Abstract
Introduction:
The prognosis of recurrent pancreatic cancer is poor even after curative resection. There have been no reports of MRI-guided radiation therapy for locally recurrent pancreatic cancer after curative resection and chemotherapy.Case Presentation:
A 66-year-old man with pancreatic cancer was referred to our institution for local recurrence after failure of surgical resection and second-line chemotherapy. He did not want to undergo further chemotherapy, so high-dose MRI-guided adaptive radiation therapy was performed in daily doses of 2.5 Gy to a total dose of 70 Gy over a period of 5.5 weeks. Three months after radiation therapy, the recurrent tumors disappeared and his CA19-9 level was within normal range without chemotherapy. There were no adverse events during treatment and three months of follow-up.Conclusions:
High-dose MRI-guided adaptive radiation therapy may be safe and useful for locally recurrent pancreatic cancer.Keywords
1. Introduction
Despite advances in chemotherapy and surgery, the prognosis of pancreatic ductal adenocarcinoma (PDAC) remains poor even after curative resection. Multiagent chemotherapy is usually offered for recurrent PDAC, but the standard treatment for patients with locally recurrent PDAC has not yet been established (1, 2). High-dose MRI-guided radiation therapy for locally advanced PDAC has been suggested to improve overall survival and local control, relative to patients receiving lower radiation doses without increasing the rate of serious gastrointestinal toxicity (3). There have been no reports of MRI-guided radiation therapy for local recurrence after curative surgery and chemotherapy for PDAC. Here, we report a case of locally recurrent PDAC treated with high-dose fractionated MRI-guided adaptive radiation therapy.
2. Case Presentation
A 66-year-old man with a 4-year history of PDAC was referred to our institution for local recurrence after failure of surgical resection and second-line chemotherapy. The patient underwent pancreaticoduodenectomy and segmental resection of the superior mesenteric vein for PDAC in the head of the pancreas four years ago (pT4N1M0, AJCC 7e). The surgical margins were considered to be microscopically positive, and single-agent gemcitabine was administered for six months after surgery. He was subsequently followed up with no further treatment upon the patient’s request, but two years after surgery, his serum CA19-9 levels increased gradually (50 - 60 U/mL, normal: < 37 U/mL) and chemotherapy with S-1 was initiated. His CA19-9 levels decreased and remained at around 50 U/mL, but gradually increased to 111.3 U/mL two years after the start of chemotherapy with S-1. Whole-body contrast-enhanced CT showed no distant metastasis, but soft tissue lesions appeared in the tumor bed and around the superior mesenteric artery, infiltrating the mesenteric and para-aortic regions (Figure 1A). The tumors near the superior mesenteric artery were hyperintense relative to the adjacent muscles on T2-weighted MRI (Figure 1B), while diffusion-weighted image (DWI) showed multiple hyperintense lesions in the tumor bed and along the superior mesenteric artery (Figure 1C). These lesions were diagnosed as local recurrence, as they could not be detected by repeated postoperative CT and MRI. Endoscopic biopsy of the tumors near the superior mesenteric artery was considered technically difficult.
CT and MRI findings of locally recurrent pancreatic cancer. A, Contrast-enhanced CT scan showed tumor lesions surrounding the superior mesenteric artery (arrow), which infiltrated the mesentery and the para-aortic region. B, The recurrent lesion (arrow) was hyperintense relative to the adjacent muscles on T2-weighted MRI. C, Diffusion-weighted image obtained with b factor of 800 s/mm2 showed multiple nodules (arrows) with high signal intensity compared with the spinal cord. D, Three months after radiation therapy, there was no hyperintense tumor in the tumor bed and around the superior mesenteric artery on T2-weighted MRI (arrow). E, Three months after radiation therapy, the multiple hyperintense nodules on diffusion-weighted image disappeared (arrows).
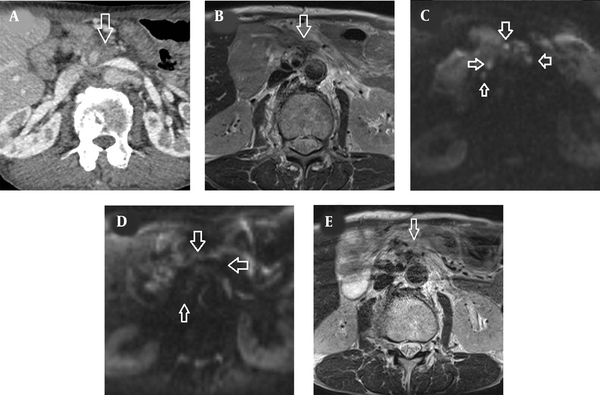
The patient was suggested combination chemotherapy, but he did not agree. After the protocol was approved by the institutional review board, he was treated with MRI-guided adaptive radiation therapy. Written informed consent was signed by the patient. All procedures were carried out in accordance with the ethical standards of Helsinki Declaration and its later amendments or comparable ethical standards. Since the chemotherapy with S-1 failed to control the recurrent tumor, the chemotherapy was discontinued. As adaptive two-step intensity modulated radiation therapy (IMRT), true fast imaging with steady precession (FISP) MRI was performed before MRI-guided IMRT for the initial plan of 40 Gy/16 fractions and during treatment for the boost plan of 30 Gy/12 fractions with a total dose of 70 Gy.
2.1. Simulation and Treatment Planning
The patient underwent both CT and MRI simulation using a 64-row multidetector CT scanner (Discovery CT750 HD, GE, Chicago, IL, USA) and a 3T MRI unit (MAGNETOM Skyra, Siemens Healthcare, Erlangen, Germany), respectively. We used a Vac-Lok cushion as an immobilization system. Because the MRI-guided radiotherapy system can simultaneously track the tumor and surrounding normal tissue during irradiation, no respiratory control device was used. CT simulation was carried out using non-contrast and contrast-enhanced axial CT images reconstructed to 2.5 mm slice thickness. MRI simulation was also carried out using axial two-dimensional turbo spin-echo T2-weighted MRI and DWI with a b-value of 800 s/mm2, both of which were reconstructed to 2.5 mm slice thickness. The patient also underwent MRI simulation on 0.35T MRI-guided radiotherapy system (MRIdian® System, ViewRayTM Inc, Oakwood Village, Ohio, USA) with surface coils on the abdomen using true FISP images. 0.35T MRI was taken at the same position as when CT and 3T MRI were taken. Fusion images of CT, 3T and 0.35T MRI were generated using Monaco 5.0 treatment planning software (Elekta AB, Stockholm, Sweden) to determine gross tumor volume (GTV). In fact, GTV was defined as macroscopic tumor lesions detected by contrast-enhanced CT and all MRI pulse sequences. Planning target volume (PTV) in the initial radiotherapy plan (PTV1) was defined as the volume containing the tumor bed, the lymph node region from the celiac artery to the upper edge of the horizontal part of the duodenum, and the infiltrates around the superior mesenteric artery. The prescribed dose to the D95% of the PTV1 (the dose covered 95% of the PTV1) was 40 Gy in 16 fractions (Figure 2A). For simulation of offline adaptive re-planning during radiation therapy course, PTV in the adaptive plan (PTV2) was generated in the true FISP images acquired after the 3rd week of treatment. PTV2 was defined as the GTV with 3-mm margin all around. The prescribed dose to the D95% of the PTV2 (the dose covered 95% of the PTV2) was 30 Gy in 12 fractions (Figure 2B). Treatment plan was performed using IMRT five days a week. The patient was treated with real-time 0.35T MRI-guided radiotherapy linac system (MRIdian® System, ViewRayTM Inc, Oakwood Village, Ohio, USA). At each treatment session, true FISP MRI in the treatment position was taken and the image was registered by the attending radiation oncologists and/or radiation technologists.
MRI-guided radiation therapy isodose lines with corresponding actual radiation dose. A, The initial plan. B, The adaptive boost plan.
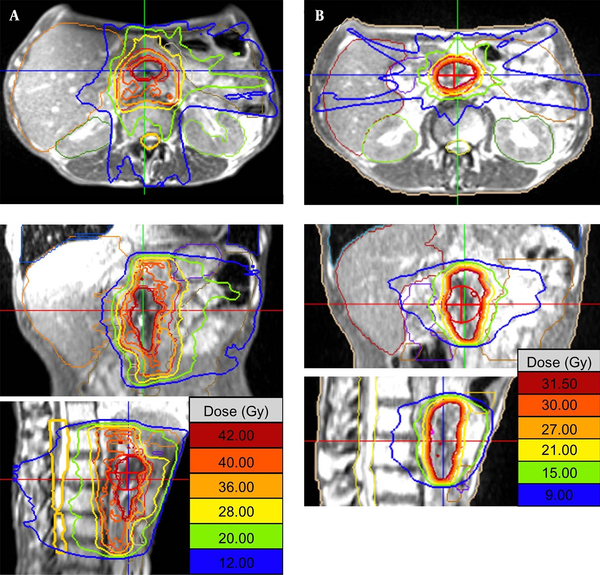
2.2. Short-term Treatment Outcome
The patient received the planned radiation therapy as scheduled. There were no radiation-induced adverse events during treatment and three months of follow-up. On MRI, all recurrent tumors disappeared three months after MRI-guided radiation therapy (Figure 1D-E). His CA19-9 level decreased to 29.3 U/mL (normal: < 37 U/mL) without chemotherapy. Based on the MRI findings and tumor marker values three months after radiation therapy, his recurrent PDAC was considered complete remission.
3. Discussion
Although the recurrence rate of PDAC after curative resection is as high as 85%, optimal treatment for locoregional recurrence has not been established (4). Systemic chemotherapy may be given to treat recurrent PDAC, but the median survival is usually less than one year (1, 2, 4). In this case, the recurrent PDAC was ineffective with second-line chemotherapy. We considered whether to choose the best supportive care or palliative radiation therapy. Since MRI-guided radiation therapy for locally advanced PDAC has been shown to improve overall survival and local control without increasing toxicity (3), we thought that it could be applied to local recurrence. KRAS G12C inhibitors may have helped to some extent to enhance the therapeutic effect, but KRAS G12C mutation was not detected in this case (5). Another treatment option is immunotherapy using immune checkpoint inhibitors such as pembrolizumab (6). However, the anti-PD-1 antibody pembrolizumab was not indicated for this patient because the tumor was microsatellite instability-low PDAC.
MRI-guided radiation therapy has enabled us to observe daily changes in the patient’s internal anatomy and to deliver high-dose radiation to the target while minimizing dose to the surrounding normal tissues. Since chemotherapy does not cure recurrent PDAC, high-dose MRI-guided radiation therapy might be considered an alternative option for treatment. If the tumor shrinks or the shape or position of the stomach and intestine changes, the use of adaptive planning may allow for more accurate radiation therapy (7). The previous study suggested that MRI-guided daily online adaptive radiation therapy would decrease the dose to organs at risk and improve target coverage in patients with pancreatic cancer (8). However, there is no evidence for the efficacy and safety of MRI-guided adaptive radiation therapy for postoperative local recurrence. Since there are some pitfalls regarding safety in daily online adaptive radiation therapy (8), and quality assurance and quality control for IMRT could not be performed, planned adaptive radiation therapy was chosen in this case. While the efficacy of high-dose MRI-guided hypofractionated radiation therapy for locally advanced pancreatic cancer has been shown (3, 7, 8), the optimal protocol for the total dose and fractions of radiation for local recurrence has not yet been established.
There are several limitations in this study that could be addressed. First, this is a single case study and it cannot be generalized to others without further scientific verifications. However, considering that MRI-guided adaptive radiation therapy is a new treatment method and has been shown to be effective for unresectable pancreatic cancer, it is important to try to expand its indications to include postoperative local recurrence. Based on previous reports and the results of this case, the most appropriate case for MRI-guided adaptive radiation therapy would be local recurrence without distant metastasis (2-4). Secondly, the follow-up period is short, and its long-term safety and efficacy are unknown. But, the purpose of this study was to present a proof of principle of high-dose MRI-guided adaptive radiation therapy for locally recurrent pancreatic cancer. Large-scale prospective studies will be needed to demonstrate safety and efficacy.
In conclusion, high-dose MRI-guided planned adaptive radiation therapy may be safe and useful for locally recurrent pancreatic cancer. Prospective evaluation of MRI-guided radiation therapy regimens with optimal treatment parameters is warranted.
References
-
1.
Gbolahan OB, Tong Y, Sehdev A, O'Neil B, Shahda S. Overall survival of patients with recurrent pancreatic cancer treated with systemic therapy: a retrospective study. BMC Cancer. 2019;19(1):468. [PubMed ID: 31101022]. [PubMed Central ID: PMC6525379]. https://doi.org/10.1186/s12885-019-5630-4.
-
2.
Serafini S, Sperti C, Friziero A, Brazzale AR, Buratin A, Ponzoni A, et al. Systematic Review and Meta-Analysis of Surgical Treatment for Isolated Local Recurrence of Pancreatic Cancer. Cancers (Basel). 2021;13(6). [PubMed ID: 33805716]. [PubMed Central ID: PMC7998253]. https://doi.org/10.3390/cancers13061277.
-
3.
Heerkens HD, van Vulpen M, Erickson B, Reerink O, Intven MP, van den Berg CA, et al. MRI guided stereotactic radiotherapy for locally advanced pancreatic cancer. Br J Radiol. 2018;91(1091):20170563. [PubMed ID: 30063383]. [PubMed Central ID: PMC6475956]. https://doi.org/10.1259/bjr.20170563.
-
4.
Lee JC, Ahn S, Cho IK, Lee J, Kim J, Hwang JH. Management of recurrent pancreatic cancer after surgical resection: a protocol for systematic review, evidence mapping and meta-analysis. BMJ Open. 2018;8(4). e017249. [PubMed ID: 29632079]. [PubMed Central ID: PMC5892773]. https://doi.org/10.1136/bmjopen-2017-017249.
-
5.
Kwan AK, Piazza GA, Keeton AB, Leite CA. The path to the clinic: a comprehensive review on direct KRAS(G12C) inhibitors. J Exp Clin Cancer Res. 2022;41(1):27. [PubMed ID: 35045886]. [PubMed Central ID: PMC8767686]. https://doi.org/10.1186/s13046-021-02225-w.
-
6.
Nevala-Plagemann C, Hidalgo M, Garrido-Laguna I. From state-of-the-art treatments to novel therapies for advanced-stage pancreatic cancer. Nat Rev Clin Oncol. 2020;17(2):108-23. [PubMed ID: 31705130]. https://doi.org/10.1038/s41571-019-0281-6.
-
7.
Boldrini L, Cusumano D, Cellini F, Azario L, Mattiucci GC, Valentini V. Online adaptive magnetic resonance guided radiotherapy for pancreatic cancer: state of the art, pearls and pitfalls. Radiat Oncol. 2019;14(1):71. [PubMed ID: 31036034]. [PubMed Central ID: PMC6489212]. https://doi.org/10.1186/s13014-019-1275-3.
-
8.
El-Bared N, Portelance L, Spieler BO, Kwon D, Padgett KR, Brown KM, et al. Dosimetric Benefits and Practical Pitfalls of Daily Online Adaptive MRI-Guided Stereotactic Radiation Therapy for Pancreatic Cancer. Pract Radiat Oncol. 2019;9(1):e46-54. [PubMed ID: 30149192]. https://doi.org/10.1016/j.prro.2018.08.010.