Abstract
Background:
Muscle ultrasonography (US) can be used to evaluate muscle quantity and quality affected by a neuromuscular disease and aging. The evaluation of muscle thickness and echo intensity using US are useful ways of estimating muscle mass and composition. During US, diligent attention to steadying the position, orientation, and inward pressure of a transducer is required; however, little is known about the quantitative influence of different inward pressures of the transducer on muscle thickness and echo intensity during US.Objectives:
The purpose of this study was to quantify the changes in muscle thickness and echo intensity induced by different inward pressures of the transducer during US.Materials and Methods:
Fifteen healthy male volunteers (mean ± SD, 20.7 ± 0.7 years old) participated in the study. Thickness and echo intensity of the right rectus femoris muscle (RF) was measured by US in the following three conditions: inward pressures of 0.5 N, 1.0 N, and 2.0 N. A repeated-measures ANOVA was utilized to determine the influence of inward pressure on the thickness and echo intensity of the RF.Results:
Muscle thickness was significantly decreased during increased inward pressures of the transducer among the three conditions (P < 0.001). On the other hand, echo intensity from the 0.5-N to 1.0-N condition was significantly decreased (P = 0.002), and from the 1.0-N to 2.0-N condition, it was significantly increased (P = 0.019).Conclusions:
Our results indicate that changes in muscle thickness and echo intensity are induced by different inward pressures of the transducer during US. When using a technique that involves a handheld transducer, the examiner should attempt to maintain consistent inward pressure of the transducer during US, to quantify the minimal change of the RF mass and composition.Keywords
1. Background
Muscle ultrasonography (US) can be used to evaluate the muscle quantity and quality affected by neuromuscular disorders and aging (1). Muscle thickness measured by US is strongly correlated with site-matched skeletal muscle mass measured using magnetic resonance imaging (2, 3). Muscle composition can also be evaluated using US, whereby enhanced echo intensity represents changes caused by increased intramuscular fibrous and adipose tissue (4, 5). Therefore, the evaluation of muscle thickness and echo intensity using US are useful ways of estimating muscle mass and composition.
During US, diligent attention to steadying the position, orientation, and inward pressure of the transducer is required. Reimers et al. (6) indicated that excessive transducer pressure may produce falsely small muscle thickness and falsely increased muscle echo intensity as measured by US (7, 8). However, little is known about the quantitative influence of different inward pressures of the transducer on the muscle thickness and echo intensity during US. Though previous studies showed the influence of inward pressures of the transducer on trunk muscle thickness during US , the rectus femoris muscle (RF) is one of the typical muscles used to evaluate muscle thickness and echo intensity in individuals with neuromuscular disorders (1).
2. Objectives
The purpose of this study was to quantify the changes in the RF muscle thickness and echo intensity induced by different inward pressures of the transducer during US.
3. Materials and Methods
3.1. Participants
Participants were recruited using advertisements aimed at physiotherapy students from Kawasaki University of Medical Welfare. Fifteen healthy male volunteers were recruited. The subjects’ ages, heights, weights, and BMI (mean ± standard deviation) were 20.7 ± 0.7 years old, 169.2 ± 4.8 cm, 60.5 ± 8.1 kg, and 21.1 ± 2.4 kg/m2, respectively. None had any history of limb surgery or neuromuscular disorders. The ethics committee of the Kawasaki University of Medical Welfare approved the protocol for this study. Written informed consent was obtained, and the rights of the subjects were protected.
3.2. Procedure
One investigator was responsible for collecting US data. The investigator had five years of experience in muscle US. B-mode ultrasound (Aloka, SSD-3500SX; Aloka co. Ltd. Tokyo, Japan) with a 10-MHz linear transducer was used to measure US of the RF. The equipment settings included gain (49 dB), dynamic range (56 dB), and time gain compensation in the neutral position, and these parameters were maintained for all measurements. The depth setting was fixed at 5 cm. A custom-made holder was used to enable hands-free application of the ultrasound transducer, which could maintain inward pressures of approximately 0.5 N, 1.0 N, and 2.0 N and the use of three constant-force springs (CR-1; CR-2; CR-3, Accurate Co. Ltd., Tokyo, Japan) (Figure 1) (7). The participants were positioned in a left side-lying posture with both hips positioned at 90° flexion and both knees positioned at 90° flexion. The height of towels between the knees was adjusted to attain both hips’ position of 0° abduction. Gel was interposed between the transducer and skin; the transducer was then placed transversely on the anterior of the right RF at 60% of the distance from the greater trochanter to the lateral epicondyle of the femur (Figure 1) (9). To avoid refraction artifacts and the deflection phenomenon, imaging was performed with the transducer in short-axis with respect to the RF (6). The transducer was tilted so as to image clearly the epimysium of the RF. Furthermore, the location of the transducer holder was fixed. After fixation, we could therefore repeat testing under different inward pressures using the same position and orientation of the transducer holder. One set of the three conditions, 0.5 N, 1.0 N, and 2.0 N, were performed in random order. Data were collected twice to examine the reliability of the measured values. Between the two sets, the participant was instructed to stand up and then reposition himself in the side-lying posture. The resulting pictures were stored as JPEG files and had a resolution of 640 × 480 pixels (Figure 2). The anterior-posterior muscle thickness of the RF (mm) was measured as the length between the superficial and deep epimysium of the RF using Image-J (National Institute of Health, USA, version 1.45). Echo intensity was determined via gray-scale analysis using the standard histogram function in Image-J. A region of interest was drawn by hand to include as much of the RF as possible without any surrounding fascia. The echo intensity in the region of interest was expressed in values between 0 and 256 (0: black; 256: white). The same investigator made all measurements of muscle thickness and echo intensity. We took the average of the two muscle thickness and echo intensity measurements for each condition as a representative value.
Position of Transducer for Ultrasonography of the Rectus Femoris Muscle
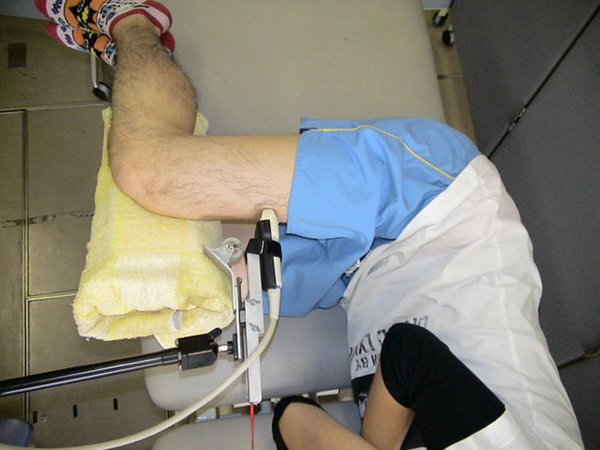
Ultrasonography of the Rectus Femoris Muscle
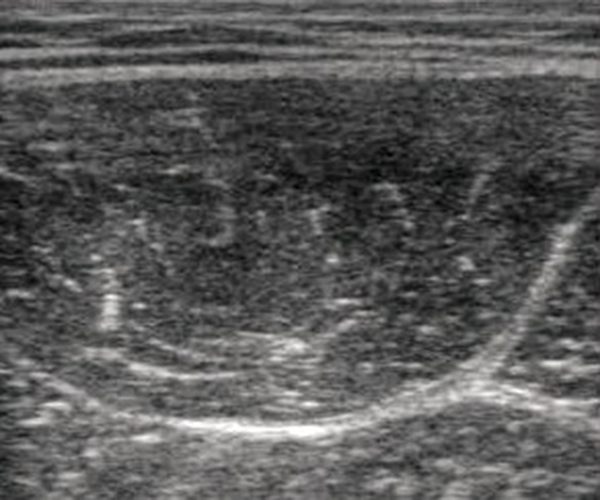
3.3. Statistical Analysis
SPSS statistics 22.0 was used for the statistical analysis. The reliability of the measured values of muscle thickness and echo intensity of the RF were examined by calculating the intraclass correlation coefficient (ICC1, 2). The standard error of measurement (SEM = SD × √(1 – ICC)) and the minimal detectable change for a 95% confidence interval (MDC = SEM × √2 × 1.96) were calculated (10). One-way repeated-measures analysis of variance (ANOVA) for each variable was used to assess differences, and a post-hoc analysis was performed with the Bonferroni test. The level of significance was set at P < 0.05. We also used G-Power software (Franz Faul, Univesitat Kiel, Germany) to calculate the post-hoc effect size and the actual power of the sample.
4. Results
The reliability of the measured values is listed in Table 1. Significant differences (ANOVA results) were observed in all parameters (Table 2). Muscle thickness and echo intensity of the RF are listed in Table 2. Significant differences of mean values between data gathered using Bonferroni’s test are listed in Table 3. Muscle thickness was significantly different among the three conditions; the mean difference in magnitude of muscle thickness between the 0.5-N and 2.0-N conditions was greater than the MDC of the 0.5-N and 2.0-N conditions. Echo intensity of the 1.0-N condition was significantly lower than that of the 0.5-N condition, and echo intensity of the 2.0-N condition was significantly higher than that of the 1.0-N condition. The mean difference in magnitude of echo intensity between the 0.5-N and 1.0-N conditions was smaller than the MDC of the 0.5-N and 1.0-N conditions, and the mean difference in magnitude of echo intensity between the 1.0-N and 2.0-N conditions was smaller than the MDC of the 1.0-N and 2.0-N conditions.
Reliability of Measured Values
Thicknesses (mm) and Echo Intensity of the Rectus Femoris Muscle
Significant Differences of Mean Values Between Data, Using Bonferroni’s Test
Muscle Thickness | Echo Intensity | |||
---|---|---|---|---|
Differencea | P Value | Difference | P Value | |
0.5 N vs. | ||||
1.0 N | −0.6 | < 0.001 | −2.4 | 0.002 |
2.0 N | −2.0 | < 0.001 | −0.2 | 1 |
1.0 N vs. | ||||
0.5 N | 0.6 | < 0.001 | 2.4 | 0.002 |
2.0 N | −1.3 | < 0.001 | 2.2 | 0.019 |
2.0 N vs. | ||||
0.5 N | 1.9 | < 0.001 | 0.2 | 1 |
1.0 N | 1.3 | < 0.001 | −2.2 | 0.019 |
5. Discussion
To our knowledge, this is the first study to demonstrate the changes in muscle thickness and echo intensity of the RF induced by different inward pressures of a transducer during US. When using a technique that involves a handheld transducer, the examiner should attempt to maintain consistent inward pressure of the transducer during US to quantify the minimal change of the RF. In this study, muscle thickness was significantly decreased during increased inward pressures of the transducer among the three conditions. The differences in magnitude of muscle thickness might be meaningful between the 0.5-N and 2.0-N conditions to consider the MDC. Previous studies have shown changes in the transverse abdominis, internal oblique, and external oblique muscle thickness induced by different inward pressures of the transducer during US (7). Their results showed that differences of mean muscle thickness of the transverse abdominis, internal oblique, and external oblique muscles between the 0.5-N and 2.0-N conditions were −0.3, −0.6, and −1.2 mm, respectively (7). In this study, the difference of mean muscle thickness of the RF between 0.5-N and 2.0-N conditions was −2.0 mm. The elasticity of tissues between the target muscle and the transducer might influence the pressure applied to the target muscle during US (8), and a previous study showed that subcutaneous thickness at the thigh was thinner compared with the abdomen (11). One potential cause of these higher observed changes in the RF versus the abdominal muscles is a thinner layer of subcutaneous tissue between the RF and the transducer, compared with that of the abdominal muscles. Accordingly, the RF was sensitive to pressure, because there was reaction force from the femoral bone under the vastus intermedius. The elasticity of tissues under the target muscle might influence pressure on the target muscle during US (8); therefore, maintaining consistent transducer-induced inward pressure is required to clarify the minimal changes of muscle thickness induced by neuromuscular disease and aging in the RF.
On the other hand, echo intensity from the 0.5-N to 1.0-N condition was significantly decreased, and that from 1.0-N to 2.0-N condition was significantly increased in this study. These were major findings of this study. Previous studies have demonstrated that compression was observed to cause a flattening of muscle fiber cross-sections, aligning them at 90 degrees to the direction in which the deformation was applied (12). Skeletal muscle is composed of about 70 - 80% water, 3% fat, and 10% collagen (13), so muscle tissue might be considered as made of fluid-filled fiber fascicles surrounded by connective tissue, which constrains the flow of fluid, and the movement of the fluid component appears to be easier along the direction of the fibers than across their direction (14). When compression takes place in the cross-fiber direction, the fluid is free to rearrange itself, whereas during compression in the fiber direction, the fluid is further constrained by the endomysium and perimysium layer (14). In this study, the transducer was tilted and positioned to image clearly the epimysium of the RF before the measurements. However, it was impossible to position the transducer exactly at perpendicular to the longitudinal axis of all perimysium and endomysium of the RF. Therefore, light increased inward pressure of the transducer from the 0.5-N to 1.0-N condition might contribute to aligning the muscle fiber direction at 90 degrees to the direction in which the inward pressure was applied, and further increased inward pressure of the transducer from the 1.0-N to 2.0-N condition might contribute to flattening the muscle fibers’ cross-sections. The ultrasound image is created because of acoustic interfacing, wherein sound waves are reflected upon encountering a tissue of a different density. Perimysium and endomysium have different acoustical impedances, thereby aligning longitudinal muscle fascia direction at 90 degrees to the direction of ultrasound beam in the muscle, which might give the muscle a more hypoechoic appearance during inward pressure of the transducer from the 0.5-N to 1.0-N condition in this study. The amount of returning echoes per area determines the gray value of the image, therefore a flattening of muscle fibers’ cross-sections might give the muscle a more hyperechoic appearance during inward pressure of the transducer from the 1.0-N to 2.0-N condition. However, the differences in magnitude of echo intensity might be meaningless to consider the MDC.
This study had some limitations. The same investigator should not make all measurements of muscle thickness and echo intensity for blinding during the data collection. The direction and angle of the transducer are common variables related to margins of error in US procedures, and that should have been accounted for in the measurements taken in this study. Accordingly, muscle fiber directions are different among the muscles. Further study in several muscles is needed to determine the influence of different inward pressures of a transducer on muscle thickness and echo intensity. Another limitation of this study was the fact that only young males were recruited as subjects. The influence of gender on the influence of different inward pressures of the transducer on muscle thickness and echo intensity remains unknown. As part of the normative aging process, there is a decline in muscle thickness and an incline in echo intensity of the RF (1). Further study of a larger sample, including other age groups, is needed to determine the changes in muscle thickness and echo intensity induced by different inward pressures of a transducer during US.
Acknowledgements
References
-
1.
Pillen S, Arts IM, Zwarts MJ. Muscle ultrasound in neuromuscular disorders. Muscle Nerve. 2008;37(6):679-93. [PubMed ID: 18506712]. https://doi.org/10.1002/mus.21015.
-
2.
Dupont AC, Sauerbrei EE, Fenton PV, Shragge PC, Loeb GE, Richmond FJ. Real-time sonography to estimate muscle thickness: comparison with MRI and CT. J Clin Ultrasound. 2001;29(4):230-6. [PubMed ID: 11323778]. https://doi.org/10.1002/jcu.1025.
-
3.
Miyatani M, Kanehisa H, Ito M, Kawakami Y, Fukunaga T. The accuracy of volume estimates using ultrasound muscle thickness measurements in different muscle groups. Eur J Appl Physiol. 2004;91(2-3):264-72. [PubMed ID: 14569399]. https://doi.org/10.1007/s00421-003-0974-4.
-
4.
Reimers K, Reimers CD, Wagner S, Paetzke I, Pongratz DE. Skeletal muscle sonography: a correlative study of echogenicity and morphology. J Ultrasound Med. 1993;12(2):73-7. [PubMed ID: 8468739].
-
5.
Pillen S, Tak RO, Zwarts MJ, Lammens MM, Verrijp KN, Arts IM, et al. Skeletal muscle ultrasound: correlation between fibrous tissue and echo intensity. Ultrasound Med Biol. 2009;35(3):443-6. [PubMed ID: 19081667]. https://doi.org/10.1016/j.ultrasmedbio.2008.09.016.
-
6.
Reimers CD, Kellner H. Muscle Ultrasound. In: Fleckenstein JL, Crues JV, Reimers CD, editors. Muscle Imaging in Health and Disease. New York, USA: Springer New York; 1996. p. 13-20. https://doi.org/10.1007/978-1-4612-2314-6_2.
-
7.
Ishida H, Watanabe S. Influence of inward pressure of the transducer on lateral abdominal muscle thickness during ultrasound imaging. J Orthop Sports Phys Ther. 2012;42(9):815-8. [PubMed ID: 22517245]. https://doi.org/10.2519/jospt.2012.4064.
-
8.
Ishida H, Watanabe S. Influence of inward pressure applied by the transducer on trunk muscle thickness during ultrasound imaging. Kawasaki j med welfare. 2014;19(2):32-7.
-
9.
Cadore EL, Izquierdo M, Conceicao M, Radaelli R, Pinto RS, Baroni BM, et al. Echo intensity is associated with skeletal muscle power and cardiovascular performance in elderly men. Exp Gerontol. 2012;47(6):473-8. [PubMed ID: 22525196]. https://doi.org/10.1016/j.exger.2012.04.002.
-
10.
Weir JP. Quantifying test-retest reliability using the intraclass correlation coefficient and the SEM. J Strength Cond Res. 2005;19(1):231-40. [PubMed ID: 15705040]. https://doi.org/10.1519/15184.1.
-
11.
Eston R, Evans R, Fu F. Estimation of body composition in Chinese and British men by ultrasonographic assessment of segmental adipose tissue volume. Br J Sports Med. 1994;28(1):9-13. [PubMed ID: 8044501].
-
12.
Takaza M, Cooney GM, McManus G, Stafford P, Simms CK. Assessing the microstructural response to applied deformation in porcine passive skeletal muscle. J Mech Behav Biomed Mater. 2014;40:115-26. [PubMed ID: 25222870]. https://doi.org/10.1016/j.jmbbm.2014.08.019.
-
13.
Vignos PJ, Lefkowitz M. A biochemical study of certain skeletal muscle constituents in human progressive muscular dystrophy. J Clin Invest. 1959;38(6):873-81. [PubMed ID: 13654523]. https://doi.org/10.1172/JCI103869.
-
14.
Van Loocke M, Lyons CG, Simms CK. Viscoelastic properties of passive skeletal muscle in compression: stress-relaxation behaviour and constitutive modelling. J Biomech. 2008;41(7):1555-66. [PubMed ID: 18396290]. https://doi.org/10.1016/j.jbiomech.2008.02.007.