that the animal spent in each arm was recorded.
3.5. Measurements of Oxidative Stress Markers in the Brain
After the behavioral experiments on postnatal day 60, rats were sacrificed under deep CO2/O2 anesthesia (25). Their brains were dissected, and oxidative stress markers were measured. The brain was isolated, and the cortical layer of the brain was dissected completely from rostral to caudal and immersed in cold 0.9% saline, then wrapped up and kept at -70°C. Homogenizer (Polytron PT 2100, KINEMATICA AG, Switzerland) was used for the preparation of homogenates. For malondialdehyde (MDA) assays, brain tissue was homogenized (1: 10 w/v) in cold 1.15% KCl. Homogenates for glutathione (GLU) and catalase (CAT) were prepared in a ratio of 100 mg tissue to 1 ml phosphate buffer (50 mmol/L; pH 7.5) containing one mM EDTA. The supernatants were obtained following centrifugation (20,000 × g for 10 min at 4°C) and were used for biochemical analyses. The level of total protein in supernatants was determined by the Bradford method using bovine serum albumin as the standard. The oxidative stress marker MDA (a marker of lipid peroxidation) was evaluated by means of a thiobarbituric-1 acid-reacting two substances (TBARS) assay. Also, antioxidant markers, GLU and CAT, were assayed by using an ELISA kit (Bioassay Technology Laboratory, China).
3.6. Statistical Analysis
For statistical analysis of the variables in behavioral, we used three-way ANOVA, and for oxidative stress, two-way ANOVA was used following Tukey’s post-tests, provided by GB-STAT, version 5 software.
4. Results
4.1. Effects of Vitamin D and E or Combination on the Thermal Pain Threshold
Three-way ANOVA [with three variables: (1) period of investigation (pre- or post-treatment with vitamins); (2) maternal VPA or saline treatment groups; and (3) postnatal vitamin treatment groups (treatment with vitamin D or E or both or vehicle)] showed a significant effect in the period (F 1,80 = 4.9, P = 0.02), VPA treatment (F 1,80 = 32.6, P < 0.001), vitamin treatment (F 3,80 = 3.7, P = 0.01), and with significant interactions between period and VPA treatment (F 1,80 = 4.87, P = 0.03) and period and vitamin treatment (F 3,80 = 2.87, P = 0.04) on thermal pain threshold. Pairwise analysis with Tukey's post hoc test showed that the pain threshold in the VPA-offspring-vehicle group was significantly higher than in the saline-offspring group (P < 0.01). This finding indicated that maternal VPA treatment increased the pain threshold. These findings suggest that administering vitamins D and E alone or in combination reduced the thermal pain threshold in VPA offspring (Figure 2A).
Effects of vitamin D E and combined on: A, Pain responses by a hot plate; B, Motor balance by rotarod; C, Anxiety-like behavior by an open field; D, Anxiety-like behavior, by the elevated maze; and E, Spatial learning, by T-maze, in male offspring rats that prenatally exposed to VPA [data are expressed as mean ± SEM; n = 6; **P < 0.01, *P < 0.05 versus control-VEH (vehicle) group; ##P < 0.01 versus VPA-VEH group (autism); &&P < 0.01 post-treatment versus pre-treatment with vitamins; VEH, vehicle].
![Effects of vitamin D E and combined on: A, Pain responses by a hot plate; B, Motor balance by rotarod; C, Anxiety-like behavior by an open field; D, Anxiety-like behavior, by the elevated maze; and E, Spatial learning, by T-maze, in male offspring rats that prenatally exposed to VPA [data are expressed as mean ± SEM; n = 6; **P < 0.01, *P < 0.05 versus control-VEH (vehicle) group; ##P < 0.01 versus VPA-VEH group (autism); &&P < 0.01 post-treatment versus pre-treatment with vitamins; VEH, vehicle]. Effects of vitamin D E and combined on: A, Pain responses by a hot plate; B, Motor balance by rotarod; C, Anxiety-like behavior by an open field; D, Anxiety-like behavior, by the elevated maze; and E, Spatial learning, by T-maze, in male offspring rats that prenatally exposed to VPA [data are expressed as mean ± SEM; n = 6; **P < 0.01, *P < 0.05 versus control-VEH (vehicle) group; ##P < 0.01 versus VPA-VEH group (autism); &&P < 0.01 post-treatment versus pre-treatment with vitamins; VEH, vehicle].](https://services.brieflands.com/cdn/serve/31643/88979e8905212a548931f41e2501a6a0a7911015/mejrh-10-2-129627-i002-preview.png)
4.2. Effects of Vitamin D and E or Combination on Motor Activity and Balance in the Rotarod
Three-way ANOVA only showed a significant effect of the period (F1, 80 = 6.7, P = 0.01) and VPA treatment (F1, 80 = 47.66, P = 0.0001) on the rotarod motor and balance test. Pairwise analysis with Tukey's post hoc test showed that the duration of maintaining balance in rotarod was significantly shorter in VPA-offspring compared to the saline-offspring group (P < 0.01). These findings indicated that the maternal VPA treatment had caused instability in maintaining balance in offspring (Figure 2B).
4.3. Effects of Vitamin D and E or Combination on Anxiety-Like Behavior in the Open Field (OF)
Three-way ANOVA showed only a significant effect of VPA treatment (F1, 80 = 16.49, P = 0.0001) on OF activity test. Subsequent analysis with Tukey's post hoc test showed that the time spent in the center of OF in the VPA-offspring vehicle was significantly shorter than the saline-offspring group (P < 0.01). These findings indicated that the maternal VPA treatment caused anxiety-like behavior in offspring (Figure 2C).
4.4. Effects of Vitamin D and E or Combination on Anxiety-Like Behavior in the Elevated Plus Maze (EPM)
For the time rat spent in the open arm, three-way ANOVA showed a significant effect of the period (F1, 80 = 16.34, P = 0.0001), VPA treatment (F1, 80 = 14.10, P = 0.0003), vitamin treatment (F3, 80 = 3.47, P = 0.02) and a significant interaction between period and VPA treatment (F1, 80 = 9.67, P = 0.002), VPA and vitamin treatments (F3, 80 = 3.92, P = 0.01) and period, VPA and vitamin treatments (F3, 80 = 3.3, P = 0.02) on EPM activity. Pairwise analysis with Tukey's post hoc test showed that the time spent in the open arm in the VPA-offspring-vehicle group was significantly shorter than in the saline-offspring group (P < 0.01). These findings indicated that maternal VPA caused anxiety-like behavior in offspring (Figure 2D).
For the number of entries in the open arm, three-way ANOVA showed a significant effect of the period (F1, 80 = 25.57, P < 0.0001), a significant interaction between period and VPA treatment (F1, 80 = 25.5, P < 0.0001), a significant interaction between VPA and vitamin treatments (F3, 80 = 3.69, P = 0.01) and a significant interaction between period, VPA and vitamin treatments (F3, 80 = 2.78, P = 0.04). Subsequent analysis with Tukey's post hoc test showed that the number of entrances in the open arm in the autistic-like vehicle group was significantly lower than in the healthy group (P < 0.05) (Figure 2E).
4.5. Effects of Vitamin D and E or Combination on Spatial Learning in the T-Maze
Three-way ANOVA showed only a significant effect of VPA treatment (F1, 80 = 8.87, P = 0.004) on the T-maze activity test. Pairwise analysis with Tukey's post hoc test showed that the time spent in the target arm in the T-maze test in the VPA-offspring vehicle was significantly higher than the saline-offspring group (P < 0.01) (Figure 2F).
4.6. Effects of Vitamin D and E or Combination on the Levels of Brain Stress Oxidative Markers (MDA, GLU, and CAT)
For MDA activity, a two-way ANOVA (VPA and vitamin treatment groups) showed a significant effect of VPA treatment (F1, 16 = 32.04, P < 0.0001) and significant interaction between VPA and vitamin treatment groups (F3, 16 = 4.13, P = 0.02). For GLU activity, a significant effect of VPA treatment (F1, 16 = 5.44, P = 0.03) and a significant interaction between VPA and vitamin treatment groups (F3, 16 = 1.62, P = 0.02) were detected. Only the significant effect of VPA treatment (F1, 16 = 62.5, P < 0.0001) was detected for CAT activity. Pairwise analysis with Tukey's post hoc test showed that the amount of MDA in the cortex of the VPA-offspring vehicle group was significantly higher, but the amount of GLU and CAT were significantly lower than the saline-offspring group (P < 0.01) (Figure 3A - C).
Effects of vitamin D, E, and combined on brain stress oxidative markers. A, malondialdehyde; B, glutathione; C, catalase in male offspring rats that were prenatally exposed to VPA [data are expressed as mean ± SEM; n = 6; **P < 0.01; *P < 0.05 versus control-VEH; ##P < 0.01; #P < 0.05 versus VPA (autism); VEH, vehicle].
![Effects of vitamin D, E, and combined on brain stress oxidative markers. A, malondialdehyde; B, glutathione; C, catalase in male offspring rats that were prenatally exposed to VPA [data are expressed as mean ± SEM; n = 6; **P < 0.01; *P < 0.05 versus control-VEH; ##P < 0.01; #P < 0.05 versus VPA (autism); VEH, vehicle]. Effects of vitamin D, E, and combined on brain stress oxidative markers. A, malondialdehyde; B, glutathione; C, catalase in male offspring rats that were prenatally exposed to VPA [data are expressed as mean ± SEM; n = 6; **P < 0.01; *P < 0.05 versus control-VEH; ##P < 0.01; #P < 0.05 versus VPA (autism); VEH, vehicle].](https://services.brieflands.com/cdn/serve/31643/b94936a3a7ca50806494c3dd404f8a95148813cf/mejrh-10-2-129627-i003-preview.png)
5. Discussion
According to the results of this study, the VPA-offspring rats displayed autistic-like behaviors, such as increased pain tolerance, anxiety-like behaviors, and imbalance. A previous study found that the brains of rats that received VPA during fetal life had developed abnormalities, such as anatomical changes in the cerebellum, amygdala, hippocampus, frontal lobes, and parietal lobes, similar to those seen in children with autism. Therefore, the VPA model has both structural and behavioral characteristics similar to those in autism, and therefore, it is a suitable model for the assessment of autistic-like behaviors in rodents (24).
On the other hand, administering vitamins D and E for four weeks significantly improved behavioral disorders and oxidative stress markers. One common finding between different behavioral and oxidative tests in this study was that the administration of vitamins D and E, or combined, produced their effects only in rats that received VPA but not in healthy control groups. The positive effects of these vitamins have been studied so far for other disorders related to the nervous system, such as chronic stress-induced depressive-like behaviors and vitamin D's significant effect on alleviating anhedonia. This effect is strongly suggested to be related to the regulation of vitamin D on neurotransmitter systems, especially dopamine and reward systems (26, 27). Likewise, another study has explored vitamin E deficiency during development and its association with glutamate levels and anxiety related to familial ataxia in adult mice. Vitamin E supplements alleviated anxiety symptoms (28). In relation to the autism spectrum, other studies have provided evidence of vitamin E's relieving effect on VPA-induced autistic-like behaviors, such as anxiety and repetitive/stereotypic-like behaviors, and sociability impairments in rats exposed to VPA before birth (29). Also, the administration of vitamin D supplements markedly relieved repetitive behaviors and increased grooming in rat offspring treated with VPA during pregnancy (30).
In the present study, rat offspring with maternal VPA treatment displayed significantly higher pain thresholds than normal rats. Along with our result, another study also reported an increase in the pain threshold in offspring rats caused by maternal VPA treatment, which was decreased using pregabalin (31). It seems that under the influence of VPA, the nitric oxide (NO) signaling pathway is probably affected by imbalances in neurotransmitter release, immune disorders, and oxidative stress (32). Since pain sensation in the periphery depends on the presence of different local inflammatory mediators, including NO (33), therefore, an imbalance in NO production alters the threshold of pain sensation as it happens in autistic patients (34).
In this, as well as some other studies, it has been indicated that there is a relation between vitamin D and NO function, specifically in relation to neural diseases such as autism spectrum. NO directly regulate vitamin D expression through CYP27B1. On the other hand, since inflammatory factors increase the expression of inducible Nitric Oxide synthase (iNOS) and, consequently, produce NO, vitamin D modulates the inflammatory processes and reduces NO production (32).
In the present study, using rotarod, EPM, OF, and TM that measured the rat's motor balance, anxiety levels, and spatial learning/memory indicated that VPA had induced imbalance and mal-coordination, increased anxiety, and decreased level of spatial learning in the rat. Previous studies indicate that autism spectrum and animal models such as VPA-induced autistic-like behaviors produce motor deficits through damage to the cerebellum and its connections to the motor and prefrontal cortex (35). These damages are a possible reason for mal-coordination in humans and animals under a model of autistic disorders. In line with our findings, Edalatmanesh and Samimi study showed that a decrease in balance and learning in rats with the VPA model of autism indicated a probable decrease in BDNF levels or damage to Purkinje cells in the cerebellum. They used gallic acid as an antioxidant to alleviate motor disorders (36).
It is mostly indicated that the underlying reasons for motor deficiencies related to autistic syndrome are immune system malfunction, oxidative stress, and neurotransmitter imbalance (35). Studies have shown that patients with autism have higher plasma levels of autoimmune indicators such as anti-nuclear antibodies as well as pro-inflammatory cytokines such as IL-6 and tumor necrosis factor-alpha (TNF-α) in different tissues, which may be associated with cognitive impairment in these patients. An increase in oxidative stress and mitochondrial dysfunction can produce neural damage. Also, autistic disorder is associated with a deficiency in GABA neurotransmitters as well as serotonin and dopamine (37).
Several reports also show that autism and the VPA model of autistic disorder increase excitability and anxiety in humans and animals (31, 38). Some relate the anxiety to hyperactivation of the amygdala due to alteration in GABAergic transmission or increased expression of GluN2A- and GluN2B-NMDA receptors and amplified long-term potentiation (LTP) and increased excitatory synapses in the cortex (38). Some studies suggest that the reason behind the delayed development of the sensory system, as well as anxiety and spatial learning in autistic-like syndromes, is related to an imbalance in GABA production and release in different parts of the cortex as well as the hippocampus and cerebellum. Using a VPA model of disease, they revealed decreased expression of glutamic acid decarboxylase 67, GAD67, expression in the hippocampus and cerebellum from adolescence to adulthood and temporal cortex in adulthood, while increased expression was found in the prefrontal cortex from adolescence to adulthood (39). Still, other studies are focusing on oxidation stress and immune system dysfunction as potential reasons for autistic behaviors, such as anxiety and learning deficits (40).
In our study, administering vitamins D and E effectively improved rat function in rotarod, anxiety, and spatial learning tests. Vitamins D and E have powerful antioxidants (28, 37), anti-inflammatory (41), and neurotransmitter regulatory effects (26, 37). For the GABA inhibitory system, vitamin E creates a balance in activates of the enzymes converting glutamate to GABA in different brain regions (42); also, mice with vitamin D deficiency have shown a large decrease in GAD 65/67 expression in the whole brain. The balance in the activity of the GABA inhibitory system has an important effect on the neurogenesis and development of the brain (43). Therefore, it can be strongly suggested that the improvement in movement and balance, as well as anxiety and spatial learning deficits observed in the VPA-induced autistic behaviors, are related to both vitamins, and since the action of each vitamin has been some overlapped with another (like both acting as antioxidants), maybe with a different mechanism of action, that might be the reason for the lack of augmented response seen, when both were administered together.
Our findings in the present study indicated that the levels of GLU and CAT were decreased, and the level of MDA was increased in the brains of VPA-offspring, while the administration of vitamins D and E decreased the brain levels of MDA. Previous studies indicate that increased pro-oxidants such as NO and decreased antioxidants such as GLU can cause oxidative stress. Increased oxidative stress leads to membrane lipid defects, mitochondrial dysfunction, inflammation, and immunodeficiency in children with autism, resulting in behavioral, sleep, and gastrointestinal disorders. On the other hand, clinical trials documented the improvement in the behaviors of children with autism undergoing treatment with antioxidants (6). Similarly, in another study, administrating piperin as an antioxidant agent adjusted the levels of the oxidative factor, MDA, and antioxidative agents CAT and GLU in a model of VPA-induced autism (44). Ghanizadeh et al. investigated various factors affecting the oxidative stress level that increased and decreased GLU and SOD levels in children with autism. They also suggested antioxidants as important factors in the treatment of autism (45).
Since oxidative stress is one of the major factors in the pathophysiology of autism, there have been various studies investigating the effects of different types of antioxidants as treatment options. Among all, vitamins, specifically vitamin D and E, have received special interest recently; there have been a good number of studies investigating their antioxidative effects under conditions that markedly raise oxidative stress incidents, such as chronic psychological stress. Under a model of chronic mild stress, a marked increase in the pro-inflammatory factors happened, along with a significant reduction in the SOD and glutathione peroxidase (GPx) as indigenous antioxidants. Administration of vitamin D reduced the pro-inflammatory cytokines and raised the suppressed levels of SOD and GPx (41). Likewise, Kocovska et al. investigated the association between vitamin D and autism by reviewing 15 years of animal, molecular, cellular and physiological, post-mortem brain, and neuronal imaging studies related to the role of vitamin D in autism and suggested that patients with autism are likely to be exposed to vitamin D deficiency, which might have raised the odds ratio of autistic behaviors along with other factors (18). On the other hand, Gumpricht and Rockway investigated the role of omega-3 fatty acids and Tocotrienol-rich vitamin E in reducing symptoms of Alzheimer's disease and neurodevelopmental disorders, such as autism. Their results showed an increase in the level of oxidative stress and a change in antioxidant defense factors and neuroinflammation in autistic children. Their study suggested that omega-3 fatty acids and vitamin E could be used as supplements to alleviate behavioral and cognitive symptoms in neurodevelopmental and neurodegenerative disorders such as autism and Alzheimer's disease (16). Also, another study in 2010 described the role of Thai brown rice in reducing oxidative stress, and the results showed that it reduced MDA levels as a marker of oxidative stress. Thai brown rice contains high levels of vitamins D and E (46).
This study, for the first time, investigated the effects of both vitamins D and E as strong antioxidants and anti-inflammatory agents in alleviating some disturbing syndromes related to maternal VPA administration in male offspring, such as an increase in pain threshold (indicating a high risk of tissue damage because of decreased levels of sense of pain), motor balance incoordination, anxiety, and learning deficiencies. By referring to previous studies, indicated effects may also be implied for human beings. Further investigation strongly suggests understanding the mechanism underlying their actions.
5.1. Conclusion
In this study, the model of maternal VPA-induced autistic-like behaviors in the rat male offspring; elevated pain threshold, produced motor imbalance, increased anxiety, and diminished spatial learning. The goal of this study was to evaluate the effect of vitamins D and E on the alleviation of maternal VPA-induced syndromes, as well as the levels of oxidative stress in offspring. Both vitamins, individually or in combination, regulated the rat response to thermal pain, motor balance, levels of anxiety, and spatial learning in rat offspring. This is the first study showing the effects of both vitamins D and E, as strong antioxidants, on sensory, motor, mood, and cognitive deficiencies and oxidative stress induced by maternal VPA consumption in offspring. This study also confirmed this suggestion that using both vitamins (perhaps as supplements) doesn't have an augmented effect in relieving the deficiencies.
5.2. Limitations
The main limitation of this study was the lack of accessibility to most of the tools and devices used for autistic-like behavior studies, like those that are used for investigating the social interaction deficit.
References
-
1.
Landa RJ. Diagnosis of autism spectrum disorders in the first 3 years of life. Nat Clin Pract Neurol. 2008;4(3):138-47. [PubMed ID: 18253102]. https://doi.org/10.1038/ncpneuro0731.
-
2.
Schneider T, Roman A, Basta-Kaim A, Kubera M, Budziszewska B, Schneider K, et al. Gender-specific behavioral and immunological alterations in an animal model of autism induced by prenatal exposure to valproic acid. Psychoneuroendocrinology. 2008;33(6):728-40. [PubMed ID: 18396377]. https://doi.org/10.1016/j.psyneuen.2008.02.011.
-
3.
Bauman ML, Kemper TL. Neuroanatomic observations of the brain in autism: a review and future directions. Int J Dev Neurosci. 2005;23(2-3):183-7. [PubMed ID: 15749244]. https://doi.org/10.1016/j.ijdevneu.2004.09.006.
-
4.
Patterson PH. Modeling autistic features in animals. Pediatr Res. 2011;69(5 Pt 2):34R-40R. [PubMed ID: 21289542]. [PubMed Central ID: PMC3088489]. https://doi.org/10.1203/PDR.0b013e318212b80f.
-
5.
Chaste P, Leboyer M. Autism risk factors: genes, environment, and gene-environment interactions. Dialogues Clin Neurosci. 2012;14(3):281-92. [PubMed ID: 23226953]. [PubMed Central ID: PMC3513682]. https://doi.org/10.31887/DCNS.2012.14.3/pchaste.
-
6.
Chauhan A, Chauhan V. Oxidative stress in autism. Pathophysiology. 2006;13(3):171-81. [PubMed ID: 16766163]. https://doi.org/10.1016/j.pathophys.2006.05.007.
-
7.
Martin LA, Goldowitz D, Mittleman G. Repetitive behavior and increased activity in mice with Purkinje cell loss: a model for understanding the role of cerebellar pathology in autism. Eur J Neurosci. 2010;31(3):544-55. [PubMed ID: 20105240]. [PubMed Central ID: PMC2846709]. https://doi.org/10.1111/j.1460-9568.2009.07073.x.
-
8.
Kim KC, Choi CS, Kim JW, Han SH, Cheong JH, Ryu JH, et al. MeCP2 Modulates Sex Differences in the Postsynaptic Development of the Valproate Animal Model of Autism. Mol Neurobiol. 2016;53(1):40-56. [PubMed ID: 25404090]. https://doi.org/10.1007/s12035-014-8987-z.
-
9.
Fukuchi M, Nii T, Ishimaru N, Minamino A, Hara D, Takasaki I, et al. Valproic acid induces up- or down-regulation of gene expression responsible for the neuronal excitation and inhibition in rat cortical neurons through its epigenetic actions. Neurosci Res. 2009;65(1):35-43. [PubMed ID: 19463867]. https://doi.org/10.1016/j.neures.2009.05.002.
-
10.
Kim KC, Lee DK, Go HS, Kim P, Choi CS, Kim JW, et al. Pax6-dependent cortical glutamatergic neuronal differentiation regulates autism-like behavior in prenatally valproic acid-exposed rat offspring. Mol Neurobiol. 2014;49(1):512-28. [PubMed ID: 24030726]. https://doi.org/10.1007/s12035-013-8535-2.
-
11.
Kataoka S, Takuma K, Hara Y, Maeda Y, Ago Y, Matsuda T. Autism-like behaviours with transient histone hyperacetylation in mice treated prenatally with valproic acid. Int J Neuropsychopharmacol. 2013;16(1):91-103. [PubMed ID: 22093185]. https://doi.org/10.1017/S1461145711001714.
-
12.
Phiel CJ, Zhang F, Huang EY, Guenther MG, Lazar MA, Klein PS. Histone deacetylase is a direct target of valproic acid, a potent anticonvulsant, mood stabilizer, and teratogen. J Biol Chem. 2001;276(39):36734-41. [PubMed ID: 11473107]. https://doi.org/10.1074/jbc.M101287200.
-
13.
Miyazaki K, Narita N, Narita M. Maternal administration of thalidomide or valproic acid causes abnormal serotonergic neurons in the offspring: implication for pathogenesis of autism. Int J Dev Neurosci. 2005;23(2-3):287-97. [PubMed ID: 15749253]. https://doi.org/10.1016/j.ijdevneu.2004.05.004.
-
14.
Narita N, Kato M, Tazoe M, Miyazaki K, Narita M, Okado N. Increased monoamine concentration in the brain and blood of fetal thalidomide- and valproic acid-exposed rat: putative animal models for autism. Pediatr Res. 2002;52(4):576-9. [PubMed ID: 12357053]. https://doi.org/10.1203/00006450-200210000-00018.
-
15.
Aali S, AminYazdi SA, Abdekhodaei MS, Moharreri F, Ghanaei Chamanabad A. The profile of functional emotional development of children with autism spectrum disorders from the perspective of developmental, individual differences (DIR), relationship-based approach. Int J Pediatr. 2014;2(4.1):245-56.
-
16.
Gumpricht E, Rockway S. Can omega-3 fatty acids and tocotrienol-rich vitamin E reduce symptoms of neurodevelopmental disorders? Nutrition. 2014;30(7-8):733-8. [PubMed ID: 24631384]. https://doi.org/10.1016/j.nut.2013.11.001.
-
17.
Chauhan A, Audhya T, Chauhan V. Brain region-specific glutathione redox imbalance in autism. Neurochem Res. 2012;37(8):1681-9. [PubMed ID: 22528835]. https://doi.org/10.1007/s11064-012-0775-4.
-
18.
Kocovska E, Fernell E, Billstedt E, Minnis H, Gillberg C. Vitamin D and autism: clinical review. Res Dev Disabil. 2012;33(5):1541-50. [PubMed ID: 22522213]. https://doi.org/10.1016/j.ridd.2012.02.015.
-
19.
Vinkhuyzen AAE, Eyles DW, Burne THJ, Blanken LME, Kruithof CJ, Verhulst F, et al. Gestational vitamin D deficiency and autism spectrum disorder. BJPsych Open. 2017;3(2):85-90. [PubMed ID: 28446959]. [PubMed Central ID: PMC5385921]. https://doi.org/10.1192/bjpo.bp.116.004077.
-
20.
Molloy CA, Kalkwarf HJ, Manning-Courtney P, Mills JL, Hediger ML. Plasma 25(OH)D concentration in children with autism spectrum disorder. Dev Med Child Neurol. 2010;52(10):969-71. [PubMed ID: 20497452]. [PubMed Central ID: PMC2939162]. https://doi.org/10.1111/j.1469-8749.2010.03704.x.
-
21.
Kalueff AV, Eremin KO, Tuohimaa P. Mechanisms of neuroprotective action of vitamin D(3). Biochemistry (Mosc). 2004;69(7):738-41. [PubMed ID: 15310271]. https://doi.org/10.1023/b:biry.0000040196.65686.2f.
-
22.
Humble MB, Gustafsson S, Bejerot S. Low serum levels of 25-hydroxyvitamin D (25-OHD) among psychiatric out-patients in Sweden: relations with season, age, ethnic origin and psychiatric diagnosis. J Steroid Biochem Mol Biol. 2010;121(1-2):467-70. [PubMed ID: 20214992]. https://doi.org/10.1016/j.jsbmb.2010.03.013.
-
23.
Adams JB, Audhya T, McDonough-Means S, Rubin RA, Quig D, Geis E, et al. Nutritional and metabolic status of children with autism vs. neurotypical children, and the association with autism severity. Nutr Metab (Lond). 2011;8(1):34. [PubMed ID: 21651783]. [PubMed Central ID: PMC3135510]. https://doi.org/10.1186/1743-7075-8-34.
-
24.
Schneider T, Przewlocki R. Behavioral alterations in rats prenatally exposed to valproic acid: animal model of autism. Neuropsychopharmacology. 2005;30(1):80-9. [PubMed ID: 15238991]. https://doi.org/10.1038/sj.npp.1300518.
-
25.
Kohler I, Meier R, Busato A, Neiger-Aeschbacher G, Schatzmann U. Is carbon dioxide (CO2) a useful short acting anaesthetic for small laboratory animals? Lab Anim. 1999;33(2):155-61. [PubMed ID: 10780819]. https://doi.org/10.1258/002367799780578390.
-
26.
Sedaghat K, Yousefian Z, Vafaei AA, Rashidy-Pour A, Parsaei H, Khaleghian A, et al. Mesolimbic dopamine system and its modulation by vitamin D in a chronic mild stress model of depression in the rat. Behav Brain Res. 2019;356:156-69. [PubMed ID: 30144460]. https://doi.org/10.1016/j.bbr.2018.08.020.
-
27.
Pertile RAN, Cui X, Hammond L, Eyles DW. Vitamin D regulation of GDNF/Ret signaling in dopaminergic neurons. FASEB J. 2018;32(2):819-28. [PubMed ID: 29018141]. https://doi.org/10.1096/fj.201700713R.
-
28.
Desrumaux CM, Mansuy M, Lemaire S, Przybilski J, Le Guern N, Givalois L, et al. Brain Vitamin E Deficiency During Development Is Associated With Increased Glutamate Levels and Anxiety in Adult Mice. Front Behav Neurosci. 2018;12:310. [PubMed ID: 30618663]. [PubMed Central ID: PMC6297247]. https://doi.org/10.3389/fnbeh.2018.00310.
-
29.
Alinaghi Langari A, Nezhadi A, Kameshki H, Jorjafki SM, Mirhosseini Y, Khaksari M, et al. The protective effect of prenatally administered vitamin E on behavioral alterations in an animal model of autism induced by valproic acid. Toxin Rev. 2020;40(4):676-80. https://doi.org/10.1080/15569543.2020.1747495.
-
30.
Du L, Zhao G, Duan Z, Li F. Behavioral improvements in a valproic acid rat model of autism following vitamin D supplementation. Psychiatry Res. 2017;253:28-32. [PubMed ID: 28324861]. https://doi.org/10.1016/j.psychres.2017.03.003.
-
31.
Shamsi Meymandi M, Sepehri G, Moslemizadeh A, Vakili Shahrbabaki S, Bashiri H. Prenatal pregabalin is associated with sex-dependent alterations in some behavioral parameters in valproic acid-induced autism in rat offspring. Int J Dev Neurosci. 2020;80(6):500-11. [PubMed ID: 32588482]. https://doi.org/10.1002/jdn.10046.
-
32.
Wang B, Dong H, Li H, Yue X, Xie L. A Probable Way Vitamin D Affects Autism Spectrum Disorder: The Nitric Oxide Signaling Pathway. Front Psychiatry. 2022;13:908895. [PubMed ID: 35722582]. [PubMed Central ID: PMC9199365]. https://doi.org/10.3389/fpsyt.2022.908895.
-
33.
Freire MA, Guimaraes JS, Leal WG, Pereira A. Pain modulation by nitric oxide in the spinal cord. Front Neurosci. 2009;3(2):175-81. [PubMed ID: 20011139]. [PubMed Central ID: PMC2751623]. https://doi.org/10.3389/neuro.01.024.2009.
-
34.
Allely CS. Pain sensitivity and observer perception of pain in individuals with autistic spectrum disorder. ScientificWorldJournal. 2013;2013:916178. [PubMed ID: 23843740]. [PubMed Central ID: PMC3697411]. https://doi.org/10.1155/2013/916178.
-
35.
Morakotsriwan N, Wattanathorn J, Kirisattayakul W, Chaisiwamongkol K. Autistic-Like Behaviors, Oxidative Stress Status, and Histopathological Changes in Cerebellum of Valproic Acid Rat Model of Autism Are Improved by the Combined Extract of Purple Rice and Silkworm Pupae. Oxid Med Cell Longev. 2016;2016:3206561. [PubMed ID: 27034733]. [PubMed Central ID: PMC4806649]. https://doi.org/10.1155/2016/3206561.
-
36.
Edalatmanesh MA, Samimi P. The Effect of Gallic Acid on Motor Learning and Cerebellar Level of Brain Derived Neurotrophic Factor in a Rat Model of Autism. Neurosci J Shefaye Khatam. 2018;6(4):5-13. https://doi.org/10.29252/shefa.6.4.5.
-
37.
Wang J, Huang H, Liu C, Zhang Y, Wang W, Zou Z, et al. Research Progress on the Role of Vitamin D in Autism Spectrum Disorder. Front Behav Neurosci. 2022;16:859151. [PubMed ID: 35619598]. [PubMed Central ID: PMC9128593]. https://doi.org/10.3389/fnbeh.2022.859151.
-
38.
Gao X, Zheng R, Ma X, Gong Z, Xia D, Zhou Q. Elevated Level of PKMzeta Underlies the Excessive Anxiety in an Autism Model. Front Mol Neurosci. 2019;12:291. [PubMed ID: 31849605]. [PubMed Central ID: PMC6893886]. https://doi.org/10.3389/fnmol.2019.00291.
-
39.
Hou Q, Wang Y, Li Y, Chen D, Yang F, Wang S. A Developmental Study of Abnormal Behaviors and Altered GABAergic Signaling in the VPA-Treated Rat Model of Autism. Front Behav Neurosci. 2018;12:182. [PubMed ID: 30186123]. [PubMed Central ID: PMC6110947]. https://doi.org/10.3389/fnbeh.2018.00182.
-
40.
Pangrazzi L, Balasco L, Bozzi Y. Oxidative Stress and Immune System Dysfunction in Autism Spectrum Disorders. Int J Mol Sci. 2020;21(9). [PubMed ID: 32384730]. [PubMed Central ID: PMC7247582]. https://doi.org/10.3390/ijms21093293.
-
41.
Sedaghat K, Naderian R, Pakdel R, Bandegi AR, Ghods Z. Regulatory effect of vitamin D on pro-inflammatory cytokines and anti-oxidative enzymes dysregulations due to chronic mild stress in the rat hippocampus and prefrontal cortical area. Mol Biol Rep. 2021;48(12):7865-73. [PubMed ID: 34642830]. https://doi.org/10.1007/s11033-021-06810-2.
-
42.
Pereira I, Dessai SN, Pinto A. Vitamin E-Induced Changes in Glutamate and GABA Metabolizing Enzymes of Chick Embryo Cerebrum. ISRN Neurol. 2013;2013:851235. [PubMed ID: 23984094]. [PubMed Central ID: PMC3747438]. https://doi.org/10.1155/2013/851235.
-
43.
Groves NJ, Burne THJ. The impact of vitamin D deficiency on neurogenesis in the adult brain. Neural Regen Res. 2017;12(3):393-4. [PubMed ID: 28469647]. [PubMed Central ID: PMC5399710]. https://doi.org/10.4103/1673-5374.202936.
-
44.
Pragnya B, Kameshwari JS, Veeresh B. Ameliorating effect of piperine on behavioral abnormalities and oxidative markers in sodium valproate induced autism in BALB/C mice. Behav Brain Res. 2014;270:86-94. [PubMed ID: 24803211]. https://doi.org/10.1016/j.bbr.2014.04.045.
-
45.
Ghanizadeh A, Akhondzadeh S, Hormozi M, Makarem A, Abotorabi-Zarchi M, Firoozabadi A. Glutathione-related factors and oxidative stress in autism, a review. Curr Med Chem. 2012;19(23):4000-5. [PubMed ID: 22708999]. https://doi.org/10.2174/092986712802002572.
-
46.
Prasit S, Sirichet R, Chaiyavat C, Suda R. High levels of 25-hydroxyvitamin D3 [25 (OH) D3] and α-tocopherol prevent oxidative stress in rats that consume Thai brown rice. J Med Plant Res. 2010;4(2):120-4.