Abstract
Background:
Following an anterior cruciate ligament (ACL) rupture, proprioceptive deficiency may be responsible for functional balance defects and joint instability. Recently, using transcranial direct current stimulation (tDCS) or biofeedback has been recommended for athletes with ACL rupture.Objectives:
This study aimed to evaluate the effects of tDCS and biofeedback modalities while performing contraction exercises in athlete with an ACL rupture.Methods:
Thirty-three athletes with ACL rupture were randomly divided into three groups of tDCS, biofeedback, and control, with 11 people in each group. All groups underwent a ten-session training of intermittent contractions of the lower extremity muscles in a standing position at different knee angles, including 30, 45, and 90 degrees of knee flexion during four weeks. The control group received no tDCS or biofeedback modalities except the contraction exercises. All subjects were evaluated both before and at the end of interventions. The proprioception measured as rate of absolute error in the knee joint reconstruction was assessed by the digital photography technique at knee flexion angles of 30, 45, and 90 degrees. The functional balance was assessed by the star excursion balance test in eight different directions. All measurements were carried out on the injured limb.Results:
The results showed no significant difference among three groups regarding all variables related to the proprioception and functional balance (P > 0.05). Intra-group comparison (before and after intervention) indicated that biofeedback significantly improved both the functional balance in all directions and the proprioception of the injured knee at three knee flexion angles (P < 0.05). Moreover, tDCS significantly improved the functional balance in six directions, except anterior-lateral and posterior, and also improved the proprioception at all angles of knee flexion.Conclusions:
Although the results did not show a large difference among groups, both tDCS and biofeedback modalities, along with intermittent isometric exercises, may have reduced knee absolute error at the knee flexion angles of 30, 45, and 90 degrees as well as improved the functional balance. Therefore, it was recommended that these modalities should be included in rehabilitation program targeting ACL-deficient knee subjects.Keywords
Anterior Cruciate Ligament tDCS, Biofeedback Proprioception Balance Performance
1. Background
The anterior cruciate ligament (ACL) is responsible for maintaining the static and dynamic stability of knee joint in the sagittal plane. The ACL not only has a mechanical role in inhibiting excessive forward movement of the tibia and its rotational motion relative to the femur, but also plays an important role in transmitting information about joint position and movement due to its many mechanical receptors (1). ACL injury is one of the most common knee injuries during sport activities, with a prevalence of 30 per 100,000 in the United States (2). Injury to the ACL could lead to damage to proprioception as joint position sense, thereby impairing the activity of mechanical receptors in transmitting information to the central nervous system (3).
The proprioception is responsible for perceiving movement and positioning different parts of the body without using eyesight (3, 4). Proprioceptive information receiving tone and length of muscles and position of joints along with vestibular and visual information is needed to control posture and maintain balance in a particular position or during dynamic movement (5, 6). Also, balance is one of the indicators of functional independence to maintain the center of gravity of body on the base of support and to create appropriate muscular responses by integrating sensory information from a body position in space (7). Impairment of proprioception, especially in athletes, impairs functional balance, which is a prerequisite for dynamic and static activities (8). According to available evidence, a decrease of postural control function in people with ACL injury is due to a reduction of sensory information and sensory stimulation from position of joint angle in the injured leg (9, 10). Therefore, the ACL is more than a mechanical limiter of the knee movement and is known as an important motor sensory component for local control (11).
Following ACL rupture, treatments seek to achieve optimal range of motion, maintain, and promote joint stability, strengthen knee muscles, and achieve normal neuromuscular control. In recent years, attention to understanding the mechanisms related to neuromuscular control and dynamic stability of the knee in patients with ACL defects has been increasing (12, 13). In the rehabilitation process, however, improving proprioceptive and neuromuscular control is one of the little-known issues (14). Today, the use of new technologies such as neurofeedback, biofeedback, and transcranial direct current stimulation (tDCS) as safe and painless methods have been considered to improve brain function, proprioception, and balance (15-17). Biofeedback is a technique used to facilitate normal movement patterns after injury (18). This technique provides biological information to clients in real time, which can sometimes refer to external or complementary feedback. Therefore, it provides additional information for the user. Electromyography biofeedback is a method of muscle retraining by creating a new feedback system that converts myoelectric signals in muscle into visual and auditory signals (19). These feedbacks permit for alterations in neuromuscular control, allowing a patient to reach a desired goal of muscular contraction (20). Electromyography biofeedback is often utilized along with conventional rehabilitation techniques to improve strength, balance, and proprioception (21) by facilitating patients’ compliance, modulating muscle activation, and improving neuromuscular control (22).
Another new intervention in the rehabilitation of neuromuscular disorder used along with conventional rehabilitation techniques is the tDCS. The tDCS is a non-invasive and safe method of brain stimulation, that uses low-intensity electrical current directly to desired areas of the brain through electrodes on the skull surface and can alter cortical irritability by modulating the resting potential of the neuronal membrane (23). Electric stimulation works by changing the resting potential of cortical neurons. Preliminary studies on animal specimens have indicated a change in the activity of the cerebral cortex due to electric current in the form of a decrease or increase in excitability, depending on the conditions of the electric current transmitted (24). TDCS can directly regulate brain waves by means of an electric current delivered at a certain frequency, and if it is prolonged enough, it can also have neuroplasticity effects (25).
2. Objectives
Although ACL damage initially causes a peripheral disorder, it can functionally affect the central information processing system (26). The development of effective modalities such as biofeedback on the proprioception and balance along with traditional rehabilitation has been reported to in improve the performance following ACL injury (27, 28). For the TDCS as a safe and painless method to stimulate the central motor cortex in changing brain plasticity, no research has been conducted in comparison with modalities that affect the peripheral nervous system, such as biofeedback after ACL injury. Assuming the effectiveness of training methods, therefore, this study aimed to answer the question of whether a ten-session period of standing at different angles of knee while performing contraction exercises of lower extremity muscles along with the biofeedback or TDCS interventions can improve the proprioceptive and functional balance of athletes with complete and isolated rupture of the ACL, as well as to find any differences between tDCS and biofeedback regarding these outcomes.
3. Methods
3.1. Participants
Using convenience sampling method, 33 male athletes with ACL injuries were recruited in this quasi-experimental study. Inclusion criteria were subjects with an isolated and complete ACL injury in the 18 - 30 age range injured for at least four months and diagnosed with an ACL rupture by orthopedist using MRI, no history of neuromuscular diseases affecting balance, and no length discrepancy between two limbs. Also, the use of sleeping pills and sedatives was controlled before the tests. The subjects with meniscus rupture, lateral ligament injury, cartilage injury, signs of injury to the opposite knee more than 36 months after the injury, and previous ACL surgery were excluded from the study. Also, individuals with psychological problems, epilepsy, and migraine were excluded. These individuals were selected from the surgical waiting list of the Akhtar Hospital, Shahid Beheshti University of Medical Sciences. All subjects were matched based on their age and then randomly divided into three tDCS, biofeedback, and control groups. This study was approved by ethical committee of Shahid Beheshti University of Medical Sciences (IR.SBMU.RETECH.REC.1398.603). Demographic characteristics of the subjects are shown in Table 1.
Groups | Age (y) | Height (m) | Weight (kg) | BMI (kg/m2) |
---|---|---|---|---|
Control group (n=11) | 30.25 ± 1.25 | 1.78 ± 5.35 | 78.75 ± 5.37 | 24.62 ± 1.75 |
Biofeedback group (n=11) | 33.18 ± 7.63 | 1.78 ± 3.53 | 81.54 ± 6.8 | 25.58 ± 2.45 |
tDCS group (n=11) | 29.91 ± 8.75 | 1.77 ± 7.26 | 81.37 ± 16.36 | 25.85 ± 4.49 |
Regarding the 95% confidence level and 80% power as well as considering the mean and standard deviation (SD) of Star Excursion Balance Test (SEBT) for the anterior-lateral direction on the male athletes in a previous study (29), the number of subjects was calculated as 11 per group by:
3.2. Outcome Measures
Primary outcome measures were the knee absolute error for 30, 45, and 90 degrees as proprioception, and the functional balance using the SEBT in eight different directions.
3.2.1. Proprioception Analysis
To evaluate the joint proprioception in each group, the method of reconstructing the target angle in the standing position was performed using a system consisting of skin marking and digital photography. For marking, participants were placed in a supine, comfortable position on the treatment bed, and 3 colored skin markers with a diameter of 4 cm were taped on the areas, including one to the upper 1/4 of the line between the greater trochanter and the middle of the lateral knee joint, the second to the upper part of the lateral malleolus, and the third to the upper part of the popliteal fossa along the upper edge of the patella. A smart phone was placed at a distance of 185 cm from the person, 65 cm from the ground, and parallel to the knee joint so that all three points were recognizable, and then the pictures were taken. The difference between the test and reconstruction angles was assumed as an absolute error. The absolute errors, as the amount of deviation from the target angle in reconstructing the angles of motion without taking into account the deviation direction, were analyzed with Digimizer image analysis software version 5.3.4.
3.2.2. Functional Balance Analysis
To evaluate the functional balance, the SEBT (30) was utilized to measure the maximum distance in eight directions including anterior, anterior-lateral, lateral, posterior-lateral, posterior, posterior-medial, medial, and posterior-medial on injured limb. The subject performed each direction three times, and their averages were calculated and divided by the leg length in centimeters, then multiplied by one hundred to obtain the achievement distance in percentage of the leg length.
3.3. Procedure
All individuals carried out the isometric contraction exercises in the standing position of two legs and one leg at three knee flexion angles of 30, 45, and 90 degrees, according to the evidence (31). All tests of the proprioception and functional balance were performed on injured limb by a trained examiner (other than the researcher) in one-leg position. The control group received only the rehabilitation exercises without any electromyography biofeedback or tDCS. All groups were subjected to interventions for a ten-session period during four weeks. After the initial evaluation of the subjects, the pre-study tests (baseline) were performed, and the results were recorded. The second tests were carried out when the last session was finished.
Intervention in tDCS group was performed in a separate room without noise in the biomechanics laboratory. In this research, the ActivaDose tDCS device made by Active Tek Company from United States was used. This device has the ability to increase the current intensity up to 5 milliamperes. For the present study, the current intensity was set on 1 milliampere. It includes a small monitor screen with the adjustment buttons below it, two anode (red) and cathode (black) electrodes, two square rubber sheets (to place the head electrode inside), two sponge pads, and two bands with strap to keep the pads on the head. To perform the intervention, the subjects sat on a chair. After connecting the electrodes to the device and placing them inside the sponge pad, the stimulation points of subjects’ head was determined according to the international 10 - 20 system (32). We measured from the middle of the nasal to the middle and end of the occiput with a meter and started from the nasal part and marked 20% to the back to reach the Fz area. Then, we marked another 20% back to the Cz area, another 20% back to Pz, and again 20% to the back to reach the middle of O1 and O2 (occipital cortex) (Figure 1). We fixed a pad with the anode electrode in the O1 region and a pad with the cathode electrode in the O2 region, both using a strap pad (Figure 2). For the tDCS group, it was stimulated for 20 minutes. If the person was in the control group, the current was cut off after 30 seconds, but the electrodes remained on the head for 20 minutes, and the subject was unaware of the current interruption during the intervention. During tDCS intervention, isometric contraction exercises were performed in the standing position of two legs and one leg at angles of 30, 45, and 90 degrees (33-35).
Electrode sites in tDCS
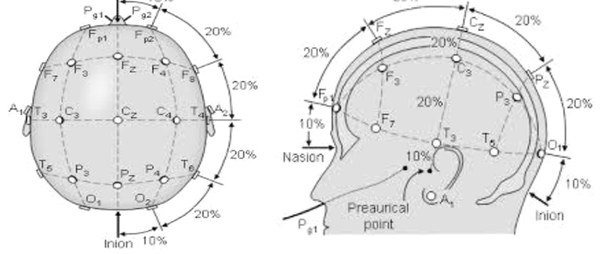
Placement of anode and cathode electrodes
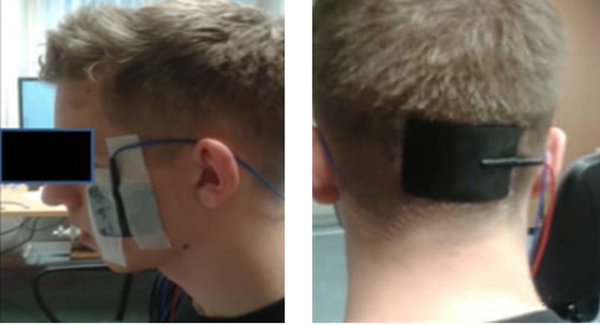
Intervention in biofeedback group was performed through the electromyography biofeedback as a recording in which the person received visual and auditory feedback via computer (Figure 3). In this study, a 5-channel biofeedback device from ProComp5 thought Technology Canada model SA7525 with serial number CB1825, BioGraph Infiniti Software, and EMG MyoScan-Pro™ sensors -T9401M-60 were utilized. After launching the device and connecting the electromyography surface electrodes to the vastus medialis muscle, the baseline electrical activity of this muscle was recorded. With standing on one leg and two legs and with the maximum isometric contraction of the knee in three angles of 30, 45, and 90 degrees, the person performed it for 10 seconds and then rested for the same time period, and this rhythm was repeated three times. During every 10 seconds of maximum isometric knee contraction, the mean value of EMG signals was recorded. Through visual feedback received by EMG signals, individuals had to increase the maximum threshold EMG value (27, 36).
Placement of electrodes on the patient's leg by biofeedback
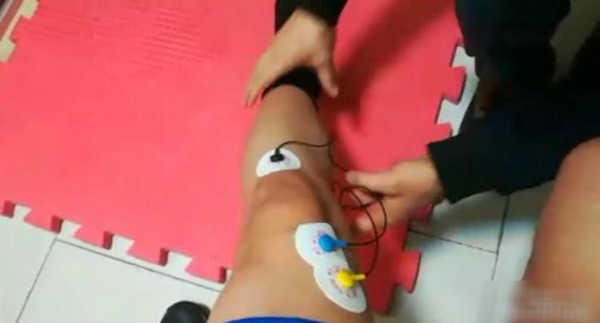
3.4. Statistical Analysis
The normality of the data distribution was evaluated using Shapiro Wilk test. To perform a pre-and post-intervention inter-group comparison, one-way ANOVA was used; and for a pre-and post-intervention intra-group comparison, Bonferroni test was used. All data were analyzed with SPSS software, version 22, at a significant level of P ≤ 0.05.
4. Results
4.1. Inter-group Comparison
There was no significant difference between three groups in terms of the subjects’ demographic characteristics (P > 0.05, Table 1). The inter-group comparison indicated no significant differences among three groups after the interventions regarding the functional balance outcome on SEBT in any eight different directions (P > 0.05, Table 2). Regarding the proprioception, no significant difference was also found among three groups in knee absolute error in 30, 45, and 90 degrees (P > 0.05, Table 3).
Comparison of Functional Balance in 8 Different Directions in Three Groups; Control, Biofeedback and tDCS Group Pre and Post Intervention in People with ACL Rupture
Directions of SEBT and Groups | Pre-intervention | Post-intervention | Differences | P-Value | P-Value (Inter-group Comparison) |
---|---|---|---|---|---|
Anterior | 0.3 | ||||
Control | 84.8 ± 8.76 | 86.3 ± 7.93 | 1.50 ± 0.83 a | 0.17 | |
Biofeedback | 85.2 ± 8.96 | 97.00 ± 11.90 | 11.80 ± 2.94 a | 0.003 a | |
tDCS | 84.01 ± 15.62 | 96.4 ± 15.39 | 12.40 ± 0.23 a | 0.037 a | |
Anterior- lateral | 0.7 | ||||
Control | 81.50 ± 11.68 | 84.30 ± 12.95 | 2.80 ± 1.27 a | 0.28 | |
Biofeedback | 73.60 ± 20.17 | 85.80 ± 9.84 | 12.20 ± 10.33 a | 0.05 a | |
tDCS | 82.70 ± 12.78 | 91.10 ± 19.38 | 8.40 ± 6.60 a | 0.30 | |
lateral | 0.49 | ||||
Control | 78.8 ± 12.73 | 85.60 ± 10.26 | 6.80 ± 2.47 a | 0.30 | |
Biofeedback | 80.10 ± 16.04 | 87.80 ± 11.14 | 7.70 ± 4.90 a | 0.04 a | |
tDCS | 82.00 ± 12.09 | 88.80 ± 18.40 | 6.80 ± 6.31 a | 0.009 a | |
Lateral-posterior | 0.48 | ||||
Control | 84.6 ± 8.72 | 87.80 ± 5.19 | 3.20 ± 3.53 a | 0.34 | |
Biofeedback | 81.10 ± 11.82 | 94.80 ± 15.6 | 13.70 ± 3.78 a | 0.02 a | |
tDCS | 85.07 ± 13.89 | 97.90 ± 16.22 | 12.83 ± 2.33 a | 0.05 a | |
Posterior | 0.6 | ||||
Control | 79.40 ± 8.94 | 85.10 ± 6.92 | 5.70 ± 2.02 a | 0.03 a | |
Biofeedback | 89.40 ± 11.72 | 97.50 ± 14.45 | 8.10 ± 2.73 a | 0.03 a | |
tDCS | 85.10 ± 15.32 | 95.70 ± 18.95 | 10.06 ± 3.60 a | 0.08 | |
Posterior-medial | 0.54 | ||||
Control | 80.97 ± 11.43 | 93.10 ± 7.70 | 12.13 ± 3.73 a | 0.04 a | |
Biofeedback | 82.01 ± 10.90 | 92.40 ± 17.11 | 10.39 ± 6.21 a | 0.05 a | |
tDCS | 83.86 ± 14.21 | 96.08 ± 20.58 | 12.22 ± 6.37 a | 0.005 a | |
Medial | 0.5 | ||||
Control | 67.90 ± 12.80 | 72.80 ± 12.69 | 4.90 ± 0.11 a | 0.04 a | |
Biofeedback | 68.60 ± 18.82 | 82.60 ± 17.64 | 14.00 ± 1.18 a | 0.05 a | |
tDCS | 72.01 ± 17.06 | 86.30 ± 21.95 | 14.29 ± 4.89 a | 0.005 a | |
Anterior- medial | 0.5 | ||||
Control | 55.20 ± 12.78 | 59.67 ± 15.25 | 4.47 ± 2.47 a | 0.054 | |
Biofeedback | 60.68 ± 23.56 | 72.50 ± 25.42 | 11.82 ± 1.86 a | 0.009 a | |
tDCS | 60.59 ± 17.19 | 72.10 ± 24.44 | 11.51 ± 7.25 a | 0.01 a |
Comparison of Proprioception in Three Different Knee Angel at 30, 45, and 90 Degrees in in Three Groups; Control, Biofeedback and tDCS Group Pre- and Post-intervention in People with ACL Rupture
Knee Angles and Groups | Pre-intervention | Post-intervention | Differences | P-Value | P-Value (Inter-group Comparison) |
---|---|---|---|---|---|
Knee absolute error (30 degrees) | 0.09 | ||||
Control | 23.20 ± 4.20 | 21.02 ± 2.97 | 2.22 ± 1.23 a | 0.03 b | |
Biofeedback | 17.02 ± 8.90 | 9.20 ± 4.10 | 7.82 ± 6.49 a | 0.003 b | |
tDCS | 17.10 ± 6.92 | 13.10 ± 5.09 | 4.00 ± 1.83 a | 0.001 b | |
Knee absolute error (45 degrees) | 0.21 | ||||
Control | 28.70 ± 12.40 | 23.50 ± 9.37 | 5.20 ± 3.03 a | 0.09 | |
Biofeedback | 23.80 ± 10.68 | 12.30 ± 5.75 | 11.41 ± 4.93 a | 0.001 b | |
tDCS | 21.60 ± 9.55 | 14.08 ± 5.76 | 7.52 ± 3.79 a | 0.001 b | |
Knee absolute error (90 degrees) | 0.68 | ||||
Control | 14.80 ± 3.32 | 7.20 ± 4.11 | 7.60 ± 0.79 a | 0.01 b | |
Biofeedback | 15.82 ± 8.96 | 8.30 ± 3.50 | 7.52 ± 5.46 a | 0.001 b | |
tDCS | 16.00 ± 6.39 | 14.40 ± 3.67 | 1.60 ± 2.72 a | 0.001 b |
4.2. Intra-group Comparison
The intra-group comparison showed that ten-session biofeedback modality used in individuals with ACL rupture significantly improved the functional balance on SEBT at all directions in injured limb compared with baseline condition (P < 0.05, Table 2). For tDCS group, the functional balance at six directions, including anterior, lateral, posterior-lateral, posterior-medial, medial, and posterior-medial was significantly improved (P < 0.05, Table 2). No significant difference was observed for anterior-lateral and posterior directions using tDCS. For the control group, a significant improvement was observed in posterior, posterior-medial, and medial directions following rehabilitation exercises without any electromyography biofeedback or tDCS, but not for other directions.
Furthermore, absolute error in knee reconstruction at three knee flexion angles (30, 45, and 90 degrees) of the injured knee joint was significantly decreased after interventions in both groups receiving biofeedback and tDCS compared to before interventions. For the control group, a significant reduction was observed at knee angles of 30 and 90 degrees (Table 3, P < 0.05), with no significant difference at an angle 45 degree (Table 3, P > 0.05).
5. Discussion
In this study, it was hypothesized that proprioception and balance may have affected by the intervention type. According to our results, however, no significant difference was seen among groups. The lack of differences between interventions may have been due to the short-term follow-up of using the biofeedback and tDCS modalities. Since all groups received contraction exercises at different training angles, a ten-session period of training may not have been enough to demonstrate a large difference among interventions. Therefore, it was speculated that longer follow-up using biofeedback or tDCS while exercising may have detected enough changes between interventions, and it was suggested that this possibility should be investigated by further studies.
Although no significant difference was found in the measured outcomes among the groups, the findings confirmed an improvement in proprioception as the knee absolute error at the knee flexion angles of 30, 45, and 90 degrees was reduced following the use of biofeedback or tDCS modalities while exercising. However, its improvement was not found for the angle of 45 degrees in group receiving intermittent isometric exercises alone. Our study results regarding the effect of biofeedback on improving the knee proprioception were in line with the findings from studies by Ghomashchi (37), Christanell et al. (28), and Carpinella et al. (38) investigating patients with stroke, ACL rupture, and Parkinson, respectively. The accuracy of angle reconstruction tested in assessing joint position sense of the knee depends on the amount of information sent from the skin, muscle, and joint receptors as proprioceptive sources. The proprioception increases the information sent from the knee receptors and enhances the accuracy of signals. This is because the received visual feedback can lead to learning precise movements by correcting the errors observed during the exercises, which is consistent with the analysis of Oh and Hwangbo’s study (2018) (39). They used visual biofeedback along with exercise training in patients with knee arthroscopy and suggested that information received through visual feedback could act as a sensory alternative to compensate for the proprioception of the injured limb in patients (39). Regarding the effect of tDCS, on the other hand, the previous studies have also demonstrated that it could have a positive role in the improvement of absolute error of proprioception in healthy young people (40-42) and patients with tendinitis (43). Stagg et al. indicated that applying tDCS over left dorsolateral prefrontal cortex (DLPFC) caused increased activity of the sensorimotor cortex and decreased activity of the thalamus. It was hypothesized that increased activity of the sensorimotor cortex might enhance proprioception (44). Therefore, the tDCS is another therapeutic method applied through electrodes on the scalp, and it is believed that cortical irritability is altered by changing the potential of the cell membrane due to the cathodal effect.
Proprioception is defined as the sense of awareness of joint position. It is a process in which the central nervous system receives information from the environments about the position and movement of different parts of body in conscious and unconscious levels. In addition to the visual and vestibular systems information, the proprioception provides information for planning and designing various movements to the central nervous system. Injuries to the ACL cause irreversible damages to knee proprioception. Therefore, a comprehensive rehabilitation program can improve the proprioception in order to create an environment for restoration and development of motor responses for involved limb. It seems that in people with ACL rupture, due to the presence of knee proprioception defect, performing simultaneous proprioception exercises with modalities such as biofeedback (affecting the peripheral nervous system) and tDCS (affecting the central nervous system) could exert an effective impact on improvement of proprioception. In addition, since the exercises at angles of 30, 45, and 90 were associated with repetitive movements during stimulation, it also had a retraining aspect for the participants.
According to the evidence (31), on the other hand, exercises in different angles of knee flexion with active and passive positions have been emphasized as therapeutic exercises. In the present study, we found a significant improvement of proprioception in all angles of 30, 45, and 90 degrees, with no preference of an angle on others. Thus, it was recommend that proprioception exercises should be performed at different flexion angles of knee.
According to our SEBT results, functional balance for both groups of biofeedback and tDCS in the most directions and for the control group in only three directions was significantly improved compared to before intervention in the participants with ACL rupture. During the SEBT, both hamstring and quadriceps muscle contraction, as muscle stabilizers during the control of torso movements, are necessary to maintain balance, as Ambegaonkar et al. indicated a significant relationship between the strength of hamstring and quadriceps muscles with better performance during this test (45). Another study by Yang et al. also focused on improving the activity of the rectus femoris and biceps femoris muscles following the use of tDCS (46).
The effect of TDCS on skill learning by applying the cerebellar program has been shown previous studies (47, 48). The cerebellum is a part of the motor skill learning network that plays a special role in the early stages of learning due to the connection between the posterior-lateral hemisphere of the cerebellum and the prefrontal region (49). Biofeedback also intensifies the information sent from the knee receptors and promotes proprioception along with visual feedback, which leads to learning precise movements by correcting the errors observed during exercise. In the present study, the biofeedback and tDCS, in addition to increasing the accuracy of sensory information (by affecting the nervous-central and peripheral system as well as strengthening the muscles around the knee according to training positions), may have played an important role in regulating movements using muscle control and improving functional balance in different directions. According to our study results and those from a recent systematic review, therefore, both biofeedback (17, 50) and tDCS (15, 16) were methods effective in dealing with outcomes related to balance in different populations.
This study faced few limitations. First limitation was its short-term follow-up. Also, the effect of gender difference on responding to tDCS or biofeedback was not determined since all participants were male athletes. Therefore, it was recommended that further studies should be conducted to investigate the cooper and non-cooper patients and compare these two groups together, examine the individuals after ACL reconstruction, and record the electrical activity of knee muscles.
5.1. Conclusions
Although the results did not show a large difference among groups, using both biofeedback and tDCS modalities along with intermittent isometric exercises may have reduced the value of absolute errors at 30, 45, and 90 degree of knee flexion angles as well as improved functional balance. Therefore, it was recommended that these modalities should be included in rehabilitation program targeting ACL-deficient knee subjects.
Acknowledgements
References
-
1.
Georgoulis AD, Ristanis S, Moraiti CO, Paschos N, Zampeli F, Xergia S, et al. ACL injury and reconstruction: Clinical related in vivo biomechanics. Revue de Chirurgie Orthopédique et Traumatologique. 2010;96(8):S339-48. https://doi.org/10.1016/j.rcot.2010.09.015.
-
2.
Miyasaka KC. The incidence of knee ligament injuries in the general population. Am J Knee Surg. 1991;1:43-8.
-
3.
Angoules AG, Mavrogenis AF, Dimitriou R, Karzis K, Drakoulakis E, Michos J, et al. Knee proprioception following ACL reconstruction; a prospective trial comparing hamstrings with bone-patellar tendon-bone autograft. Knee. 2011;18(2):76-82. [PubMed ID: 20149662]. https://doi.org/10.1016/j.knee.2010.01.009.
-
4.
Bonfim TR, Jansen Paccola CA, Barela JA. Proprioceptive and behavior impairments in individuals with anterior cruciate ligament reconstructed knees. Arch Phys Med Rehabil. 2003;84(8):1217-23. [PubMed ID: 12917863]. https://doi.org/10.1016/s0003-9993(03)00147-3.
-
5.
Vaugoyeau M, Viel S, Amblard B, Azulay JP, Assaiante C. Proprioceptive contribution of postural control as assessed from very slow oscillations of the support in healthy humans. Gait Posture. 2008;27(2):294-302. [PubMed ID: 17509884]. https://doi.org/10.1016/j.gaitpost.2007.04.003.
-
6.
Riemann BL, Lephart SM. The Sensorimotor System, Part II: The Role of Proprioception in Motor Control and Functional Joint Stability. J Athl Train. 2002;37(1):80-4. [PubMed ID: 16558671]. [PubMed Central ID: PMC164312].
-
7.
Lacour M, Bernard-Demanze L, Dumitrescu M. Posture control, aging, and attention resources: models and posture-analysis methods. Neurophysiol Clin. 2008;38(6):411-21. [PubMed ID: 19026961]. https://doi.org/10.1016/j.neucli.2008.09.005.
-
8.
Wang H, Ji Z, Jiang G, Liu W, Jiao X. Correlation among proprioception, muscle strength, and balance. J Phys Ther Sci. 2016;28(12):3468-72. [PubMed ID: 28174475]. [PubMed Central ID: PMC5276784]. https://doi.org/10.1589/jpts.28.3468.
-
9.
Bonfim TR, Grossi DB, Paccola CA, Barela JA. Additional sensory information reduces body sway of individuals with anterior cruciate ligament injury. Neurosci Lett. 2008;441(3):257-60. [PubMed ID: 18582536]. https://doi.org/10.1016/j.neulet.2008.06.039.
-
10.
Trulsson A, Roos EM, Ageberg E, Garwicz M. Relationships between postural orientation and self reported function, hop performance and muscle power in subjects with anterior cruciate ligament injury. BMC Musculoskelet Disord. 2010;11:143. [PubMed ID: 20594339]. [PubMed Central ID: PMC2908077]. https://doi.org/10.1186/1471-2474-11-143.
-
11.
McNair PJ, Wood GA, Marshall RN. Stiffness of the hamstring muscles and its relationship to function in anterior cruciate ligament deficient individuals. Clin Biomech (Bristol, Avon). 1992;7(3):131-7. [PubMed ID: 23915720]. https://doi.org/10.1016/0268-0033(92)90027-2.
-
12.
Blasimann A, Koenig I, Baert I, Baur H, Vissers D. Which assessments are used to analyze neuromuscular control by electromyography after an anterior cruciate ligament injury to determine readiness to return to sports? A systematic review. BMC Sports Sci Med Rehabil. 2021;13(1):142. [PubMed ID: 34749813]. [PubMed Central ID: PMC8577028]. https://doi.org/10.1186/s13102-021-00370-5.
-
13.
Balachandar V, Marciniak JL, Wall O, Balachandar C. Effects of the menstrual cycle on lower-limb biomechanics, neuromuscular control, and anterior cruciate ligament injury risk: a systematic review. Muscles Ligaments Tendons J. 2017;7(1):136-46. [PubMed ID: 28717621]. [PubMed Central ID: PMC5505581]. https://doi.org/10.11138/mltj/2017.7.1.136.
-
14.
Ma J, Zhang D, Zhao T, Liu X, Wang J, Zheng H, et al. The effects of proprioceptive training on anterior cruciate ligament reconstruction rehabilitation: A systematic review and meta-analysis. Clin Rehabil. 2021;35(4):506-21. [PubMed ID: 33222527]. https://doi.org/10.1177/0269215520970737.
-
15.
Baharlouei H, Saba MA, Shaterzadeh Yazdi MJ, Jaberzadeh S. The effect of transcranial direct current stimulation on balance in healthy young and older adults: A systematic review of the literature. Neurophysiol Clin. 2020;50(2):119-31. [PubMed ID: 32113708]. https://doi.org/10.1016/j.neucli.2020.01.006.
-
16.
Navarro-López V, Molina-Rueda F, Jiménez-Jiménez S, Alguacil-Diego IM, Carratalá-Tejada M. Effects of Transcranial Direct Current Stimulation Combined with Physiotherapy on Gait Pattern, Balance, and Functionality in Stroke Patients. A Systematic Review. Diagnostics (Basel). 2021;11(4). [PubMed ID: 33916442]. [PubMed Central ID: PMC8066876]. https://doi.org/10.3390/diagnostics11040656.
-
17.
Bowman T, Gervasoni E, Arienti C, Lazzarini SG, Negrini S, Crea S, et al. Wearable Devices for Biofeedback Rehabilitation: A Systematic Review and Meta-Analysis to Design Application Rules and Estimate the Effectiveness on Balance and Gait Outcomes in Neurological Diseases. Sensors (Basel). 2021;21(10). [PubMed ID: 34063355]. [PubMed Central ID: PMC8156914]. https://doi.org/10.3390/s21103444.
-
18.
Afzal MR, Oh MK, Choi HY, Yoon J. A novel balance training system using multimodal biofeedback. Biomed Eng Online. 2016;15:42. [PubMed ID: 27103536]. [PubMed Central ID: PMC4840978]. https://doi.org/10.1186/s12938-016-0160-7.
-
19.
Duarte-Moreira RJ, Castro KV, Luz-Santos C, Martins JVP, Sá KN, Baptista AF. Electromyographic Biofeedback in Motor Function Recovery After Peripheral Nerve Injury: An Integrative Review of the Literature. Appl Psychophysiol Biofeedback. 2018;43(4):247-57. [PubMed ID: 30168003]. https://doi.org/10.1007/s10484-018-9403-7.
-
20.
Karaborklu Argut S, Celik D, Yasacı Z. Effectiveness of therapeutic electromyographic biofeedback after orthopedic knee surgeries: a systematic review. Disabil Rehabil. 2022;44(14):3364-72. [PubMed ID: 33417500]. https://doi.org/10.1080/09638288.2020.1867904.
-
21.
OĞUl A, Ercan S, ÇEtİN C, Canbulut A, Ergan M, Acar HT, et al. The effect of biofeedback exercises for the quadriceps muscle on the muscle strength, balance, and proprioception. Med dello Sport. 2022;75(1). https://doi.org/10.23736/s0025-7826.22.03894-7.
-
22.
Kiefer AW, Kushner AM, Groene J, Williams C, Riley MA, Myer GD. A Commentary on Real-Time Biofeedback to Augment Neuromuscular Training for ACL Injury Prevention in Adolescent Athletes. J Sports Sci Med. 2015;14(1):1-8. [PubMed ID: 25729282]. [PubMed Central ID: PMC4306760].
-
23.
Kuo MF, Paulus W, Nitsche MA. Therapeutic effects of non-invasive brain stimulation with direct currents (tDCS) in neuropsychiatric diseases. Neuroimage. 2014;85 Pt 3:948-60. [PubMed ID: 23747962]. https://doi.org/10.1016/j.neuroimage.2013.05.117.
-
24.
Jwa A. Early adopters of the magical thinking cap: a study on do-it-yourself (DIY) transcranial direct current stimulation (tDCS) user community. J Law Biosci. 2015;2(2):292-335. [PubMed ID: 27774197]. [PubMed Central ID: PMC5034380]. https://doi.org/10.1093/jlb/lsv017.
-
25.
Lee Y, Yang H, Jeong C, Yoo Y, Jeong S, Jeon O, et al. The Effects of Transcranial Direct Current Stimulation on Functional Movement Performance and Balance of the Lower Extremities. J Phys Ther Sci. 2012;24(12):1215-8. https://doi.org/10.1589/jpts.24.1215.
-
26.
Valeriani M, Restuccia D, Di Lazzaro V, Franceschi F, Fabbriciani C, Tonali P. Central nervous system modifications in patients with lesion of the anterior cruciate ligament of the knee. Brain. 1996;119 ( Pt 5):1751-62. [PubMed ID: 8931595]. https://doi.org/10.1093/brain/119.5.1751.
-
27.
Molka AZ, Lisiński P, Huber J. Visual biofeedback exercises for improving body balance control after anterior cruciate ligament reconstruction. J Phys Ther Sci. 2015;27(7):2357-60. [PubMed ID: 26311983]. [PubMed Central ID: PMC4540881]. https://doi.org/10.1589/jpts.27.2357.
-
28.
Christanell F, Hoser C, Huber R, Fink C, Luomajoki H. The influence of electromyographic biofeedback therapy on knee extension following anterior cruciate ligament reconstruction: a randomized controlled trial. Sports Med Arthrosc Rehabil Ther Technol. 2012;4(1):41. [PubMed ID: 23126601]. [PubMed Central ID: PMC3582470]. https://doi.org/10.1186/1758-2555-4-41.
-
29.
Hamdolah HADI, Farhadi H, Bashiri M. Effect of six week strength and plyometric training on dynamic balance of male athletic student. Res Rehabil Sci. 2011;7(2):215-24.
-
30.
Gribble PA, Hertel J, Plisky P. Using the Star Excursion Balance Test to assess dynamic postural-control deficits and outcomes in lower extremity injury: a literature and systematic review. J Athl Train. 2012;47(3):339-57. [PubMed ID: 22892416]. [PubMed Central ID: PMC3392165]. https://doi.org/10.4085/1062-6050-47.3.08.
-
31.
Noyes FR. Noyes' Knee Disorders: Surgery, Rehabilitation, Clinical Outcomes E-Book. Amsterdam: Elsevier Health Sciences; 2016.
-
32.
Faria P, Leal A, Miranda PC. Comparing different electrode configurations using the 10-10 international system in tDCS: a finite element model analysis. Annu Int Conf IEEE Eng Med Biol Soc. 2009;2009:1596-9. [PubMed ID: 19964541]. https://doi.org/10.1109/iembs.2009.5334121.
-
33.
Kaminski E, Steele CJ, Hoff M, Gundlach C, Rjosk V, Sehm B, et al. Transcranial direct current stimulation (tDCS) over primary motor cortex leg area promotes dynamic balance task performance. Clin Neurophysiol. 2016;127(6):2455-62. [PubMed ID: 27178865]. https://doi.org/10.1016/j.clinph.2016.03.018.
-
34.
Duarte Nde A, Grecco LA, Galli M, Fregni F, Oliveira CS. Effect of transcranial direct-current stimulation combined with treadmill training on balance and functional performance in children with cerebral palsy: a double-blind randomized controlled trial. PLoS One. 2014;9(8). e105777. [PubMed ID: 25171216]. [PubMed Central ID: PMC4149519]. https://doi.org/10.1371/journal.pone.0105777.
-
35.
Kaski D, Dominguez RO, Allum JH, Bronstein AM. Improving gait and balance in patients with leukoaraiosis using transcranial direct current stimulation and physical training: an exploratory study. Neurorehabil Neural Repair. 2013;27(9):864-71. [PubMed ID: 23897903]. https://doi.org/10.1177/1545968313496328.
-
36.
Akkaya N, Ardic F, Ozgen M, Akkaya S, Sahin F, Kilic A. Efficacy of electromyographic biofeedback and electrical stimulation following arthroscopic partial meniscectomy: a randomized controlled trial. Clin Rehabil. 2012;26(3):224-36. [PubMed ID: 21971752]. https://doi.org/10.1177/0269215511419382.
-
37.
Ghomashchi H. Investigating the effects of visual biofeedback therapy on recovery of postural balance in stroke patients using a complexity measure. Top Stroke Rehabil. 2016;23(3):178-83. [PubMed ID: 27077976]. https://doi.org/10.1080/10749357.2015.1122311.
-
38.
Carpinella I, Cattaneo D, Bonora G, Bowman T, Martina L, Montesano A, et al. Wearable Sensor-Based Biofeedback Training for Balance and Gait in Parkinson Disease: A Pilot Randomized Controlled Trial. Arch Phys Med Rehabil. 2017;98(4):622-630000. [PubMed ID: 27965005]. https://doi.org/10.1016/j.apmr.2016.11.003.
-
39.
Oh HT, Hwangbo G. The effects of proprioception exercise with and without visual feedback on the pain and balance in patients after total knee arthroplasty. J Phys Ther Sci. 2018;30(1):124-6. [PubMed ID: 29410581]. [PubMed Central ID: PMC5788790]. https://doi.org/10.1589/jpts.30.124.
-
40.
Kamii Y, Kojima S, Onishi H. Transcranial direct current stimulation over the posterior parietal cortex improves visuomotor performance and proprioception in the lower extremities. Front Hum Neurosci. 2022;16:876083. [PubMed ID: 36061503]. [PubMed Central ID: PMC9434688]. https://doi.org/10.3389/fnhum.2022.876083.
-
41.
Jamebozorgi AA, Sadeghi H, Rahimi A, Kazemi SM, Matinhomaei H. The effect of a Biofeedback Training Course and Transcranial Direct Current Stimulation (tDCS) on Balance, Proprioception and Performance. J Clin Physiother Res. 2020;5(1). e5.
-
42.
Beck EN, Narayanan SS, McDermott R, Witney AG. Can transcranial direct current stimulation over the dorsolateral prefrontal cortex enhance proprioception? bioRxiv. 2019;Preprint. https://doi.org/10.1101/539510.
-
43.
Arzani P, Khalkhalizavieh M, Kalantari K, Baghban A. The Effect of Transcranial Direct Current Stimulation (tDCS) Modality on Proprioception in Patients with Tendinitis: A Review. J Complement Med Res. 2021;12(2):101. https://doi.org/10.5455/jcmr.2021.12.02.14.
-
44.
Stagg CJ, Lin RL, Mezue M, Segerdahl A, Kong Y, Xie J, et al. Widespread modulation of cerebral perfusion induced during and after transcranial direct current stimulation applied to the left dorsolateral prefrontal cortex. J Neurosci. 2013;33(28):11425-31. [PubMed ID: 23843514]. [PubMed Central ID: PMC3724554]. https://doi.org/10.1523/jneurosci.3887-12.2013.
-
45.
Ambegaonkar JP, Mettinger LM, Caswell SV, Burtt A, Cortes N. Relationships between core endurance, hip strength, and balance in collegiate female athletes. Int J Sports Phys Ther. 2014;9(5):604-16. [PubMed ID: 25328823]. [PubMed Central ID: PMC4196325].
-
46.
Yang DJ, Park SK, Uhm YH. Influence of Transcranial Direct Current Stimulation on Lower Limb Muscle Activation and Balance Ability in Soccer Player. J Korean Phys Ther. 2018;30(6):211-7. https://doi.org/10.18857/jkpt.2018.30.6.211.
-
47.
Kumari N, Taylor D, Signal N. The Effect of Cerebellar Transcranial Direct Current Stimulation on Motor Learning: A Systematic Review of Randomized Controlled Trials. Front Hum Neurosci. 2019;13:328. [PubMed ID: 31636552]. [PubMed Central ID: PMC6788395]. https://doi.org/10.3389/fnhum.2019.00328.
-
48.
Ferrucci R, Brunoni AR, Parazzini M, Vergari M, Rossi E, Fumagalli M, et al. Modulating human procedural learning by cerebellar transcranial direct current stimulation. Cerebellum. 2013;12(4):485-92. [PubMed ID: 23328908]. https://doi.org/10.1007/s12311-012-0436-9.
-
49.
Doyon J, Penhune V, Ungerleider LG. Distinct contribution of the cortico-striatal and cortico-cerebellar systems to motor skill learning. Neuropsychologia. 2003;41(3):252-62. [PubMed ID: 12457751]. https://doi.org/10.1016/s0028-3932(02)00158-6.
-
50.
Alhasan H, Hood V, Mainwaring F. The effect of visual biofeedback on balance in elderly population: a systematic review. Clin Interv Aging. 2017;12:487-97. [PubMed ID: 28293105]. [PubMed Central ID: PMC5345985]. https://doi.org/10.2147/cia.s127023.