Abstract
Background:
Deafness is recognized as the most common sensory-neural defect.Objectives:
This study aimed to investigate the effect of hopping training on the static and dynamic balance of Iraqi deaf athletes.Methods:
The current research was a semi-experimental study with a pre-test-post-test design and a control group. The statistical population included deaf male athletes aged 15 to 25 in Baghdad, Iraq. Fifty deaf athletes were purposefully selected and randomly divided into experimental and control groups. Static and dynamic balance were evaluated using the stork test and the Y balance measuring device, respectively. The experimental group underwent an eight-week hopping training program (three sessions per week, each lasting one hour). Data analysis was conducted using descriptive statistics and covariance analysis at a significance level of 0.05, using SPSS version 25.Results:
The findings revealed that eight weeks of hopping training significantly improved static balance (F = 40.58, P = 0.001, η² = 0.46), dynamic balance in the anterior direction (F = 44.60, P = 0.001, η² = 0.48), posterior-external direction (F = 36.88, P = 0.007, η² = 0.42), posterior-internal direction (F = 39.50, P = 0.004, η² = 0.43), and the total balance score (F = 42.60, P = 0.001, η² = 0.45). No significant differences were observed in the control group following the intervention.Conclusions:
This study demonstrated that an eight-week program of hopping training can enhance both static and dynamic balance performance in deaf athletes. It is recommended to incorporate this type of intervention as an effective alternative for balance training, especially in settings with limited facilities for individuals with hearing impairments.Keywords
Athlete Deaf Hopping Trainings Static Balance Dynamic Balance
1. Background
Hearing is fundamental to communication development and serves as the foundation for learning (1). Individuals who experience communication difficulties often face disruptions in their personality and social development (2, 3). Nearly 30% of people with learning disabilities also have some form of hearing loss (4, 5). Deafness is the most common sensory-neural defect, affecting approximately one in every 1,000 children born, who are either deaf or hard of hearing (2, 5). The World Health Organization estimates the global population of deaf and hard-of-hearing individuals to exceed 360 million (6). Hearing impairment is most prevalent among Asian children, reported at a rate of 6.2 per 1,000 births, compared to 0.7 per 1,000 births in non-Asian children (2, 5). Although communication difficulties are the primary challenge for individuals with hearing loss (6), these individuals may also experience physical problems, such as balance deficits (7, 8). Numerous studies on perceptual skills in the deaf population support the theory of perceptual insufficiency, indicating that hearing loss negatively impacts perceptual organization, functions, and related skills (1, 6, 9). Damage to the vestibular-cochlear nerve not only leads to hearing loss and deafness but can also result in balance issues due to vestibular nerve damage (2, 5). Research indicates that 49% to 95% of hearing-impaired and deaf individuals experience balance problems (4, 10). Balance control is a complex motor ability that involves integrating and coordinating flexible movement patterns, as well as processing sensory inputs from visual, vestibular, and sensory-motor tracking systems (11). Deficiencies in balance and changes in gait patterns due to impaired sensory-motor integration have been documented in deaf individuals (1, 8, 9). Balance is defined as the state of equilibrium achieved through coordinated action and reaction between body parts and is categorized into static and dynamic balance (12). Static balance refers to maintaining a stable body position while standing, while dynamic balance involves sustaining body position during movement or non-stationary states (2, 10). Research consistently highlights balance disturbances in deaf individuals compared to those with normal hearing. For instance, Melo et al. demonstrated that deaf children performed worse on balance tasks requiring postural control on both stable and unstable surfaces, with performance declining as the severity of deafness increased (13). Ebrahimi et al. found that deaf children, especially those with cochlear implants, are at greater risk of movement and balance impairments (14). Hartman et al., in a study on the motor performance of deaf elementary school children, reported significantly higher rates of movement problems, including balance and manipulative skills, compared to their peers with normal hearing (4). Similarly, Wuang observed poorer balance performance in hearing-impaired individuals using the paw-heel test compared to healthy controls (15).
Physical activity and training have been identified as low-cost and effective interventions to improve balance and gait in deaf individuals (9, 10, 16). Melo et al. suggested that sports and recreational training programs show promise in enhancing balance and mobility in children and adolescents with hearing impairments (10). Zhou and Qi, in a review study, reported that sports-based interventions positively impacted the balance of children and adolescents with hearing impairments, with interventions lasting 8 to 16 weeks proving more effective than shorter programs (17). Additionally, Arshad et al. found balance training to be a suitable method for improving balance in individuals with hearing impairments (9).
In the pursuit of more effective training methods to enhance athletic performance, researchers have developed and implemented various training programs, including core stability training, Pilates, and neuromuscular training. These approaches continue to be explored for their potential to improve balance and overall motor function in individuals with hearing impairments. Recently, hopping training has emerged as a dynamic method for enhancing lower limb functionality (18). This type of training incorporates eccentric contractions followed by concentric contractions, integrating muscle strength, coordination, joint stability, balance, and proprioception (19). Hopping exercises are often used as a key criterion for returning to sports, as they involve explosive initial stretching that can improve neuromuscular efficiency (8, 20). Researchers have referred to hopping training as a bridge between strength and coordination, directly enhancing athletic performance in competitive settings (18, 19).
Hosseinzadeh and Norasteh compared hopping exercises with strength and balance training, concluding that both significantly improved balance in athletes with chronic ankle instability. However, strength and balance training showed slightly greater effectiveness, particularly in the Y-balance test (21). Similarly, Ashkezari et al. examined the effects of six weeks of hopping and balance training in athletes with ankle instability. Both training methods improved key kinematic variables critical to dynamic balance during running, such as dorsiflexion and knee flexion (18). Hammami et al. further demonstrated that combining jumping and balance training could be particularly effective. Pre-conditioning with balance training, followed by jumping exercises, resulted in significant improvements in balance performance, as evidenced by increased Y-balance test scores in young athletes (22).
With increasing life expectancy worldwide, the prevalence of deafness and hearing loss has risen, leading to higher societal and familial costs. Rehabilitation researchers are therefore seeking innovative strategies to enable individuals with disabilities to perform daily tasks independently. Furthermore, participation in sports activities can foster a sense of vitality, happiness, and confidence among individuals with disabilities (5, 6). Since balance control is a fundamental prerequisite for daily activities and is closely linked to motor perception skills and cognitive processes such as memory, understanding its role in deaf individuals’ motor learning and retention is critical. The limited research in this area underscores the necessity of this study.
2. Objectives
This study aimed to investigate the effect of eight weeks of hopping training on the static and dynamic balance of Iraqi deaf athletes.
3. Methods
3.1. Study Design and Participants
The current research employed a semi-experimental design with a pre-test and post-test framework, including a control group. The statistical population comprised all deaf male athletes aged 15 to 25 years in Baghdad, Iraq, in 2023. The researchers commenced their work after presenting a letter of introduction to the management of the Deaf Association of Baghdad and making the necessary arrangements with the association's officials. The study was approved with ethical code IR.IAU.KHUISF.REC.1402.386 by the Islamic Azad University of Isfahan, Khorasgan Branch.
Fifty individuals with total deafness (hearing loss exceeding 90 dB) were selected based on a review of their medical records. The G*Power program was used to calculate the test power, ensuring a measurable control of 80% (1-β = 0.80) with an alpha level of 0.05, which is widely accepted for producing rigorous and valid results. This calculation was informed by a pilot study with the same sample size, providing sufficient statistical power to detect significant differences between the groups.
The sample size estimation was based on published effect sizes from similar interventions in balance training. Specifically, studies such as Ashkezari et al. reported effect sizes ranging from 0.5 to 0.6 for improvements in dynamic balance following hopping training (18). The 50 participants were randomly assigned to either the experimental group (25 individuals) or the control group (25 individuals). Figure 1 presents a CONSORT diagram detailing the flow of participants through each stage of the study.
Consort chart
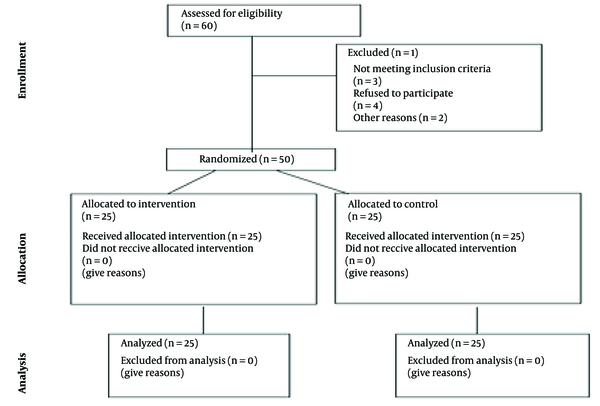
3.2. Inclusion Criteria
The inclusion criteria for this research were as follows: Participants with deafness exceeding 90 decibels, aged between 15 to 25 years, male gender, active athletes with at least 5 years of regular exercise history, no visible abnormalities in the lower limbs, no specific diseases or conditions such as respiratory or cardiovascular disorders, no use of medications affecting the central nervous system, no history of neuromuscular diseases, no history of spinal or upper and lower limb surgeries within the past year, and no fractures, dislocations, or sprains in the lower or upper limbs within the last 6 months.
3.3. Exclusion Criteria
Failure to participate in two consecutive training sessions or three non-consecutive training sessions, the occurrence of musculoskeletal pain following the training, or withdrawal of consent to participate in the research.
3.4. Measuring Instruments
3.4.1. Static Balance; Stork Test
This test was developed by Johnson and Nelson (1969). Subjects were instructed to place their hands on their waist while positioning the sole of their non-dominant foot against the inner region of the dominant leg near the knee. The subjects then stood on the ball of their dominant foot for as long as possible, maintaining this position. The test concluded when the heel of the dominant foot touched the floor, the hands were removed from the waist, or the sole of the non-dominant foot was detached from the knee of the dominant leg. Each subject performed two attempts with a 15-second rest interval, and the best time, recorded in seconds, was noted as the subject's score. Johnson and Nelson reported the validity and reliability of this test as 0.87 and 0.83, respectively (23).
3.4.2. Dynamic Balance; Star Balance Test
The Y balance test, a modified version of the star balance test, was utilized to assess dynamic balance. In this test, three directions (anterior, external, and posterior) were marked, each separated by an angle of 135 degrees. After receiving detailed instructions on the test procedure, each subject practiced the test six times to familiarize themselves with the method. The subject stood on one leg at the center of the star and, using the other leg, reached maximally in the direction specified by the examiner before returning to the starting position without errors. To minimize the learning effect, each subject practiced reaching in each direction six times, with a 15-second rest between attempts.
Following a 5-minute rest period, the subject performed the reach in directions randomly selected by the examiner, who measured the distance (in centimeters) from the center of the star to the furthest point of contact made by the subject’s foot. Gribble and Hertel reported the validity and reliability of this test as 0.81 and 0.96, respectively (24). The composite score for each direction and the total score (mean) across all directions were calculated for data analysis.
3.5. Ethical Considerations
Informed consent was obtained from all participants. Confidentiality and anonymity of the excluded subjects were maintained and strictly upheld throughout the study.
3.6. Procedure
All evaluations for each participant were conducted in a single session lasting approximately 45 minutes. It should be noted that static and dynamic balance assessments were carried out under standard conditions, with minimal clothing, in full adherence to ethical principles and social distancing. These evaluations took place in a covered gymnasium with an appropriate ambient temperature and adequate lighting, while strictly following health protocols. Communication with the deaf participants was facilitated by sign language interpreters who were trainers from the Deaf Association.
After familiarizing participants with the research process, obtaining written consent from their families, and assuring them about the confidentiality of the information, ethical considerations were observed. The degree of deafness for each participant was recorded. Anthropometric characteristics, including height, weight, and body mass index, were measured and documented for both groups. Subsequently, in two stages—pre-test and post-test—the static and dynamic balance of both the control and experimental groups was assessed. All research evaluations and measurements were entirely non-invasive and posed no risk to the health of the participants. Static balance was evaluated using the one-leg stand test, while dynamic balance was assessed using the modified Y-balance test. The dominant leg was identified based on which leg the participant would preferentially use to kick a soccer ball.
Stretching and flexibility exercises for warming up and cooling down were incorporated at the beginning and end of each training session. Participants in the experimental group performed hopping exercises for eight weeks (three sessions per week, one hour per session) under the supervision of the researcher. Each session began with a kinetic warm-up phase involving light jogging for five minutes, followed by stretching exercises targeting the hamstrings, quadriceps, gluteal muscles, and calves for another five minutes. After the warm-up, participants in the experimental group completed 60 minutes of hopping exercises under the trainer's supervision. Each session concluded with a five-minute cool-down phase.
The training program lasted for eight weeks. During this time, participants in the control group were instructed to maintain their regular daily activities and refrain from engaging in any structured sports activities. At the end of the eight weeks, static and dynamic balance assessments were conducted again for both the control and experimental groups during the post-test phase.
3.7. Intervention
The training program included six types of hopping exercises performed over eight weeks, with three sessions per week, each lasting 60 minutes (Table 1). The training intensity followed the principles of overload and progression, incorporating additional movements each week to ensure gradual advancement. The protocol comprised forward hopping, square hopping, sideways hopping, forward-backward hopping, zigzag hopping, and Latin eight hopping, designed based on previous research (25, 26).
Training Intervention
Weeks | Training Volume (Number of Jump) | Type of Training (Hopping) | Set | Repetition |
---|---|---|---|---|
First | 70 | Sideways with both legs (hands free) | 10 | 3 |
Forward-backward with two feet (hands free) | 10 | 2 | ||
By moving forward with both feet (hands free) | 10 | 2 | ||
Second | 90 | Sideways with both legs (hands on the chest) | 15 | 2 |
Forward-backward with two feet (hands free) | 10 | 2 | ||
By moving forward with both feet (hands free) | 10 | 2 | ||
Sideways with one leg (hands free) | 4 | 5 | ||
Third | 100 | Sideways with one leg (hands on the chest) | 10 | 3 |
Forward-backward with one leg (hands free) | 10 | 2 | ||
By moving forward with both legs (hands on the chest) | 10 | 3 | ||
Zigzag with two legs (hands free) | 10 | 2 | ||
Fourth | 110 | Sideways with one leg (hands on the chest) | 10 | 2 |
Forward-backward with one leg (hands on the chest) | 10 | 2 | ||
By moving forward with one leg (hands free) | 10 | 3 | ||
Zigzag with one leg (hands free) | 10 | 2 | ||
In a square with two feet (hands free) | 10 | 2 | ||
Fifth and sixth | 120 | Sideways with one leg (hands behind the head) | 10 | 2 |
Forward with one leg (hands behind the head) | 10 | 2 | ||
By moving forward with one leg (hands on the chest) | 10 | 2 | ||
Zigzag with one leg (hands on chest) | 10 | 2 | ||
In a square with one leg (hands free) | 10 | 2 | ||
In a Latin figure of eight with two legs (hands free) | 10 | 2 | ||
Seventh and eighth | 130 | sideways with one leg (hands behind the head) | 10 | 2 |
Forward-backwards with one leg | 10 | 3 | ||
By moving forward with one leg (hands behind the head) | 10 | 2 | ||
Zigzag with one leg (hands behind the head) | 10 | 2 | ||
In a square with one leg (hands on the chest) | 10 | 2 | ||
In a Latin figure of eight with one leg (hands free) | 10 | 2 |
Participants performed the exercises sequentially, with 30 seconds of rest between each set and a 2-minute rest between different exercises. The training volume for each session included 70-to-130-foot contacts with the ground, regulated by a metronome or the speed of execution. Initially, the exercises were performed using both legs, and as participants developed proficiency, the exercises were progressively adapted to be performed on one leg in subsequent weeks (26).
3.8. Statistical Analysis
To analyze the data, central tendency and dispersion indices were used at the descriptive level. The normality of the data and the homogeneity of variances were assessed using the Shapiro-Wilk and Levene’s tests, respectively. An independent t-test was conducted to compare demographic characteristics between groups. The intervention's effect was evaluated through ANCOVA, while the within-group effect of time was examined using repeated measures ANOVA. All statistical analyses were performed using SPSS version 25, with an alpha level of 0.05 set for significance. Effect size (n2) was calculated for all parameters, with values of 0.02, 0.13, and 0.26 interpreted as small, medium, and large effects, respectively (27).
4. Results
4.1. Demographic Characteristics
The demographic characteristics of the participants are presented in Table 2.
Demographic Characteristics of the Participants
Variables and Groups | Lowest Amount | Maximum Amount | Mean ± SD | t | P |
---|---|---|---|---|---|
Age (y) | 0.35 | 0.72 | |||
Experimental | 15.00 | 20.00 | 17.24 ± 1.76 | ||
Control | 14.00 | 20.00 | 17.40 ± 1.38 | ||
Height (cm) | 0.08 | 0.93 | |||
Experimental | 164 | 180 | 169.04 ± 5.03 | ||
Control | 163 | 181 | 168.92 ± 5.34 | ||
Weight(kg) | 1.64 | 0.10 | |||
Experimental | 67.00 | 73.00 | 66.48 ± 4.22 | ||
Control | 65.00 | 74.00 | 68.28 ± 3.47 | ||
Body Mass Index | 1.23 | 0.22 | |||
Experimental | 18.21 | 26.44 | 24.00 ± 1.97 | ||
Control | 20.75 | 27.51 | 23.31 ± 1.92 |
According to the results, the average age in the control group was 17.40 ± 1.38, while in the experimental group, it was 17.24 ± 1.76. The average height in the control group was 168.92 ± 5.34, and in the experimental group, it was 169.04 ± 5.05. The average weight in the control group was 68.28 ± 3.47, while in the experimental group, it was 66.48 ± 4.22. Finally, the average Body Mass Index (BMI) in the control group was 23.31 ± 1.92, and in the experimental group, it was 24.00 ± 1.97. The results of the independent t-test did not indicate any significant differences in the mean demographic characteristics between the groups (P > 0.05). The effect of the intervention was evaluated using ANCOVA, while repeated measures ANOVA was applied to examine the effect of time within groups.
4.2. Static Balance
Before analyzing the data, the Shapiro-Wilk test was applied to assess the normality of data distribution. The results confirmed that the data distribution was normal (P > 0.05). Additionally, Levin's test results validated the homogeneity of variances (P > 0.05). The differences in the average static balance scores of the subjects between the pre-test and post-test phases are presented in Table 3.
Comparing the Average Scores of Static and Dynamic Balance of the Participants
Variables and Groups | Mean Differences | F | P | 95%CI | Eta |
---|---|---|---|---|---|
Static balance | |||||
Experimental | 2.48 ± 1.63 | 7.57 | 0.001 | 0.43, 1.68 | 0.98 |
Control | 0.36 ± 1.70 | 1.05 | 0.30 | -1.06, 0.34 | 0.04 |
Dynamic Balance | |||||
Anterior | |||||
Experimental | 2.20 ± 2.21 | 4.96 | 0.001 | 0.13, 1.22 | 0.99 |
Control | 0.48 ± 1.63 | 0.96 | 0.26 | -1.47, -0.20 | 0.02 |
Posterior-external | |||||
Experimental | 2.67 ± 4.20 | 3.28 | 0.003 | 0.43, 2.25 | 0.99 |
Control | 0.08 ± 1.68 | 0.23 | 0.81 | -0.77, 0.61 | 0.002 |
Posterior-internal | |||||
Experimental | 2.88 ± 3.08 | 3.10 | 0.002 | 0.85, 2.31 | 0.98 |
Control | 0.36 ± 3.52 | 0.70 | 0.61 | -1.45, 0.33 | 0.06 |
Total score | |||||
Experimental | 3.36 ± 1.98 | 7.57 | 0.001 | 0.68, 1.72 | 0.99 |
Control | 0.45 ± 2.48 | 0.81 | 0.42 | -0.64, 0.39 | 0.01 |
Based on the results presented in Table 3, the average static balance (P = 0.001) of the deaf athletes in the experimental group was significantly higher in the post-test compared to the pre-test. However, in the control group, there was no significant difference in the average static balance scores (P > 0.05). According to the results in Table 4, after controlling for the pre-test effect, a significant difference in the post-test scores of static balances was observed between the experimental and control groups (F = 40.58, P = 0.001, n² = 0.46). In other words, the static balance of deaf athletes in the experimental group improved significantly compared to the control group.
Results of Covariance Analysis of Static and Dynamic Balance of Participants
Variables | F | P | Eta |
---|---|---|---|
Static balance | 142.05 | 0.001 | 0.75 |
40.58 | 0.001 | 0.46 | |
Dynamic balance | |||
Anterior | 129.28 | 0.001 | 0.73 |
44.60 | 0.001 | 0.48 | |
Posterior-external | 37.70 | 0.001 | 0.41 |
36.88 | 0.007 | 0.42 | |
Posterior-internal | 43.91 | 0.001 | 0.48 |
39.50 | 0.004 | 0.43 | |
Total score | 1501.563 | 0.001 | 0.97 |
42.60 | 0.001 | 0.45 |
4.3. Dynamic Balance
The difference between the average scores of the subjects' dynamic balance in the pre-test and post-test phases is presented in Table 3.
Based on the results in Table 3, the average anterior dynamic balance (P = 0.001), posterior-external dynamic balance (P = 0.003), posterior-internal dynamic balance (P = 0.002), and total dynamic balance score (P = 0.001) of the deaf athletes in the experimental group were significantly higher in the post-test compared to the pre-test. However, in the control group, there was no significant difference in the average dynamic balance scores (P > 0.05). According to the results in Table 4, after controlling for the pre-test effect, a significant difference in the post-test scores of anterior dynamic balance (F = 44.60, P = 0.001, n² = 0.48), posterior-external dynamic balance (F = 36.88, P = 0.007, n² = 0.42), posterior-internal dynamic balance (F = 39.50, P = 0.004, n² = 0.43), and the total dynamic balance score (F = 42.60, P = 0.001, n² = 0.45) was observed between the experimental and control groups. In other words, the dynamic balance of deaf athletes in the experimental group improved significantly compared to the control group.
5. Discussion
The purpose of this research was to investigate the effect of eight weeks of hopping training on the static and dynamic balance of Iraqi deaf athletes compared to a control group. According to the results, after implementing the hopping training program, a significant improvement was observed in the static and dynamic balance of deaf athletes in the experimental group compared to the control group. These findings align with studies suggesting that hopping training interventions can enhance the static and dynamic balance of deaf athletes (2, 8, 9, 16).
Studies have shown that hearing impairment does not necessarily hinder the development of physical and motor fitness in athletes. Participation in sports activities and the design of training sessions that consider the specific characteristics of athletes with hearing impairments are essential factors in enhancing their performance (28, 29). However, the results of this research contrast with those of Anthony, who investigated the effect of four weeks of core stability and balance training on static and dynamic balance and found no significant improvement in balance performance (7). This inconsistency could be attributed to differences in balance assessment methods, sample sizes, and the severity of participants' deafness.
Balance control relies on the ability to integrate afferent information from the vestibular, visual, and somatosensory systems (16). A functional reduction in any of these systems or the loss of their information can impair postural control (13). The central nervous system plays a crucial role in coordinating the contraction of agonist and antagonist muscles, a process essential for the lower limbs and balance control (30, 31). Coordination between the trunk and thigh muscles is critical for maintaining spinal stability, as no single muscle is solely responsible for balancing the spine (30). Hearing loss, in addition to affecting balance, can decrease self-confidence and increase the risk of falls. Consequently, sports training can enhance afferent information from the various systems involved in balance (16, 17).
Numerous studies have highlighted hopping training as an effective intervention for increasing neuromuscular adaptation and lower limb muscle strength (18, 19). The strength of the muscles surrounding the joints and their co-contraction to stabilize the lower limb joints, combined with the activity of proprioceptors and neuromuscular control to maintain balance during directional reach tasks, are vital for achieving optimal performance (1, 8). Kuitunen et al. suggested that hopping training promotes neuromuscular adaptation, enabling faster and more powerful execution of movements and improving overall performance (32).
Compared to similar interventions, hopping training not only engages the contractile elements of muscles (muscle fibers) but also activates non-contractile components (20). Latent tensile energy, akin to a stretched spring’s energy, is stored within the elastic components of muscles during stretching and is released during muscle contraction, enhancing the strength and speed of the contraction (19). The faster the muscle stretch, the stronger the subsequent contraction (33). Muscle strength and balance are fundamental aspects of hopping training (18, 20), which creates a strong link between strength and coordination, ultimately enhancing athletic performance (19).
Improving muscle strength can result in shifting the body's center of gravity toward the ankle joint (34). This strengthening aligns the center of gravity in a more desirable and stable position relative to the ankle joint, thereby enhancing balance and reducing the risk of falls (35). These changes primarily occur through the strengthening of muscles in the back, thighs, and legs, especially large muscle groups such as the quadriceps and hamstrings (34, 36).
Additionally, improvements in the endurance and strength of core muscles, including the abdominal muscles, multifidus, pelvic muscles, and diaphragm, may also contribute to balance enhancement following the hopping training protocol. These muscles play a crucial role in central stability (37). Central stability training engages these muscles to build their strength and endurance, thereby improving the body’s sensory-motor control (19, 20).
Hopping training, in particular, fosters trunk stability and minimizes excessive forces on the spine (18). Given these benefits, this training method appears to be an effective approach for preventing balance issues and improving overall balance in individuals with such needs.
5.1. Limitations
The limitations of the present study include the exclusion of both genders, the inability to control variables such as daily activity levels, weather conditions, sleep quality and duration, nutrition, and rest of the participants. Additionally, the absence of a retention test to evaluate the long-term effects of the research intervention further restricts the scope of the findings.
5.2. Conclusions
According to the results of the present research, it can be concluded that hopping training significantly improves the static and dynamic balance of deaf athletes. Therefore, this training program can be effectively utilized to achieve various objectives, including enhancing sports performance, supporting rehabilitation and recovery from injuries, and improving overall health and physical fitness. Furthermore, the simplicity, low cost, and accessibility of hopping training make it a practical intervention, offering the added benefit of reducing injury risks for athletes.
Acknowledgements
References
-
1.
Coskun B, Unlu G, Golshaei B, Kocak S, Kirazcı S. Comparison of the Static and Dynamic Balance Between Normal-Hearing and Hearing-Impaired Wrestlers. Montenegrin Journal of Sports Science and Medicine. 2019;8(1):11-6. https://doi.org/10.26773/mjssm.190302.
-
2.
Subramani A. Impact of specific balance training on static balance and dynamic balance among hearing impaired students. International J Advance Res Innovative Ideas Edu. 2015;1(4):757-61.
-
3.
Prizant BM, Audet LR, Burke GM, Hummel LJ, Maher SR, Theadore G. Communication disorders and emotional/behavioral disorders in children and adolescents. J Speech Hear Disord. 1990;55(2):179-92. [PubMed ID: 2184286]. https://doi.org/10.1044/jshd.5502.179.
-
4.
Hartman E, Houwen S, Visscher C. Motor skill performance and sports participation in deaf elementary school children. Adapt Phys Activ Q. 2011;28(2):132-45. [PubMed ID: 21757785]. https://doi.org/10.1123/apaq.28.2.132.
-
5.
Hey C, Fessler S, Hafner N, Lange BP, Euler HA, Neumann K. High prevalence of hearing loss at the special olympics: is this representative of people with intellectual disability? J Appl Res Intellect Disabil. 2014;27(2):125-33. [PubMed ID: 23610001]. https://doi.org/10.1111/jar.12057.
-
6.
Hauser PC, O'Hearn A, McKee M, Steider A, Thew D. Deaf epistemology: Deafhood and Deafness. Am Ann Deaf. 2010;154(5):486-92. discussion 493-6. [PubMed ID: 20415284]. https://doi.org/10.1353/aad.0.0120.
-
7.
Anthony Jr BP. The comparative effects of four-week core stabilization and balance-training programs on semidynamic and dynamic balance. West Virginia University; 2003.
-
8.
Andiana O, Raharjo S, Pribadi DR. The Effect of Jump Rope Training Program on the Agility in Deaf Children SLB-B Yayasan Pendidikan Tunas Bangsa (YPTB) Malang City. Sports Medicine Curiosity Journal. 2024;2(2):81-6. https://doi.org/10.15294/smcj.v2i2.78676.
-
9.
Arshad N, Rasool AG, Ashfaq M. Effects of Balance Training Exercises for Improving Static and Dynamic Balance in Deaf Population: A Randomized Controlled Trial. Pakistan Journal of Rehabilitation. 2022;11(1):110-6. https://doi.org/10.36283/pjr.zu.11.1/020.
-
10.
Melo RS, Tavares-Netto AR, Delgado A, Wiesiolek CC, Ferraz KM, Belian RB. Does the practice of sports or recreational activities improve the balance and gait of children and adolescents with sensorineural hearing loss? A systematic review. Gait Posture. 2020;77:144-55. [PubMed ID: 32036319]. https://doi.org/10.1016/j.gaitpost.2020.02.001.
-
11.
Bakhshipour E, Rahnama N, Sourtiji H, Skandari Z, Izadi Najafabadi S. [Comparing the effects of an aerobic exercise program and group-based play therapy on the balance of children with Attention Deficit Hyperactive Disorder (ADHD)]. J Res Rehabilitation Sci. 2013;9(2):161-70. FA.
-
12.
Norasteh AA, Zarei H. Studying Balance in Deaf People: A Systematic Review Study. Journal of Rehabilitation. 2019:2-15. https://doi.org/10.32598/rj.20.1.2.
-
13.
Melo Rde S, Lemos A, Macky CF, Raposo MC, Ferraz KM. Postural control assessment in students with normal hearing and sensorineural hearing loss. Braz J Otorhinolaryngol. 2015;81(4):431-8. [PubMed ID: 25382425]. [PubMed Central ID: PMC9442674]. https://doi.org/10.1016/j.bjorl.2014.08.014.
-
14.
Ebrahimi A, Movallali G, Jamshidi A, Haghgoo HA, Rahgozar M. Balance performance of deaf children with and without cochlear implants. Acta Medica Iranica. 2016;54(11):737-42.
-
15.
Wuang YP, Wang CC, Huang MH, Su CY. Profiles and cognitive predictors of motor functions among early school-age children with mild intellectual disabilities. J Intellect Disabil Res. 2008;52(12):1048-60. [PubMed ID: 18557969]. https://doi.org/10.1111/j.1365-2788.2008.01096.x.
-
16.
Zarei H, Norasteh AA, Lieberman LJ, Ertel MW, Brian A. The impacts of exercise training programs on balance in children with hearing loss: A systematic review and meta-analysis. J Bodyw Mov Ther. 2024;37:296-307. [PubMed ID: 38432821]. https://doi.org/10.1016/j.jbmt.2023.11.050.
-
17.
Zhou Y, Qi J. Effectiveness of Interventions on Improving Balance in Children and Adolescents With Hearing Impairment: A Systematic Review. Front Physiol. 2022;13:876974. [PubMed ID: 35651874]. [PubMed Central ID: PMC9150273]. https://doi.org/10.3389/fphys.2022.876974.
-
18.
Ashkezari MHK, Sahebozamani M, Daneshjoo A, Bafghi HA. [Comparison of the Effect of 6 Weeks of Balancing and Hopping Strengthening Training on the Kinematics of the Lower Extremities of Athletes with Functional Ankle Instability while Running: A Randomized Controlled Trial]. J Shahid Sadoughi Univ Med Sci. 2020;26(7). FA. https://doi.org/10.18502/ssu.v28i7.4269.
-
19.
Engeroff T, Kalo K, Merrifield R, Groneberg D, Wilke J. Progressive daily hopping exercise improves running economy in amateur runners: a randomized and controlled trial. Sci Rep. 2023;13(1):4167. [PubMed ID: 36914662]. [PubMed Central ID: PMC10011548]. https://doi.org/10.1038/s41598-023-30798-3.
-
20.
Sancho I, Morrissey D, Willy RW, Barton C, Malliaras P. Education and exercise supplemented by a pain-guided hopping intervention for male recreational runners with midportion Achilles tendinopathy: A single cohort feasibility study. Phys Ther Sport. 2019;40:107-16. [PubMed ID: 31518777]. https://doi.org/10.1016/j.ptsp.2019.08.007.
-
21.
Hosseinzadeh F, Norasteh A. Comparing the effects of hopping and strength-balance exercises on the selected muscles strength and balance in male athletes with chronic ankle instability. The Scientific Journal of Rehabilitation Medicine. 2022;11(5):794-809. https://doi.org/10.32598/sjrm.11.5.2.
-
22.
Hammami M, Negra Y, Aouadi R, Shephard RJ, Chelly MS. Effects of an In-season Plyometric Training Program on Repeated Change of Direction and Sprint Performance in the Junior Soccer Player. J Strength Cond Res. 2016;30(12):3312-20. [PubMed ID: 27135476]. https://doi.org/10.1519/JSC.0000000000001470.
-
23.
Johnson BL, Nelson JK. Practical measurements for evaluation in physical education. Burgess Publishing Company. 1969.
-
24.
Gribble PA, Hertel J. Considerations for Normalizing Measures of the Star Excursion Balance Test. Measurement in Physical Education and Exercise Science. 2003;7(2):89-100. https://doi.org/10.1207/s15327841mpee0702_3.
-
25.
Ageberg E, Zatterstrom R, Friden T, Moritz U. Individual factors affecting stabilometry and one-leg hop test in 75 healthy subjects, aged 15-44 years. Scand J Med Sci Sports. 2001;11(1):47-53. [PubMed ID: 11169235]. https://doi.org/10.1034/j.1600-0838.2001.011001047.x.
-
26.
Caffrey E, Docherty CL, Schrader J, Klossner J. The ability of 4 single-limb hopping tests to detect functional performance deficits in individuals with functional ankle instability. J Orthop Sports Phys Ther. 2009;39(11):799-806. [PubMed ID: 19881005]. https://doi.org/10.2519/jospt.2009.3042.
-
27.
Pallant J. SPSS Survival Manual. 2020. https://doi.org/10.4324/9781003117452.
-
28.
Gaweł E, Soto-Rey J, Zwierzchowska A, Perez-Tejero J. Trends and Future Directions in the Sports Performance of Deaf and Hard-of-Hearing Athletes: A Systematic Review. Applied Sciences. 2024;14(16). https://doi.org/10.3390/app14166860.
-
29.
Kordić M, Karić J. Guidelines for working with deaf athletes. Fizicka kultura. 2022;76(1):19-26. https://doi.org/10.5937/fizkul76-39360.
-
30.
Mazzocchio R, Caleo M. More than at the neuromuscular synapse: actions of botulinum neurotoxin A in the central nervous system. Neuroscientist. 2015;21(1):44-61. [PubMed ID: 24576870]. https://doi.org/10.1177/1073858414524633.
-
31.
Williams JL, Holman DW, Klein RS. Chemokines in the balance: maintenance of homeostasis and protection at CNS barriers. Front Cell Neurosci. 2014;8:154. [PubMed ID: 24920943]. [PubMed Central ID: PMC4036130]. https://doi.org/10.3389/fncel.2014.00154.
-
32.
Kuitunen S, Avela J, Kyrolainen H, Nicol C, Komi PV. Acute and prolonged reduction in joint stiffness in humans after exhausting stretch-shortening cycle exercise. Eur J Appl Physiol. 2002;88(1-2):107-16. [PubMed ID: 12436277]. https://doi.org/10.1007/s00421-002-0669-2.
-
33.
Trowbridge CA. The effects of strength and plyometric training on joint position, joint moments, and joint stiffness at the knee. Brigham Young University; 2004.
-
34.
Blackburn T, Guskiewicz KM, Petschauer MA, Prentice WE. Balance and Joint Stability: The Relative Contributions of Proprioception and Muscular Strength. Journal of Sport Rehabilitation. 2000;9(4):315-28. https://doi.org/10.1123/jsr.9.4.315.
-
35.
Tesio L, Rota V. The Motion of Body Center of Mass During Walking: A Review Oriented to Clinical Applications. Front Neurol. 2019;10:999. [PubMed ID: 31616361]. [PubMed Central ID: PMC6763727]. https://doi.org/10.3389/fneur.2019.00999.
-
36.
Kaminishi K, Chiba R, Takakusaki K, Ota J. Increase in muscle tone promotes the use of ankle strategies during perturbed stance. Gait Posture. 2021;90:67-72. [PubMed ID: 34411975]. https://doi.org/10.1016/j.gaitpost.2021.08.003.
-
37.
Irez GB, Ozdemir RA, Evin R, Irez SG, Korkusuz F. Integrating pilates exercise into an exercise program for 65+ year-old women to reduce falls. J Sports Sci Med. 2011;10(1):105-11. [PubMed ID: 24149302]. [PubMed Central ID: PMC3737905].