Abstract
Background:
Chronic lymphocytic leukemia (CLL) primarily affects the elderly, with its etiology largely unknown. It is hypothesized that hematopoietic stem cells may acquire mutations over time, such as the BCL-2 mutation, leading to disruptions in the apoptotic process. Dangui Luhui Wan, a mixture of 11 herbs used in Chinese Medicine, has shown antitumor activities across various cancer cell types. Indirubin-3'-monoxime (I3M), derived from Dangui Luhui Wan, functions as a selective inhibitor of cyclin-dependent kinases (CDKs) and can induce apoptosis in cells.Objectives:
The objective of this study was to evaluate the effectiveness of I3M against CLL cells in vitro.Methods:
Peripheral blood mononuclear cells (PBMCs) from 14 patients were treated with I3M at concentrations ranging from 0.1 μM to 80 μM over periods of 24, 48, and 72 hours. The optimal dose was determined using Annexin V and MTT assays. The expression of apoptotic genes Bcl-2/Bax and CDK1/2 was assessed using real-time PCR.Results:
The results indicated that a 20 µM concentration of I3M exhibited the highest cytotoxicity after 48 hours compared to controls (P = 0.005). Post-treatment, a decrease in Bcl-2 gene expression was observed, while changes in the Bax gene were not significant. However, an increase in the Bax/Bcl-2 gene ratio was noted, suggesting involvement of the mitochondrial pathway in I3M's apoptotic mechanism. Notably, I3M inhibited the expression of the CDK2 gene but did not affect CDK1 gene expression.Conclusions:
I3M appears to exert anti-tumor effects by inducing apoptosis and inhibiting the CDK2 gene. Further research is required to elucidate the precise mechanism of action of I3M in CLL and potentially other tumor cell lines.Keywords
Chronic lymphocytic leukemia CLL Indirubin Bcl-2 Bax CDK1 CDK2
1. Background
B-Chronic lymphocytic leukemia (B-CLL) represents 25 - 30% of all leukemias in Western countries (1). Men are more commonly affected than women, with a male-to-female ratio of 1.7: 1 (2). The disease typically presents with significant lymphocytosis in peripheral blood, and involvement of the bone marrow is almost invariably present (3). While a bone marrow biopsy is not essential for diagnosing CLL, it can be helpful for assessing treatment response or during follow-up.
The pathogenesis of CLL is still under debate, but much research has focused on the aberrant regulation of apoptotic mediators, including members of the B-cell lymphoma 2 (Bcl-2) family. This family comprises anti-apoptotic proteins such as Bcl-2, Bcl-xl, Bcl-w, and Mcl-1, and pro-apoptotic proteins, such as Bax, Bak, and Bok, often altered by genetic mutations (4). Notably, overexpression of Bcl-2 increases the Bcl-2/Bax ratio, inhibiting caspase-dependent apoptosis in CLL cells and reducing chemotherapy efficacy (5).
Recent advancements have been made in therapies targeting these mechanisms (6). Alterations in cell cycle regulatory genes may contribute to the clonal expansion of malignant B cells. Cyclin-dependent kinases (CDKs) are critical regulators of the cell cycle, transcription, and apoptosis and represent potential targets for treating hematologic malignancies (7, 8). Disruption of CDKs or their associated cyclins is frequently linked to tumorigenesis (9-11).
The B cell receptor (BCR), a transmembrane protein on B cells, consists of a membrane-bound immunoglobulin molecule and a signal transduction moiety (12). Translocations and overexpressions of cyclin D1 and cyclin D2 have been observed in CLL (13). Cyclin D2 plays a crucial role in the proliferation of CD5 B cells and mediates its effects through the BCR, which regulates progression into the G1 phase of the cell cycle in B lymphocytes (14, 15).
Targeting CDK1 could be beneficial for treating hematologic malignancies due to its role in phosphorylating the retinoblastoma protein (pRb) when paired with cyclin D. Phosphorylating the retinoblastoma protein regulates cell cycle progression and gene expression through its activities and interactions with cellular components, playing a pivotal role in cell proliferation and differentiation (16). Cyclin-dependent kinases 1 is engaged in managing the cell cycle during the G2 and M phases and is essential for processes such as DNA damage repair, mesenchymal stem cell (MSC) differentiation, and epigenetic gene silencing (16, 17). Although both CDK1 and CDK2 suppress cancer cell invasion, studies have linked CDK1 with disease progression in conditions like acute myeloid leukemia (AML) and chronic myelomonocytic leukemia (CML) (18, 19). Elevated levels of CDK1 have been detected in breast cancer, gliomas, and colon cancer, with increased CDK2 expression noted in breast cancer (13). Various CDK inhibitors, including CDKI-73© (a non-selective CDK9 inhibitor), Flavopiridol© (a pan-CDK inhibitor targeting CDKs 1, 2, 4, 6, 7, and 9), Dinaciclib© (which inhibits CDKs 1, 2, 5, and 9), and other pan-CDK inhibitors have shown anti-proliferative and pro-apoptotic effects (20). Furthermore, CDK inhibitors trigger apoptosis by damaging mitochondria in human leukemia cells, suggesting that CDK1 modulation might be a novel therapeutic avenue for CLL. However, CDK inhibitors can cause severe side effects, such as tumor lysis syndrome and secretory diarrhea, and their clinical use should be managed by experienced hematologists (21).
In addition to conventional and emerging therapies for CLL, herbal medicine is also worth exploring. Dangui Luhui Wan, composed of 11 herbs, is a widely used remedy in Chinese Medicine. Indirubin-3-monoxime (I3M), an active component of Dangui Luhui Wan, is derived from plants such as Baphicacanthus cusia,Indigofera suffruticosa, and Polygonum tinctorium (22). I3M has shown anticancer effects in several solid cancers and hematologic malignancies, including human glioma (23), ovarian cancer (24), and acute lymphoblastic leukemia (ALL) [25]. The anticancer mechanisms of I3M are believed to involve its inhibitory action on CDKs, competing with ATP binding at the catalytic site, thus inducing apoptosis and autophagy, and inhibiting STAT3 signaling (24, 25). To our knowledge, no studies have yet explored the use of I3M in CLL.
2. Objectives
This study aimed to evaluate the effects of I3M on cell survival, including the expression levels of CDK1/CDK2 and Bcl-2/Bax, as well as apoptosis and proliferation in CLL cells.
3. Methods
3.1. Subjects
Fourteen newly diagnosed CLL patients, with an average age of 60 ± 4 years, were selected based on the iwCLL criteria (26). An age and gender-matched control group of eight individuals was also included. The main demographic characteristics of the participants are summarized in Table 1. This study received ethical approval from the Ethical Committee of Semnan University of Medical Sciences (241; 1395.01.15). All participants provided informed consent prior to sampling.
Demographic and Laboratory Characteristics of Patients and Healthy Subjects
Patients (CLL) | 1 | 2 | 3 | 4 | 5 | 6 | 7 | 8 | 9 | 11 | 12 | 13 | 14 | Healthy Subjects (Ranges) |
---|---|---|---|---|---|---|---|---|---|---|---|---|---|---|
Age | 61 | 64 | 60 | 61 | 63 | 62 | 64 | 60 | 62 | 64 | 61 | 63 | 64 | 60 - 66 |
Gender | M | M | M | F | M | M | F | F | F | M | M | M | M | NA |
RAI stage | 0 | 0 | 0 | 0 | 0 | 0 | 0 | 0 | 0 | 0 | 0 | 0 | 0 | NA |
Binet stage | A | A | A | A | A | A | A | A | A | A | A | A | A | NA |
WBC/µL | 32000 | 30000 | 40000 | 35000 | 28000 | 50000 | 35000 | 42000 | 25000 | 60000 | 25000 | 40000 | 36000 | 5000 - 7000 |
Lymphocytes/µL | 14320 | 20350 | 15320 | 11500 | 8050 | 6250 | 3150 | 3140 | 47100 | 22360 | 7350 | 8600 | 45450 | 450 - 9640 |
Haemoglobin, g/dL | 14.3 | 12.2 | 16.6 | 15.1 | 14.5 | 12.4 | 14.8 | 15.4 | 16.3 | 15.2 | 14.3 | 16.3 | 14.3 | 12.2 - 14.3 |
Platelets, 103/ µL | 236 | 250 | 210 | 350 | 365 | 400 | 250 | 241 | 230 | 340 | 360 | 380 | 410 | 210 - 430 |
Beta 2 microglobulin, mg/dL | 2.0 | 2.5 | 2.8 | 2.3 | 3.2 | 2.9 | 2.1 | 2.4 | 2.7 | 3.7 | 4.1 | 3.6 | 4.2 | < 2.5 |
3.2. Peripheral Blood Mononuclear Cell Isolation and Cell Culture
Peripheral Blood Mononuclear Cell (PBMC) from CLL patients and healthy donors were isolated using a Ficoll-Hypaque density gradient (Sigma©, USA) from fresh lithium-heparinized blood samples. Briefly, 10 mL of PBS-diluted peripheral blood was layered over 5 mL of Ficoll-Hypaque and centrifuged for 20 minutes at 500 G. The PBMCs were collected from the interlayer, washed twice with PBS, and then cultured in three groups: (1) Treated with indirubin, (2) untreated (control group), and (3) treated with 1 µM fludarabine (positive control).
All groups were cultured in RPMI-1640 medium (Gibco™, USA) enriched with 10% fetal bovine serum (FBS), 2 mM L-glutamine, 100 IU/mL penicillin G, and 100 mg/mL streptomycin (Sigma, USA). The cultures were maintained at 37°C in a humidified incubator with 5% CO2 for 24, 48, and 72 hours.
3.3. Cell Viability Assay
To identify the optimal concentration of I3M that maximizes anti-tumor effects and minimizes cytotoxicity on normal cells, an MTT assay was employed. For this purpose, 105 PBMCs per well were plated in 96-well plates and treated with varying I3M concentrations (0.1, 0.25, 0.5, 1, 5, 10, 20, 40, and 80 μM) for 24, 48, and 72 hours. Afterward, 10 μL/well of MTT solution (0.5 mg/mL) was added, and the cells were incubated for 4 hours at 37°C. Subsequently, 100 µL/well of MTT solvent (10% Sodium dodecyl sulfate-SDS) was introduced, and the optical density at 570 nm was measured using a Stat Fax 2100 Microplate reader. Results were benchmarked against untreated control cells, with fludarabine-treated cells serving as a positive control. Data are presented as mean ± SD from at least three independent experiments. Furthermore, an apoptosis test was conducted on PBMCs and CD19+ cells (B cells) using various I3M concentrations at 24, 48, and 72 hours. Based on these results, an I3M concentration of 20 μM demonstrated the highest cytotoxic antitumoral effect with minimal impact on normal cells. An Annexin V assay further validated the optimal dosage (20 μM). This concentration was subsequently applied to other cells from patients and healthy donors for additional assays such as MTT and Annexin V at 48 hours. The IC50 was determined using dose-response curves to evaluate cell proliferation across different I3M concentrations.
3.4. Apoptosis Determination Assay
Apoptosis induced by I3M was assessed using flow cytometry. Briefly, 3 × 105 PBMCs per well from CLL patients and healthy subjects were cultured in 48-well plates and treated with 20 μM of I3M, then incubated for 48 hours at 37°C in 5% CO2. The Annexin V/7-AAD kit (Biolegend©, USA) was employed following the manufacturer's instructions to analyze apoptosis in PBMCs and CD19+ cells (B cells, anti-CD19-PE purchased from Biolegend). Results were quantified using a Partec cyflow© instrument (Partec©) and analyzed with FlowJo© software (Tree Star Inc©).
3.5. RNA Extraction, cDNA Synthesis, and qRT-PCR
To assess the impact of I3M on the expression levels of Bcl-2, Bax, CDK1, and CDK2 in CLL cells, 3.5 × 106 PBMCs/well were cultured with 20 µM I3M in 12-well plates for 48 hours. Total RNA was extracted using Trizol reagent (Thermo Fisher Scientific©) following the manufacturer's instructions. The quality and quantity of RNA were evaluated through gel electrophoresis and a NanoDrop instrument (Thermo Fisher Scientific©, USA), respectively. cDNA was synthesized from 1 μg of total RNA using a High-Capacity cDNA Reverse Transcription Kit. Real-time qRT-PCR was performed using SYBR Green Supermix (Amplicon©, USA). The primers used are listed in Table 2. Gene expression of Bcl-2, Bax, CDK1, and CDK2 was normalized to RPLP0 as the housekeeping internal control. Experiments were conducted using an ABI 7900 HT©, and gene expression levels were calculated using the 2−ΔΔCt method.
Primer Sequences
Genes | Primer Sequences |
---|---|
Bcl-2 | Sense: 5’-TCG GTGGGGTCATGTGTGTGG-3 |
Anti-sense: 5’-CACAAAGGCATCCCAGCCTCC-3’ | |
BAX | Sense: 5’-CTTTGTGGCCCTCAAGAGTT-3’ |
Anti-sense: 5’-AGAGGCTTTCGACGAAACATC-3’ | |
CDK1 | Sense: 5’-CTTTGTGGCCCTCAAGAGTT-3’ |
Anti-sense: 5’-AGAGGCTTTCGACGAAACATC-3’ | |
CDK2 | Sense: 5’-AATCCGCCTGGACACTGAGAC-3’ |
Anti-sense: 5’-AATCCGCCTGGACACTGAGAC-3’ |
3.6. Statistical Analysis
Statistical analyses were performed using Prism 7.0.3 software (GraphPad Software©). Normality was assessed before analysis. The Mann–Whitney U test was used for nonparametric comparisons, and the independent t-test was used for parametric data. Results are expressed as the mean ± standard deviation, with a P-value < 0.05 considered statistically significant.
4. Results
4.1. Determination of Optimal I3M Concentration
The cytotoxic effects of I3M were evaluated using MTT and Annexin V assays on CLL patients and healthy donors at concentrations ranging from 0.1 to 80 µM over 24, 48, and 72 hours. The optimal concentration was determined based on the highest cytotoxicity and apoptosis with the minimal side effects, identified as 20 µM (Figure 1A, B, and C). Fludarabine served as a positive control. PBMCs from CLL patients were exposed to varying concentrations of I3M for 48 hours to determine the IC50, which was found to be 8.6 µM, demonstrating significant inhibitory effects on cell proliferation (Figure 2).
Assessment of CLL patients' PBMC cytotoxicity over 24, 48, and 72 hours using serial dilutions of indirubin. MTT method, n = 14, CTRL: Control, Flu: Fludarabine 1 μM, I3M: Indirubin-3-monoxime ranging from 100 nm to 80 µM, * P = 0.05, ** P = 0.01, *** P = 0.0002, 0.0008, **** P = 0.0002, 0.0001
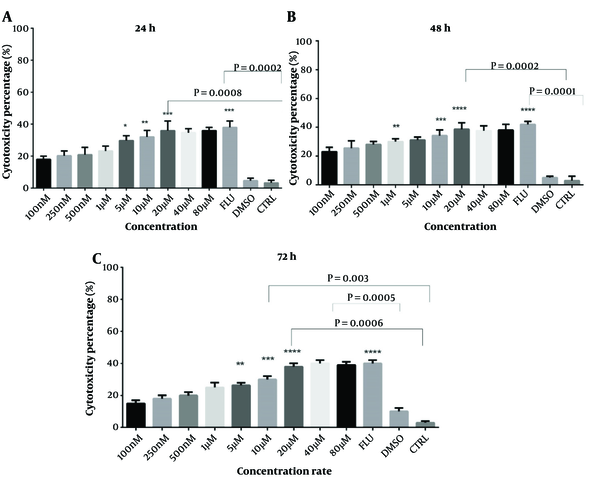
I3M’s inhibitory effects on cancer cell proliferation. CLL patients' PBMCs were treated with various I3M concentrations for 48 hours to determine the IC50
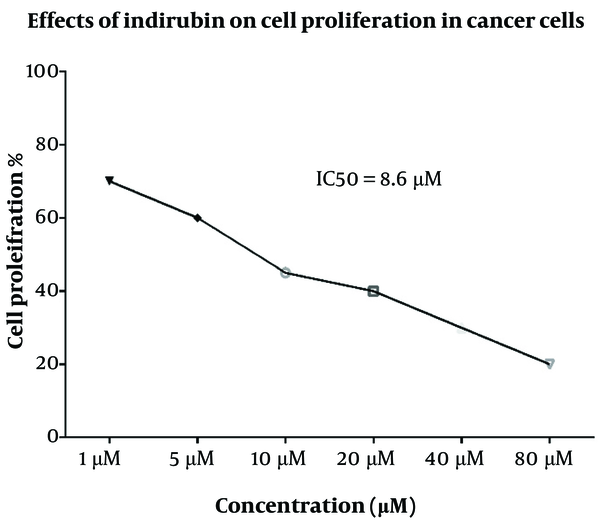
4.2. Cytotoxic Effect of I3M on CLL Cells but Not on Healthy Control Cells
The results showed that I3M at a 20 µM concentration exhibited the highest cytotoxicity after a 48-hour incubation compared to controls (P = 0.005). Fludarabine, used as a positive control, showed statistically significant cytotoxicity compared to controls (P = 0.0003). As illustrated in Figure 3B, there was a statistically significant increase in cell cytotoxicity in CLL cells (n = 14) following a 48-hour incubation with 20 µM I3M (P = 0.0001). The assessment of the anti-proliferative/cytotoxic impact of 20 µM I3M on PBMCs from CLL patients, compared with untreated cells, demonstrated a statistically significant decrease in cell viability (P = 0.0001) (Figure 3A). However, there was no statistically significant difference in cell viability between the I3M-treated group and the fludarabine-treated group (P > 0.5, Figure 3A). Conversely, in PBMCs from healthy controls, no significant anti-proliferative/cytotoxic effect was observed upon treatment with 20 µM I3M compared with untreated cells (P > 0.5).
Investigating the cytotoxicity of PBMC cells of CLL patients for 48 hours. MTT method, n = 14, CTRL: Control, DMSO: Carrier, Flu: Fludarabine 1 μM: I3M: Indirubin-3-monoxime; 20 μM **** P = 0.0001
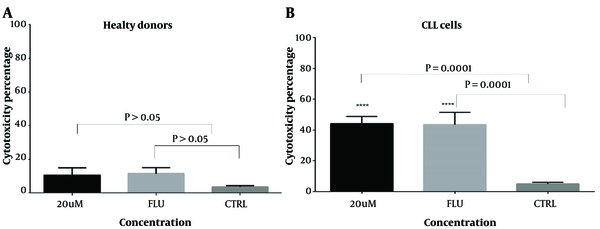
4.3. Apoptotic Effect of I3M on PBMCs and CD19+ CLL Cells
The apoptotic effects of I3M were evaluated in PBMCs and CD19+ CLL cells from both CLL patients and healthy controls. Cells were treated with various concentrations of I3M (0.1, 0.25, 0.5, 1, 5, 10, 20, 40, and 80 µM) over incubation periods of 24, 48, and 72 hours and then analyzed by flow cytometry. The most significant apoptotic effect of I3M was observed at a concentration of 20 µM after 48 hours of incubation (P = 0.0008; Figure 4A, B, and C, with flow cytometry images in Figure 5). As shown in Figure 6A, I3M (20 µM) induced apoptosis in PBMCs of CLL patients, similarly to the apoptotic effect of fludarabine (P = 0.0001). However, I3M did not induce significant apoptosis in the growth of cells treated with fludarabine or in those from healthy controls (P > 0.5, Figure 6B).
Evaluation of apoptosis in CLL patients' PBMCs over 24, 48, and 72 hours using serial dilutions of indirubin. Annexin V method, n = 14, CTRL: Control, DMSO: Carrier, Flu: Fludarabine 1 μM, I3M: Indirubin-3-monoxime ranging from 100 nM to 80 μM, ** P = 0.01, *** p = 0.005, **** p = 0.0001
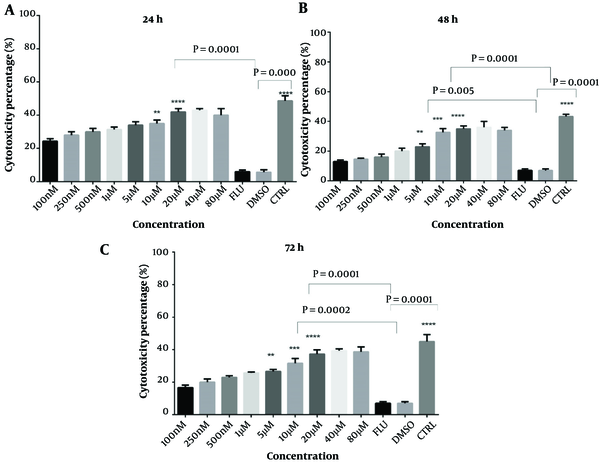
RN1 regions indicate cells stained with Annexin V dye, representing populations undergoing early apoptosis; RN3 regions show cells stained with 7AAD dyes, indicative of late-stage apoptosis. RN2 highlights cells stained with PE dye-linked anti-CD19+ antibodies, representing B cell populations
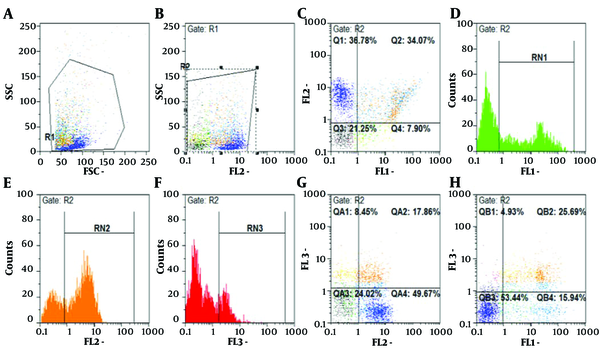
Evaluation of Apoptosis in CLL Patients' PBMCs over 48 hours using the Annexin V method, n = 14, CTRL: Control, Flu: Fludarabine 1 μM, I3M: Indirubin-3-monoxime 20 μM ***** P = 0.0001.
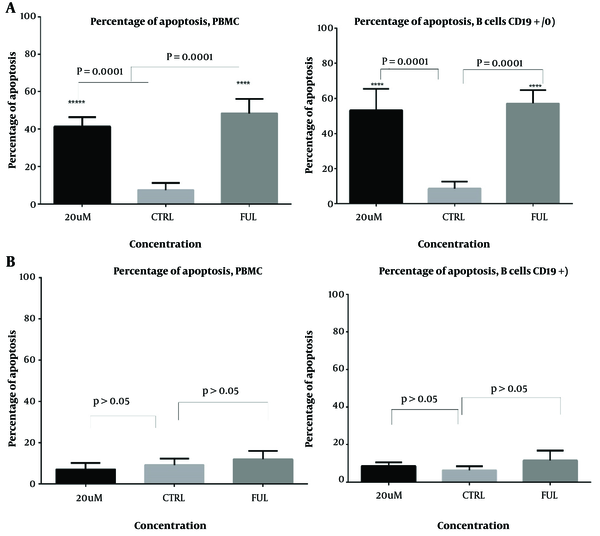
4.4. Apoptotic Impact of I3M on Bcl-2 Expression
To investigate the mechanism of apoptosis induced by I3M in CLL, we analyzed the expression levels of Bcl-2 and Bax in I3M-treated (20 µM) PBMCs from CLL patients over 48 hours compared to untreated cells. As illustrated in Figure 7A, I3M significantly reduced the expression of Bcl-2 in PBMCs from CLL patients (P = 0.03). However, there was no significant difference in Bax expression between I3M-treated and untreated cells (P > 0.5). Figure 7B shows that there was no statistically significant difference in the expression levels of Bcl-2 and Bax between I3M-treated and untreated PBMCs of healthy controls (P > 0.5 for both).
The apoptotic impact of I3M in CLL through Bcl-2 suppression; * P = 0.03.
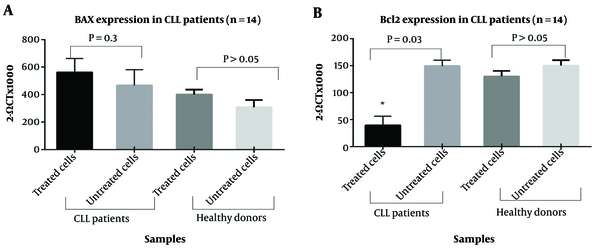
4.5. Inhibition of CDK2 Expression by I3M Treatment in CLL
To assess whether I3M affects cell cycle events, we measured the expression levels of CDK1 and CDK2 in PBMCs from CLL patients and healthy subjects treated with I3M (20 µM) for 48 hours, compared to untreated cells. As shown in Figure 8A, there was no statistically significant difference in CDK1 expression between I3M-treated and untreated cells, both in CLL patients and healthy controls. Conversely, CDK2 expression significantly decreased in I3M-treated cells compared to untreated cells (P = 0.03), as depicted in Figure 8B. However, no significant difference was observed in the expression levels of CDK2 in PBMCs of healthy controls compared to untreated cells (P > 0.5).
Inhibition of cyclin-dependent kinases (CDK2) expression by I3M treatment in CLL; * P = 0.03.
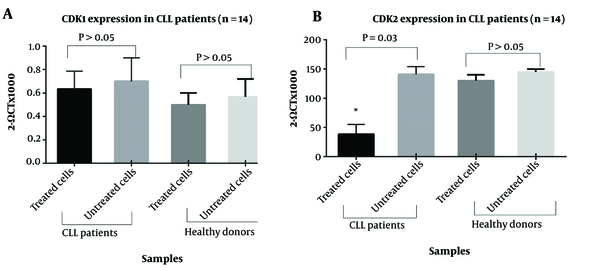
5. Discussion
With advancements in pharmaceutical technology, the exploration of bioactive compounds from herbal medicine offers a new avenue for cancer therapy. These compounds are recognized for their therapeutic potentials, yet scientific validation is often limited. Indirubin-3ʹ-monoxime (I3M) has been identified as a potent inducer of apoptotic cell death in various human cancer cells, including breast cancer, acute lymphoblastic leukemia, chronic myelomonocytic leukemia, osteosarcoma, and neuroblastoma, while exhibiting minimal cytotoxicity towards normal cells (27-29). The precise mechanisms by which I3M induces apoptotic cell death remain to be fully understood. This study provides compelling evidence that I3M induces apoptosis by modulating anti-apoptotic Bcl-2 expression and reducing CDK2 expression.
Our primary endpoint was to identify the optimal I3M concentration that maximizes anti-cancer effects while minimizing toxicity to normal cells. We tested various I3M concentrations ranging from 0.1 μM to 80 μM. Consistent with prior studies, we found that 20 μM of I3M is the optimal concentration (30, 31). Subsequent tests were carried out using 20 μM of I3M. Previous research demonstrated that I3M promotes apoptosis in CLL cells by reducing Bcl-2 expression (23, 27). Li et al. (32) reported an increase in Bax expression in glioma cells under similar conditions. Zhang et al. observed that I3M treatment led to the downregulation of Bcl-2, Bcl-xl, Mcl-1, and survivin, and upregulation of Bax in osteosarcoma cells (27). However, in our study, the expression level of Bax in I3M-treated cells did not significantly differ from untreated cells. I3M treatment triggers caspase-8 activation, resulting in Bid cleavage, Bax conformational change, cytochrome c release, and cell apoptosis (33). Previous research indicated that I3M-induced apoptosis involves both anti-apoptotic and pro-apoptotic Bcl-2 family members at the mitochondrial site (18, 24), which warrants further investigation in CLL contexts.
Moreover, it was demonstrated that I3M acts as an inhibitor of CDKs and induces cell cycle arrest in both the G2/M and G1/S phases, typically followed by apoptosis. Indirubin derivatives can directly bind to the ATP-binding site of CDKs in an ATP-competitive manner, effectively blocking the cell cycle (25). Our findings in CLL cells revealed that I3M suppressed the expression of CDK2 without significantly impacting CDK1 expression. This suggests that the downregulation of CDK2 contributes to cell cycle arrest in CLL cells. The identification of CDKs as specific molecular targets of I3M was initially reported by Hoessel et al. (34) and subsequently by Marko et al. These authors demonstrated the inhibitory impact of I3M on the proliferation of the mammary carcinoma cell line (MCF-7), by arresting cells in the G2/M phase of the cell cycle, potentially through inhibiting CDK1 (35). Similarly, Liao and Leung found that I3M reduced the expression of CDK2 and cyclin E, leading to cell cycle arrest at the G0/G1 phase in the human neuroblastoma cell line (LA-N-1) (36).
5.1. Conclusions
In conclusion, our results suggest that the anti-tumor effects of I3M in CLL cells are mediated by the suppression of anti-apoptotic proteins such as Bcl-2 and the inhibition of CDK2. Additionally, I3M demonstrated minimal cytotoxicity toward normal cells. Further detailed characterization of the anti-tumor mechanisms of I3M is necessary to support its potential as a therapeutic drug for CLL.
References
-
1.
Ahmed RAEM, Osman IM. Clinical and haematological pattern of chronic lymphocytic leukaemia in sudanese patients. J Int Blood Res Rev. 2017;7(1):1-10.
-
2.
Hasan KM. Clinical Aspects, Immunophenotypic Analysis and Survival Rate of Chronic Lymphocytic Leukaemia Patients in Erbil City, Iraq. Sultan Qaboos Univ Med J. 2018;18(4):e461-7. [PubMed ID: 30988964]. [PubMed Central ID: PMC6443287]. https://doi.org/10.18295/squmj.2018.18.04.006.
-
3.
Spring LM, Zangardi ML, Moy B, Bardia A. Clinical Management of Potential Toxicities and Drug Interactions Related to Cyclin-Dependent Kinase 4/6 Inhibitors in Breast Cancer: Practical Considerations and Recommendations. Oncologist. 2017;22(9):1039-48. [PubMed ID: 28706010]. [PubMed Central ID: PMC5599204]. https://doi.org/10.1634/theoncologist.2017-0142.
-
4.
Chiorazzi N, Rai KR, Ferrarini M. Chronic lymphocytic leukemia. N Engl J Med. 2005;352(8):804-15. [PubMed ID: 15728813]. https://doi.org/10.1056/NEJMra041720.
-
5.
Fu C, Gong Y, Shi X, Sun Z, Niu M, Sang W, et al. Plumbagin reduces chronic lymphocytic leukemia cell survival by downregulation of Bcl-2 but upregulation of the Bax protein level. Oncol Rep. 2016;36(3):1605-11. [PubMed ID: 27461100]. https://doi.org/10.3892/or.2016.4950.
-
6.
Hallek M, Al-Sawaf O. Chronic lymphocytic leukemia: 2022 update on diagnostic and therapeutic procedures. Am J Hematol. 2021;96(12):1679-705. [PubMed ID: 34625994]. https://doi.org/10.1002/ajh.26367.
-
7.
Otto T, Sicinski P. Cell cycle proteins as promising targets in cancer therapy. Nat Rev Cancer. 2017;17(2):93-115. [PubMed ID: 28127048]. [PubMed Central ID: PMC5345933]. https://doi.org/10.1038/nrc.2016.138.
-
8.
Sobecki M, Mrouj K, Colinge J, Gerbe F, Jay P, Krasinska L, et al. Cell-Cycle Regulation Accounts for Variability in Ki-67 Expression Levels. Cancer Res. 2017;77(10):2722-34. [PubMed ID: 28283655]. https://doi.org/10.1158/0008-5472.CAN-16-0707.
-
9.
Decker T, Schneller F, Hipp S, Miething C, Jahn T, Duyster J, et al. Cell cycle progression of chronic lymphocytic leukemia cells is controlled by cyclin D2, cyclin D3, cyclin-dependent kinase (cdk) 4 and the cdk inhibitor p27. Leukemia. 2002;16(3):327-34. [PubMed ID: 11896535]. https://doi.org/10.1038/sj.leu.2402389.
-
10.
Zhang M, Zhang L, Hei R, Li X, Cai H, Wu X, et al. CDK inhibitors in cancer therapy, an overview of recent development. J American J Cancer Res. 2021;11(5):1913.
-
11.
Knudsen ES, Kumarasamy V, Nambiar R, Pearson JD, Vail P, Rosenheck H, et al. CDK/cyclin dependencies define extreme cancer cell-cycle heterogeneity and collateral vulnerabilities. Cell Rep. 2022;38(9):110448. [PubMed ID: 35235778]. [PubMed Central ID: PMC9022184]. https://doi.org/10.1016/j.celrep.2022.110448.
-
12.
Li XW, Rees JS, Xue P, Zhang H, Hamaia SW, Sanderson B, et al. New insights into the DT40 B cell receptor cluster using a proteomic proximity labeling assay. J Biol Chem. 2014;289(21):14434-47. [PubMed ID: 24706754]. [PubMed Central ID: PMC4031500]. https://doi.org/10.1074/jbc.M113.529578.
-
13.
Aleem E, Arceci RJ. Targeting cell cycle regulators in hematologic malignancies. Front Cell Dev Biol. 2015;3:16. [PubMed ID: 25914884]. [PubMed Central ID: PMC4390903]. https://doi.org/10.3389/fcell.2015.00016.
-
14.
Solvason N, Wu WW, Parry D, Mahony D, Lam EW, Glassford J, et al. Cyclin D2 is essential for BCR-mediated proliferation and CD5 B cell development. Int Immunol. 2000;12(5):631-8. [PubMed ID: 10784609]. https://doi.org/10.1093/intimm/12.5.631.
-
15.
Chiles TC. Regulation and function of cyclin D2 in B lymphocyte subsets. J Immunol. 2004;173(5):2901-7. [PubMed ID: 15322145]. https://doi.org/10.4049/jimmunol.173.5.2901.
-
16.
Santamaria D, Barriere C, Cerqueira A, Hunt S, Tardy C, Newton K, et al. Cdk1 is sufficient to drive the mammalian cell cycle. Nature. 2007;448(7155):811-5. [PubMed ID: 17700700]. https://doi.org/10.1038/nature06046.
-
17.
Huertas P, Cortes-Ledesma F, Sartori AA, Aguilera A, Jackson SP. CDK targets Sae2 to control DNA-end resection and homologous recombination. Nature. 2008;455(7213):689-92. [PubMed ID: 18716619]. [PubMed Central ID: PMC2635538]. https://doi.org/10.1038/nature07215.
-
18.
Biggs JR, Peterson LF, Zhang Y, Kraft AS, Zhang DE. AML1/RUNX1 phosphorylation by cyclin-dependent kinases regulates the degradation of AML1/RUNX1 by the anaphase-promoting complex. Mol Cell Biol. 2006;26(20):7420-9. [PubMed ID: 17015473]. [PubMed Central ID: PMC1636878]. https://doi.org/10.1128/MCB.00597-06.
-
19.
Xu J, Wang YY, Dai YJ, Zhang W, Zhang WN, Xiong SM, et al. DNMT3A Arg882 mutation drives chronic myelomonocytic leukemia through disturbing gene expression/DNA methylation in hematopoietic cells. Proc Natl Acad Sci U S A. 2014;111(7):2620-5. [PubMed ID: 24497509]. [PubMed Central ID: PMC3932885]. https://doi.org/10.1073/pnas.1400150111.
-
20.
Richter A, Schoenwaelder N, Sender S, Junghanss C, Maletzki C. Cyclin-Dependent Kinase Inhibitors in Hematological Malignancies-Current Understanding, (Pre-)Clinical Application and Promising Approaches. Cancers (Basel). 2021;13(10). [PubMed ID: 34065376]. [PubMed Central ID: PMC8161389]. https://doi.org/10.3390/cancers13102497.
-
21.
Kubczak M, Szustka A, Blonski JZ, Gucky T, Misiewicz M, Krystof V, et al. Dose and drug changes in chronic lymphocytic leukemia cell response in vitro: A comparison of standard therapy regimens with two novel cyclin‑dependent kinase inhibitors. Mol Med Rep. 2019;19(5):3593-603. [PubMed ID: 30864706]. [PubMed Central ID: PMC6470834]. https://doi.org/10.3892/mmr.2019.10007.
-
22.
Yue G. Inhibitory effect of indirubin on growth of some cancer cells and its mechanism. Tianjin J Traditional Chinese Med. 2008.
-
23.
Li Z, Wang H, Wei J, Han L, Guo Z. Indirubin exerts anticancer effects on human glioma cells by inducing apoptosis and autophagy. AMB Express. 2020;10(1):171. [PubMed ID: 32975633]. [PubMed Central ID: PMC7519025]. https://doi.org/10.1186/s13568-020-01107-2.
-
24.
Chen L, Wang J, Wu J, Zheng Q, Hu J. Indirubin suppresses ovarian cancer cell viabilities through the STAT3 signaling pathway. Drug Des Devel Ther. 2018;12:3335-42. [PubMed ID: 30323565]. [PubMed Central ID: PMC6174913]. https://doi.org/10.2147/DDDT.S174613.
-
25.
Perabo FG, Froessler C, Landwehrs G, Schmidt DH, Von Ruecker A, Wirger A, et al. Indirubin-3′-monoxime, a CDK inhibitor induces growth inhibition and apoptosis-independent up-regulation of survivin in transitional cell cancer. J Anticancer Res. 2006;26(3A):2129-35.
-
26.
Hallek M, Cheson BD, Catovsky D, Caligaris-Cappio F, Dighiero G, Dohner H, et al. Guidelines for the diagnosis and treatment of chronic lymphocytic leukemia: a report from the International Workshop on Chronic Lymphocytic Leukemia updating the National Cancer Institute-Working Group 1996 guidelines. Blood. 2008;111(12):5446-56. [PubMed ID: 18216293]. [PubMed Central ID: PMC2972576]. https://doi.org/10.1182/blood-2007-06-093906.
-
27.
Zhang Y, Song L, Li J, Zhang Y, Lu X, Zhang B. Inhibitory effects of indirubin-3'-monoxime against human osteosarcoma. IUBMB Life. 2019;71(10):1465-74. [PubMed ID: 31050877]. https://doi.org/10.1002/iub.2058.
-
28.
Kim SA, Kim YC, Kim SW, Lee SH, Min JJ, Ahn SG, et al. Antitumor activity of novel indirubin derivatives in rat tumor model. Clin Cancer Res. 2007;13(1):253-9. [PubMed ID: 17200363]. https://doi.org/10.1158/1078-0432.CCR-06-1154.
-
29.
Ribas J, Bettayeb K, Ferandin Y, Knockaert M, Garrofe-Ochoa X, Totzke F, et al. 7-Bromoindirubin-3'-oxime induces caspase-independent cell death. Oncogene. 2006;25(47):6304-18. [PubMed ID: 16702956]. https://doi.org/10.1038/sj.onc.1209648.
-
30.
Lee MY, Liu YW, Chen MH, Wu JY, Ho HY, Wang QF, et al. Indirubin-3'-monoxime promotes autophagic and apoptotic death in JM1 human acute lymphoblastic leukemia cells and K562 human chronic myelogenous leukemia cells. Oncol Rep. 2013;29(5):2072-8. [PubMed ID: 23468088]. https://doi.org/10.3892/or.2013.2334.
-
31.
Shi J, Shen HM. Critical role of Bid and Bax in indirubin-3'-monoxime-induced apoptosis in human cancer cells. Biochem Pharmacol. 2008;75(9):1729-42. [PubMed ID: 18377873]. https://doi.org/10.1016/j.bcp.2008.01.021.
-
32.
Li Q, Lu XH, Wang CD, Cai L, Lu JL, Wu JS, et al. Antiproliferative and apoptosis-inducing activity of schisandrin B against human glioma cells. Cancer Cell Int. 2015;15(1):12. [PubMed ID: 25685066]. [PubMed Central ID: PMC4326453]. https://doi.org/10.1186/s12935-015-0160-x.
-
33.
Shi J, Shen H. Indirubin-3’-monoxim induced apoptosis in human tumor cells via pro-apoptotic Bcl-2 family members. J Clinical Cancer Res. 2007;13(19_Supplement):B46.
-
34.
Hoessel R, Leclerc S, Endicott JA, Nobel ME, Lawrie A, Tunnah P, et al. Indirubin, the active constituent of a Chinese antileukaemia medicine, inhibits cyclin-dependent kinases. Nat Cell Biol. 1999;1(1):60-7. [PubMed ID: 10559866]. https://doi.org/10.1038/9035.
-
35.
Marko D, Schatzle S, Friedel A, Genzlinger A, Zankl H, Meijer L, et al. Inhibition of cyclin-dependent kinase 1 (CDK1) by indirubin derivatives in human tumour cells. Br J Cancer. 2001;84(2):283-9. [PubMed ID: 11161389]. [PubMed Central ID: PMC2363695]. https://doi.org/10.1054/bjoc.2000.1546.
-
36.
Liao XM, Leung KN. Indirubin-3'-oxime induces mitochondrial dysfunction and triggers growth inhibition and cell cycle arrest in human neuroblastoma cells. Oncol Rep. 2013;29(1):371-9. [PubMed ID: 23117445]. https://doi.org/10.3892/or.2012.2094.