Abstract
Background:
Recent studies suggest that prolonged and intermittent bilateral common carotid artery occlusion (prolonged bilateral common carotid artery occlusion (PO) and intermittent bilateral common carotid artery occlusion, respectively) can lead to reduced ischemia brain injury in ischemic tolerance.Objectives:
In this study, we aimed to investigate the changes in brain lipidomics following prolonged and intermittent ischemic preconditioning.Materials and Methods:
Two groups underwent bilateral common carotid artery occlusion either intermittently (for 3 continuous periods within a timeframe of 20 minutes, releasing 3 consecutive times, yielding a total of 9 minutes (intermittent bilateral common carotid artery occlusion)) or for 9 minutes continuously (prolonged bilateral common carotid artery occlusion). The third and fourth groups were used as control and sham (without ischemia) groups, respectively. The first three groups were subdivided into middle cerebral artery occlusion-operated (middle cerebral artery occlusion MCAO), for 60 minutes of ischemia) and intact (without any surgery) subgroups. After 1 hour of ischemia and 24 hours of reperfusion, the neurologic deficit score (neurological deficit score (NDS)) and the infarct volume (infarct volume) were assessed in the middle cerebral artery occlusion-operated subgroup. Brain lipidomics were measured in the intact subgroup and the sham group.Results:
Preconditioning with prolonged bilateral common carotid artery occlusion and intermittent bilateral common carotid artery occlusion significantly decreased the neurological deficit scores and infarct volume and increased the levels of phosphatidylethanolamine, sphingomyelin, cholesterol ester, cholesterol, phosphatidylcholine, and cerebroside in the brain compared with the control and sham groups. Prolonged bilateral common carotid artery occlusion and intermittent bilateral common carotid artery occlusion significantly decreased the brain ceramide and lyso-phosphatidylcholine levels. The triglyceride levels in both groups (intermittent bilateral common carotid artery occlusion and prolonged bilateral common carotid artery occlusion) did not change in comparison with the control and sham groups.Conclusions:
Although it seems that further studies are needed to clarify the real mechanisms of ischemic tolerance, ischemic preconditioning could decrease brain ischemia injury via changes in brain lipidomics.Keywords
Preconditioning Stroke Brain Ischemia Cell Membrane Lipids Neuroprotective Common Carotid Artery Occlusion
1. Background
Ischemic preconditioning (IPC) (3 minutes) provides the maximal neuroprotection when induced 2 days prior to a lethal ischemic of a 60-minute duration (1, 2). When bilateral common carotid arteries are closed, tolerance can be induced. If IPC acts 24 hours before a lethal ischemia, sub-lethal ischemia, the most protection is afforded. This protective effect is maintained for at least 8 days. Transient ischemic and 3-nitropropionic acid protects the brain by reducing neurological deficits, the amount of tissue damage, the blood-brain barrier (BBB), and cerebral edema (3). If the brain is subjected to intermittent focal ischemia, intermittent global ischemia (4), and hypoxia (5), it may be resistant to subsequent ischemia. Ischemic tolerance induced by the activation of ATP-dependent potassium channels coupled with NMDA receptors (6) or adenosine receptors (7) during IPC is important. Among the primery cellular events that have occured following ischemia, the reduction of ATP stores, the production of reactive oxygen species (ROS), and an intracellular Ca2+ overload have been known to be critical for cells. Increases in intracellular Ca2+ activate proteolytic and lipolytic enzymes resulting in membrane protein and lipid degradation associated with an aggregation of diacylglycerol, lysophospholipids (Lyso-PL), and free fatty acids. Moreover, cell membranes are major target sites of ROS, and lipid peroxidation events is thought to play a critical role in ROS-mediated membrane injury during post-ischemic reperfusion. The loss of ATP reserves suppresses phospholipids reformation, contributing to alterations of membrane phospholipids contents. Present cellular processes have been prone to affect membrane permeability and membrane physiology, such as synaptic transmission, ion transport, and enzyme activities. Alterations in phospholipids contents have been demonstrated in various studies using different experimental models of cerebral ischemia. However, the rate of lipid turnover and the pattern of lipid changes have been shown in Drgova et al. study (8). In this experiment, by studying brain lipidomics we showed that intermittent and prolonged IPC facilitates the process of lipid synthesis in the brain after a second ischemia.
2. Objectives
Our study was conducted to examine the effect of ischemic preconditioning on the brain’s lipid changes. In fact, in this experiment was performed to investigate the effect of prolonged and intermittent ischemic preconditioning on brain lipidome changes.
3. Materials and Methods
3.1. Animals
All steps of the experimental animal methods were approved by the ethics committee of the Shahid Beheshti University of Iran. Throughout the study, they were provided with water and standard rat food ad libitum. All animals were kept in a 12:12 hour of dark-light cycle at 23 ± 2°C in the same animal facility under the same conditions.
3.2. Experimental Protocol
Thirty male Sprague-Dawley (250 - 350 g) rats were randomly allocated four experimental and a sham group including, three groups that received an ischemic preconditioning (prolonged (PO) and intermittent (IO)), and control groups. Each main animal group was assigned into MCAO (middle cerebral artery occlusion)-operated (for assesments of mortality and mordidity rates, NDS (neurological deficit score), and IV; n = 5 for PO (prolonged bilateral common carotid artery occlusion) and IO (intermittent bilateral common carotid artery occlusion), n = 9 for control) and intact subgroups (for brain lipidomics assessment; n = 5 for PO and IO, n = 9 for control). After pretreatment, rats were then placed in normal room air, after which MCAO-operated subgroups underwent 60 minutes of middle cerebral artery occlusion (MCAO). After 24 hours, neurologic deficits and infarct volume were assessed. In each intact animal subgroup, except surgery, all procedures were similar to the first subgroups. Finally, 48 hours after preconditioning, prolonged and intermittent ischemic preconditioning and control subgroups were sacrificed for the measurement of brain lipidomics in the right hemisphere. In addition, other groups underwent surgery without MCAO, as the sham-operated groups (sham groups; n = 5).
3.3. Global Cerebral Ischemia
The surgical method for the occlusion of rat two-vessel (two common carotid vessels occlusion) was adapted from the surgical techniqe of Iwasaki et al. (9). The present surgical technique for the induction of forebrain ischemia was first proposed about 20 years ago (10). Briefly, under chloral hydrate (400 mg/kg) anesthesia (Merck co., Germany), a vertical midline-neck incision in neck skin was given. Then, common carotid arteries were visualized and isolated carefully from the vagosympathetic nerve. For IO ischemia induction, animal brains entered into ischemic periods by occluding the bilateral common carotid artery (BCCA) for 3 continuous minutes of each 20 minutes (interval) releasing for 3 consecutive times and reperfusion was allowed for 20 minutes by releasing the block (3 minutes × 3 times = 9 minutes. For the PO ischemia studies, the rat brains were made ischemic by occluding the BCCA for a duration of 9 minutes and reperfusion was allowed for 20 minutes. The body temperature of animals was controled at about 37°C during the microsurgry with the help of cooling and heating instruments (10, 11).
3.4. Focal Cerebral Ischemia
The animals were weighed and anesthetized with 400 mg/kg of chloral hydrate intraperitoneally (Merck, Germany). MCAO was done as described by Longa et al. (12). Briefly, during a micsurgical procedure, a 3- 0 silicone coated nylon suture was introduced via the external carotid artery stump. The occluder was extended into the internal carotid artery 20 to 22 mm from the carotid bifurcation. The mild resistance of the nylon suture extention indicated that the blood flow in the middle cerebral artery was blocked. Reperfusion was intiated by withdrawing the suture after 60 minutes of ischemia. The rectal temperature was controlled (Geratherm color, Germany) at 37°C.
3.5. Neurobehavioral Evaluation
After withdrawing the nylon suture, the animals were returned to their isolated cages. Then, 24 hours after reperfusion, the animals were measured neurologically by an observer who was blind to the grouping. The neurologic deficit scoring was done using a six-point scale, as was previously presented by Longa et al. (12): normal neurologic function = 0; flexion of the contralateral torso and forelimb = 1; circling to the contralateral side =2; loss of the righting reflex (ability to regain footing from a back position) = 3; loss of motor activity and hypokinesia = 4. If the rats died due to asphyxia, pulmonary insufficiency, and subarachnoid hemorrhage, they were removed from the study.
3.6. Infarct Volume Assessment
The animals of the MCAO-operated subgroup were sacrificed with chloral hydrate (800 mg/kg) and then decapitated, and the rat brains were rapidly removed and maintained in 4°C saline for 15 minutes. Eight coronal whole brain slices of 2 mm were provided (Brain Matrix Co., Iran), strarting at the olfactory bulb. The brain sections were submerged in 2, 3, 5-triphenyltetrazolium chloride (TTC) solution 2% (Merck Co., Germany) and were maintained for 15 minutes at 37°C in a water bath. Then, the brain sections were photographed by a camera (DSC-W310, Japan) connected to a computer. Unstained areas were identified as injury tissue and were assessed using picture analysis software (Image Tools, NIH). The infarct or injury volume was measured by calculating the stained and unstained areas in each hemisphere section and multiplied by section thickness (2 mm), and then all 8 sections were summed based on the method used in (13): corrected IV = left hemisphere volume − (right hemisphere volume-IV).
3.7. Lipid Isolation, Purification, and Quantization
At 48 hours after pretreatment, the sham sham (without ischemia) and intact (without any surgery) animals were sacrificed by chloral hydrate (800 mg/kg) and decapitated for the assessment of lipid analysis. The penumbra, subcortex, and core of the brain tissue were removed according to previous methods (14). The core, penumbra, and subcortex were weighted and stored at -80°C. These areas were then lyophilized for lipid assessment. Lipids isolation and purification were done using perivious modifications procedures of lyophilized brain tissue (15, 16). The total content of lipids was extracted with 5 mL chloroform and methanol 1:1 by vol and 0.5 mL of dH2O. The present solution was mixed by a magnetic stirrer for at least 8 h at room temperature and then centrifuged for 10 minutes at 1200 × g. The supernatant was eliminated and the pellet was dissolved with 2 mL chloroform: methanol (1:1 by vol) and centrifuged as before. The supernatant was mixed and maintained at 4°C for the purification and isolation of lipid contents. The brain lipids were isolated by DEAE Sephadex column chromatography. The lipid extract dissolved in solvent A (chloroform: methanol: water, 30:60:8 by vol) was then used in a DEAE-Sephadex column that had been equilibrated with solvent A. The column was rinsed twice with 20 mL of solvent A, and then a fraction of neutral lipids was collected. The fraction of lipids contained Chol, CE, PC, Lyso-PC, PE, TG, SM, Cer, and CB.
3.8. High-Performance Thin-Layer Chromatography
The isolated lipids were assessed qualitatively using high-performance thin-layer chromatograph (HPTLC) based on previous methods. For better lipid solubility, a mixture of 200 µL of ethanol and 100 µL of chloroform was used, and then the upper phase was used for HPTLC analysis. A CAMAG TLC system composed of Linomat 5Auto sampler, winCATS 1.2.2 software (CAMAG Co., Switzerland), and TLC scanner 3 was used. Chromatography was done on TLC pre-coated silica gel 60 F254 plates 20 × 10 cm of (Merck Co., Germany). The sample solutions were applied to the HPTLC plates in 6mm length at 10 mm intervals under N2 stream using a Linomat 5 applicator. For neutral phospholipids, the plates were developed to a height of either 4.5 cm or 6 cm, respectively, with chloroform: methanol: acetic acid: formic acid: water (35:15:6:2:1 v/v), and then both were developed to 9 cm with hexanes: diisopropyl ether: acetic acid (65:35:2 v/v). Neutral phospholipids were detected using 3% cupric acetate in phosphoric acid (8%) solution and then heated in an oven at 165°C for 7 minutes as previously described technique. The density and distribution percentage of the individual bands was determined as done in previous methods. Briefly, the evaluation of samples was performed by scanning at λ 350 nm with a CAMAG TLC scanner III controlled by winCATS 1.2.2 software (16). The employed lipid methodology is capable of visulizing relatively slight differences in the brain’s lipid levels (less than 10%), as previously reported (14). Isolations of lipids were assessed based on their retention factor (Rf) using a HPTLC technique and comparing the previous methods laid out by (17). The resulting Rf value is insufficient for the identification of the compound, each spot was picked up from the plate and the predicted targets of compounds were investigated using the offline ESI/IT mass spectrometry.
3.9. ESI/IT Mass Spectrometry
As mentioned above, the extracted lipid was separated using the HPTLC method. However, the Rf value was not sufficient for the identification of compound on the plate. The identified fraction containing lipids was scarped from the plate and re-extracted for 5 minutes with (methanol: chloroform, 1:1 (v/v)) assisted by an ultrasound extraction method. The extracts were centrifugation for 5 minutes at 6000 g, and the supernatants were then analyzed using offline positive ESI/IT mass spectrometry (LCQ Thermofisher Scientific Co., Germany). The ESI/MS (Electrospray ionization-ion trap mass spectrometry) under the condition of 2.0 kV capillary voltage and 20 skimmer cone voltage was applied. In each analysis, the syringe speed was adjusted to 25 µL/minutes under the 60 mL/minutes of sheath gas and 20 mL/minutes of auxiliary gas. The capillary temperature and the discharge current were 250°C and 4.80 - 5.00 µA, respectively.
3.10. Blood Flow Laser Doppler
Blood flow and velocity assessment (MBF3D, Moor Instrument, Axminster, UK) was applied to measure the regional cerebral blood flow (rCBF). The related probe of a laser Doppler flowmeter was positioned using a stereotaxic device and a low-speed dental drill at the surface. Thus, a burr hole 2 mm in diameter was provided over the skull 5 mm lateral and 1 mm posterior to the bregma on the right side. A needle-shaped laser probe was located on the dura away from middle cerebral artery. Steady-state baselines of blood flow were monitored before MCAO, so that blood flow values were expressed as percentages of the respective basal value in all regional cerebral areas (18). Doppler flux was continuously assessed from -30 minutes MCAO until +30 minutes after reperfusion.
3.11. Statistical Analysis
Lipids including CE (cholesterol ester), Chol (cholesterol), TG (triglyceride), CB (cerebroside), Cer, PC (phosphatidylcholine), PE (phosphatidylethanolamine), SM (sphingomyelin), lyso-PC (lyso-phosphatidylcholine), rCBF (regional cerebral blood flow), and IV (infarct volume) levels were expressed as means ± SEM. One-way ANOVA (SPSS 16.0 post hoc LSD) was used to compare the means of various groups. NDS were expressed as the median (range) and analyzed using the Mann-Whitney U test. P < 0.05 was considered significant.
4. Results
4.1. Experimental Condition Parameters
Normal cerebral blood flow features varied under the IPC rats and did not differ from those of the control rats. In each studied group, the induction of middle cerebral artery ischemia reduced the mean cerebral blood flow to less than 23% of that at baseline (Figure 1).
Regional Cerebral Blood Flow (rCBF)
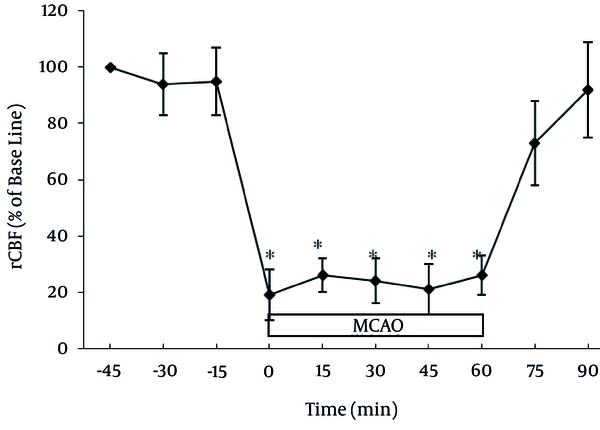
The Effect of Prolonged and Intermittent Common Carotid Artery Occlusion (IO; Black and PO; Gray) and Control (White) on Infarct Volume in the Total Area, Core, and Penumbra and Subcortex Areas in MCAO (O) Subgroups (n = 5)
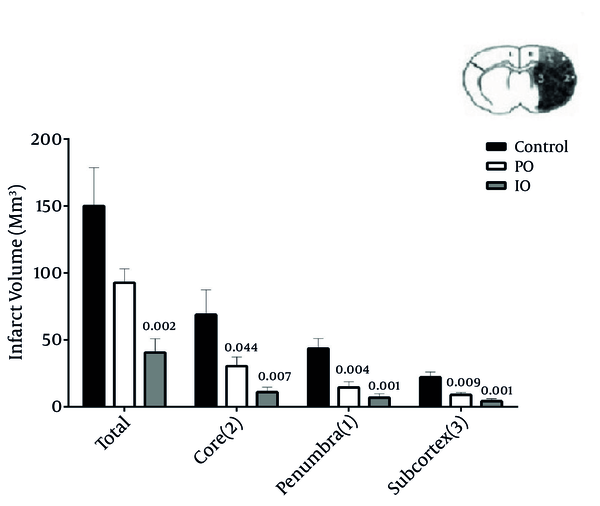
4.2. Effects of HO on Neurobehavioral Deficit Scores and Infarct Volume
The median neurologic deficit scores decreased by prolonged and intermittent ischemic preconditioning, and were 2 (range: 0 - 5), 1 (range: 0 - 2), and 2 (range: 2 - 4) in the PO, IO, and control groups, respectively (Table 1). The putative beneficial effects of prolonged and intermittent ischemic preconditioning were confirmed by a reduction in infarct volume. Pretreatment with prolonged and intermittent resulted in a reduction of infarct volume, while RA groups had no effect. The infarct volume in core (P = 0.007 and P = 0.044), penumbra (P = 0.001 and P = 0.004), and subcortex (P = 0.001 and P = 0.009) was significantly reduced in intermitent and prolonged IPC groups, respectively.
The Distribution of Neurologic Deficit Scores in Each Group
4.3. Effect of Prolonged and Intermittent Ischemic Preconditioning on Brain Lipidomics in the Core
The brain phosphatidylethanolamine (PE), sphingomyelin (SM), cholesterol ester (CE), phosphatidylcholine (PC), and cerebroside (CB) levels were greater in the prolonged common carotid artery occlusion group when than the control groups (sham and control). PO significantly reduced the brain cholesterol, ceramide, and lyso-phosphatidylcholine (LPC) levels in the prolonged ischemic preconditioning groups. The brain phosphatidylethanolamine, sphingomyelin, cholesterol ester, cholesterol, phosphatidylcholine, and cerebroside levels were greater in the intermittent common carotid artery occlusion group than the control groups (sham and control). IO significantly decreased the brain ceramide and lyso-phosphatidylcholine levels in the intermittent ischemic preconditioning groups. The triglyceride (TG) level in both groups (IO and PO) did not change in comparison with the control groups (sham and control) (Figure 3A) (Table 2).
Effect of Prolonged and Intermittent Ischemic Preconditioning in Brain Lipidomics Levels in the Core, Subcortex and Penumbra Regions; Cholesterol Ester (CE), Triglyceride (TG), Lyso-Phosphatidylcholine (LPC), Phosphatidylethanolamine (PE) Phosphatidylcholine (PC), Ceramide (CER), Cerebroside (CB), Cholesterol (CHOL), Sphingomyelin(SM) (*P < 0.05, n = 5).
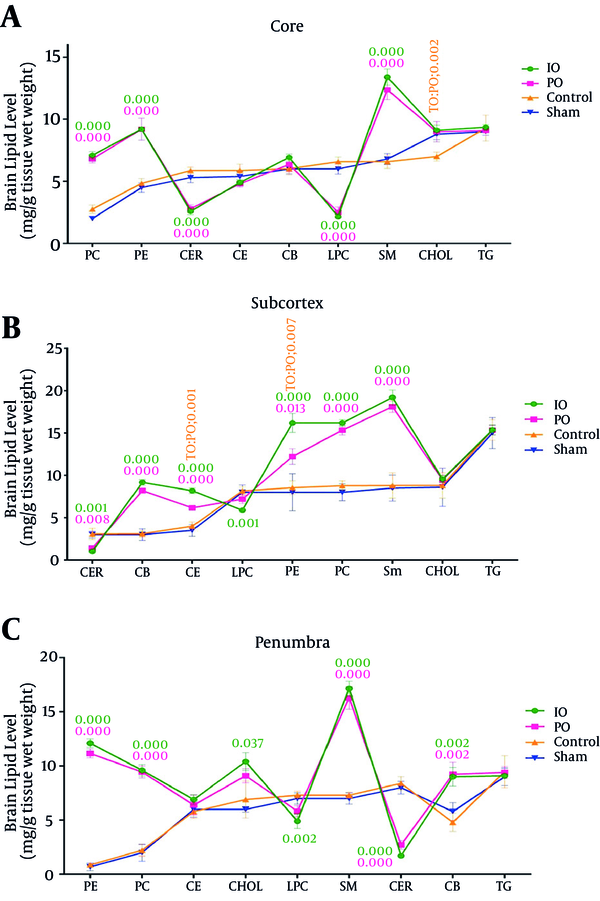
Effect of Prolonged (PO) and Intermittent (IO) Ischemic Preconditioning on Brain Lipidomics in the Core, Subcortex, and Penumbra Regionsa
Groups and Brain Regions | PE | SM | CE | PC | Chol | CB | Lyso- PC | TG | Cer |
---|---|---|---|---|---|---|---|---|---|
PO | |||||||||
core | < 0.000 | < 0.000 | NA | < 0.000 | 0.002 | NA | < 0.000 | NA | < 0.000 |
sub | < 0.000 | < 0.000 | < 0.000 | P < 0.000 | NA | < 0.000 | NA | NA | 0.008 |
pen | < 0.000 | < 0.000 | NA | < 0.000 | NA | 0.002 | NA | NA | < 0.000 |
IO | |||||||||
core | < 0.000 | < 0.000 | NA | < 0.000 | 0.002 | NA | < 0.000 | NA | < 0.000 |
sub | < 0.000 | < 0.000 | < 0.000 | < 0.000 | NA | < 0.000 | 0.001 | NA | 0.001 |
pen | < 0.000 | < 0.000 | NA | < 0.000 | 0.037 | 0.002 | 0.002 | NA | 0.000 |
4.4. Effect of Prolonged and Intermittent Ischemic Preconditioning on Brain Lipidomics in the Subcortex
The brain phosphatidylethanolamine, sphingomyelin, cholesterol ester, cholesterol, phosphatidylcholine, and cerebroside levels were greater in the prolonged common carotid artery occlusion group than the control groups (sham and control). PO significantly decreased the brain ceramide and lyso-phosphatidylcholine levels in the prolonged ischemic preconditioning groups. The brain phosphatidylethanolamine, sphingomyelin, cholesterol ester, cholesterol, phosphatidylcholine, and cerebroside levels were greater in the intermittent common carotid artery occlusion group than the control group (sham and control). IO significantly decreased the brain ceramide and lyso-phosphatidylcholine levels in the intermittent ischemic preconditioning groups. The triglyceride level in both groups (IO and PO) did not change in comparison with the control groups (sham and control) (Figure 3B) (Table 2).
4.5. Effect of Prolonged and Intermittent Ischemic Preconditioning on Brain Lipidomics in the Penumbra
The brain phosphatidylethanolamine, sphingomyelin, cholesterol ester, cholesterol, phosphatidylcholine and cerebroside levels were greater in the prolonged common carotid artery occlusion group than the control groups (sham and control). PO significantly decreased the brain ceramide and lyso-phosphatidylcholine levels in the prolonged ischemic preconditioning groups. The brain phosphatidylethanolamine, sphingomyelin, cholesterol ester, cholesterol, phosphatidylcholine, and cerebroside levels were greater in the intermittent common carotid artery occlusion group than the control groups (sham and control). IO significantly decreased the brain ceramide and lyso-phosphatidylcholine levels in the intermittent ischemic preconditioning groups. The triglyceride level in both groups (IO and PO) did not change in comparison with the control groups (sham and control) (Figure 3C) (Table 2).
4.6. Comparison of the Effect of Prolonged and Intermittent Ischemic Preconditioning in the Right Hemisphere
In the right hemisphere, the levels of all lipids increased more in the IO group than in the PO group, except for ceramid and lyso-phosphatidylcholine. The triglyceride levels remain the same in both the IO and PO groups (Figure 4).
Comparison of Lipids in the Right Hemisphere of the Brain (Core, Subcortex, and Penumbra); Cholesterol Ester (CE), Triglyceride (TG), Lyso-Phosphatidylcholine (LPC), Phosphatidylethanolamine (PE) Phosphatidylcholine (PC), Ceramide (CER), Cerebroside (CB), Cholesterol (CHOL), Sphingomyelin (SM), (*P < 0.05, n = 5).
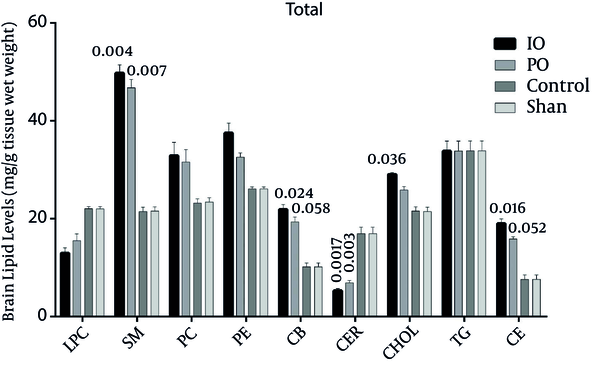
4.7. ESI/IT Mass Spectrometry
As mentioned above, isolations of lipids were detected their retention factor (Rf), using the HPTLC method by comparing the previous reports by (16) (Figure 5). On the other hand, the Rf value is not sufficient for the detection of compounds on the TLC plate; as a result, each spot was picked up from the plate and the predicted targets of compounds were investigated using offline mass spectrometry. The molecular weight of various lipids in the rat brain samples is shown in our previous study (19).
HPTLC of the Core, Subcortex, and Penumbra Brain Lipids in the Rats Pretreated With IO and PO
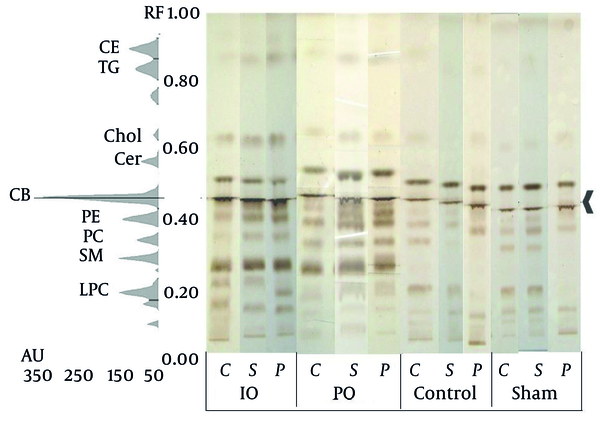
5. Discussion
The present study was the first to examine the effects of prolonged and intermittent bilateral common carotid artery occlusion on the brain lipidome in a focal cerebral ischemia animal model. The findings indicate that IV, NDS, and the brain Cer and lyso-pc levels might be lessened by a precondition with bilateral common carotid artery occlusion in an accurate animal model of a stroke followed by reperfusion.
The results demonstrated that the exposure of the brain to 9 minutes (PO) and 3 minutes (3 times) (IO) (between each occlusion, reperfusion was performed for 20 seconds) cerebral ischemia using the two-vessel occlusion technique to induce global intermittent ischemia provides strong neuroprotection against ischemic neuronal damage produced by a subsequent 60-minutes period of ischemia. It has been shown that if the brain experiences 3 minutes of cerebral ischemia in the two-vessel occlusion model of global intermittent ischemia, it is supplied with a dominant protection against ischemic neuronal damage caused by a subsequent 6- to 15-minutes period of ischemia (2). It has been reported that a precondition with a 3-minutes sub-lethal ischemia protects the rat hippocampus against subsequent deadly ischemic neuronal damage induced by a 6-minutes ischemia (7).
In our studies, ischemia inducted 3 times for 3 minutes led to greater protection than 9 minutes of ischemia. The results of the comparison of these two preconditioners on brain lipid levels after the induction of focal ischemia showed that in all three brain regions, the levels of all the lipids increased in the IO group more than in the PO group, except for ceramide and lyso-phosphatidylcholine. The triglyceride levels remained unchanged in both the IO and PO group. It has been reported that 1 to 2 minutes of ischemia is not sufficient to create tolerance, but 3 minutes provides protection (2).
Arachidonic acid and docosahexaenoic acid are free fatty acids that accumulate during focal cerebral ischemia, which is due to the activity of both phospholipase A2 and phospholipase C (20).
It has been reported that about 10 minutes after 30 minutes bilateral common carotid artery occlusion (BCCAO) increases the pool of free fatty acids in gerbil. Bralet et al. found that bilateral carotid artery occlusion in rats for 6 h led to a permanent increase in the FFA levels with a progressive deterioration of cerebral energy metabolism, suggesting a relationship between the two present processes (21).
This study demonstrates that preconditioning with intermittent and prolonged bilateral common carotid artery occlusion (PO) reduced the brain Cer and lyso-PC levels in comparison with the control and sham groups. Some selective reduction of specific phospholipids in a short period of ischemia was observed (21). It has been noted that sphingomyelinase is triggered by a performance factor called arachidonic acid for the production of ceramide. Ceramide can induce apoptosis via the inhibition of a mitochondrial electron transport chain and the release of cytochrome C. Caspase-3 is activated by cytochrome c, which can induce cell apoptosis (20). The high levels of ceramides in the brain may trigger inflammatory pathways in addition to negative effects in neurons in the aging process (22).
Our data suggest that the rise in brain PC levels caused by intermittent and prolonged bilateral common carotid artery occlusion mediated neuroprotection. The stroke model can lead to the activation of phospholipase, which will lead to a decrease in PC. Ischemia and reperfusion cause the failure of cytidylyltransferase phosphocholine activity, which is moderately corrected by cytidine-diphosphocholine. A deficiency in phosphocholine cytidylyltransferase can cause cell death by reducing the synthesis of PC (20). It has been demonstrated that post–transient MCAO neuronal injury is more hazardous in the subcortex than the cortex (23). It has also been reported that rat brains under extreme hypoxia conditions can experience an increase in free fatty acids. Moreover, two chief enzymes for the de novo synthesis of the PC in whole cell experiments on cardiomyocytes (i.e., choline kinas and CTP phosphocholine cytidylyltransferase) are inhibited by hypoxia as well as glucose deprivation. It has also been demonstrated that the choline kinase enzyme was restrained by acidosis in a cell-free experiment (24). Previous studies have shown that in the pH in the brains of stroke patients is significantly reduced (25).
In a BCCAO model, cerebral blood flow has been reported to decrease nearly 50%, whereas in a four-vessel occlusion model, it decreased by 95%. In BCCAO rats, at the level of the hippocampus, a number of customary neurochemical changes have been reported. In BCCAO rats, the formation of the inositol phosphate is increased, i.e., an effect that has also been shown to occur following ischemic injury. In addition, 7- aminobutyric acid (GABA) content is increased whereas the acetylcholine concentration is reduced (26). It has been shown that in preconditioned brains, protein formation improves following a second ischemia; i.e., it improves even quicker than after short ischemic preconditioning (27).
Our studies showed that preconditioning with intermittent and prolonged bilateral common carotid artery occlusion increased CB brain levels in comparison with the control and sham groups. A myelin sheath is chiefly composed of lipids named galactocerebrosides and sulphatides. It has been shown that cerebroside and the concentration of sulphatides decreases during ischemia, which decreases the intracellular pH in cells and thereby could lead to a release of lysosomal hydrolyses (28).
Preconditioning with intermittent and prolonged bilateral common carotid artery occlusion did not lead to different TG levels than the control groups. During ischemia and hypoxia, the TG turnover inhibition did not change in the myocardial TG level. However, it has been proposed that TG return is precipitated during ischemia and reperfusion (29). The brains of rats that were treated for 8 weeks with an olive oil diet (15%) showed higher levels of cholesterol and triglycerides than a control group (30).
IPC is a technique used to ensure the safety of tissues and organs against ischemia/reperfusion damage from decreasing ROS levels, lipid peroxidation, and apoptosis (31).
This paper examined the effect of common carotid artery occlusion on brain lipidomics, which is critical in the estimation of neuroprotection. In the training of both in vivo and in vitro organs, prior researchers have reported that the increase in the concentration of lipid peroxidation products was due to ischemia-reperfusion damage. It was also reported that oxygen free-radical production may cause lipid peroxidation to occur (32).
Our studies showed that preconditioning with intermittent and prolonged bilateral common carotid artery occlusion caused an increase in brain Chol and CE levels in comparison with the control and sham groups. Tight junctions are rich in cholesterol and are known as characteristics of the blood-brain barrier’s impermeability. The blood-brain barrier’s inability to maintain ionic gradients caused by reperfusion and ischemia has been associated with cerebral edema (33). During ischemia, oxidative stress can cause a variety of disturbances in cholesterol and sphingolipid metabolism. Recent studies have demonstrated that ceramide and oxidative stress can lead to an increase in cholesterol in non-neuronal cells. The inhibition of acyl- CoA -cholesterol acyl transferase (a converting enzyme that turns free cholesterol into cholesterol esters), which has the ability to stimulate apoptosis in addition to statins (or HMG-CoA reductase inhibitors) that are able to keep neurons away from ischemic oxidative injury, confirms the toxicity of increased levels of free cholesterol to cells (22). There is no confirmation of the gaping of the blood-brain barrier in BCCAO rats (34).
Our study shows that preconditioning with intermittent and prolonged bilateral common carotid artery occlusion reduced the brain lyso-PC levels in comparison with the control and sham groups. It has been reported that a decrease in phospholipids increased the amount of free fatty acids followed by phospholipase activation during the first day of reperfusion (35).
It has been reported that phospholipases are activated in neurons in several ways: 1) membrane damage through phospholipid deprivation, 2) increased calcium entry, and 3) the formation of lyso-PC that can be metabolized to platelet-activating factors (36).
It has been shown that PC homeostasis is controlled by the opposing effects of PCCT and the accumulation effects of PLA2, PC-PLC, and PC-PLD. It has been reported that Lyso-PC to overcome PCCT activity can lead to the disruption of PC synthesis. While the inhibition of PC synthesis is sufficient to result in cell death, a failure of PC may be a dangerous factor leading to neuronal damage (37).
Preconditioning with intermittent and prolonged bilateral common carotid artery occlusion can also increase the brain’s SM levels. Our study showed that treatment with intermittent and prolonged bilateral common carotid artery occlusion increased SM compared to the control and sham groups. It has been reported that the loss of SM was rapidly observed after an ischemia/ one-day reperfusion. It has also been shown that an increase of ROS during ischemia followed by increased tumor necrosis factor-α stimulates the sphingomyelinase enzyme (35).
It has been shown that the main source of cellular ceramide is provided by one of several sphingomyelinases that can hydrolyze the SM. Therefore, the selective hydrolysis of a mitochondrial collection of SM by overexpressed sphingomyelinase in mitochondria induces cellular apoptosis (38).
A histological analysis of the brain after ischemia suggested that myelin was relatively resistant to ischemic injury. Thus, the myelin structure is destroyed during the final stage of ischemic tissue damage (39).
Our experiments demonstrated that a rise in the brain’s PE levels can be achieved through preconditioning with intermittent and prolonged bilateral common carotid artery occlusion. It has been shown that a minor level of PE in Alzheimer disease might result in a decrease in total phospholipids (40). PE has also been reduced to 25 percent during prolonged ischemia (21).
In summary, our data suggest that a brain lipidome change in ischemic-reperfusion has an effect on the neuroprotection system and is therefore a critical entailment in stroke pathogenesis. In our studies, ischemia for 3 minutes, induced 3 times, leads to greater protection than 9 minutes of ischemia on the brain’s lipid levels. Further research is needed to expand on these findings. Finally, we hope to see further advancement in cerebro-protective strategies for those at risk of stroke or those whose cerebral perfusion might be decreased when the time of surgery comes.
References
-
1.
Kuzuya T, Hoshida S, Yamashita N, Fuji H, Oe H, Hori M, et al. Delayed effects of sublethal ischemia on the acquisition of tolerance to ischemia. Circ Res. 1993;72(6):1293-9. [PubMed ID: 8495557].
-
2.
Shamloo M, Wieloch T. Changes in protein tyrosine phosphorylation in the rat brain after cerebral ischemia in a model of ischemic tolerance. J Cereb Blood Flow Metab. 1999;19(2):173-83. [PubMed ID: 10027773]. https://doi.org/10.1097/00004647-199902000-00009.
-
3.
Bigdeli MR, Rahnama M. Comparison between preconditioning with temporary middle cerebral artery occlusion and 3-Nitropropionic Acid on reduction of brain injuries in rat stroke model. Trauma Mon. 2011;2011(2, Summer):95-100.
-
4.
Kitagawa K, Matsumoto M, Tagaya M, Hata R, Ueda H, Niinobe M, et al. ‘Ischemic tolerance’ phenomenon found in the brain. Brain Res. 1990;528(1):21-4. [PubMed ID: 2245337]. https://doi.org/10.1016/0006-8993(90)90189-i.
-
5.
Gorgias N, Maidatsi P, Tsolaki M, Alvanou A, Kiriazis G, Kaidoglou K, et al. Hypoxic pretreatment protects against neuronal damage of the rat hippocampus induced by severe hypoxia. Brain Res. 1996;714(1-2):215-25. [PubMed ID: 8861628].
-
6.
Kato H, Liu Y, Araki T, Kogure K. MK-801, but not anisomycin, inhibits the induction of tolerance to ischemia in the gerbil hippocampus. Neurosci Lett. 1992;139(1):118-21. [PubMed ID: 1407677].
-
7.
Heurteaux C, Lauritzen I, Widmann C, Lazdunski M. Essential role of adenosine, adenosine A1 receptors, and ATP-sensitive K+ channels in cerebral ischemic preconditioning. Proc Natl Acad Sci U S A. 1995;92(10):4666-70. [PubMed ID: 7753861].
-
8.
Drgova A, Likavcanova K, Dobrota D. Changes of phospholipid composition and superoxide dismutase activity during global brain ischemia and reperfusion in rats. Gen Physiol Biophys. 2004;23(3):337-46. [PubMed ID: 15638121].
-
9.
Iwasaki Y, Ito S, Suzuki M, Nagahori T, Yamamoto T, Konno H. Forebrain ischemia induced by temporary bilateral common carotid occlusion in normotensive rats. J Neurol Sci. 1989;90(2):155-65. [PubMed ID: 2723680].
-
10.
Eklof B, Siesjo BK. The effect of bilateral carotid artery ligation upon the blood flow and the energy state of the rat brain. Acta Physiol Scand. 1972;86(2):155-65. [PubMed ID: 4640167]. https://doi.org/10.1111/j.1748-1716.1972.tb05322.x.
-
11.
Yanpallewar SU, Hota D, Rai S, Kumar M, Acharya SB. Nimodipine attenuates biochemical, behavioral and histopathological alterations induced by acute transient and long-term bilateral common carotid occlusion in rats. Pharmacol Res. 2004;49(2):143-50. [PubMed ID: 14643694].
-
12.
Longa EZ, Weinstein PR, Carlson S, Cummins R. Reversible middle cerebral artery occlusion without craniectomy in rats. Stroke. 1989;20(1):84-91. [PubMed ID: 2643202].
-
13.
Swanson RA, Morton MT, Tsao-Wu G, Savalos RA, Davidson C, Sharp FR. A semiautomated method for measuring brain infarct volume. J Cereb Blood Flow Metab. 1990;10(2):290-3. [PubMed ID: 1689322]. https://doi.org/10.1038/jcbfm.1990.47.
-
14.
Lei B, Popp S, Capuano-Waters C, Cottrell JE, Kass IS. Lidocaine attenuates apoptosis in the ischemic penumbra and reduces infarct size after transient focal cerebral ischemia in rats. Neuroscience. 2004;125(3):691-701. [PubMed ID: 15099683]. https://doi.org/10.1016/j.neuroscience.2004.02.034.
-
15.
Seyfried TN, Heinecke KA, Mantis JG, Denny CA. Brain lipid analysis in mice with Rett syndrome. Neurochem Res. 2009;34(6):1057-65. [PubMed ID: 19002580]. https://doi.org/10.1007/s11064-008-9874-7.
-
16.
Macala LJ, Yu RK, Ando S. Analysis of brain lipids by high performance thin-layer chromatography and densitometry. J Lipid Res. 1983;24(9):1243-50. [PubMed ID: 6631248].
-
17.
Kasperzyk JL, El-Abbadi MM, Hauser EC, D'Azzo A, Platt FM, Seyfried TN. N-butyldeoxygalactonojirimycin reduces neonatal brain ganglioside content in a mouse model of GM1 gangliosidosis. J Neurochem. 2004;89(3):645-53. [PubMed ID: 15086521]. https://doi.org/10.1046/j.1471-4159.2004.02381.x.
-
18.
Chen S, Cheung RTF. Peripheral and central administration of neuropeptide Y in a rat middle cerebral artery occlusion stroke model reduces cerebral blood flow and increases infarct volume. Brain Res. 2002;927(2):138-43. [PubMed ID: 11821007]. https://doi.org/10.1016/s0006-8993(01)03336-4.
-
19.
Faezi M, Bigdeli MR, Mirzajani F, Ghasempour A. Normobaric Hyperoxia Preconditioning Induces Changes in the Brain Lipidome. Curr Neurovasc Res. 2015;12(4):353-62. [PubMed ID: 26285738].
-
20.
Adibhatla RM, HAtcher JF, Larsen EC, Chen X, Sun D, Tsao FH. Retraction: CDP-choline significantly restores phosphatidylcholine levels by differentially affecting phospholipase A2 and CTP: phosphocholine cytidylyltransferase after stroke. J Biol Chem. 2013;288(11):7549. [PubMed ID: 23505262]. https://doi.org/10.1074/jbc.A113.512112.
-
21.
Bralet J, Beley P, Jemaa R, Bralet AM, Beley A. Lipid metabolism, cerebral metabolic rate, and some related enzyme activities after brain infarction in rats. Stroke. 1987;18(2):418-25. [PubMed ID: 3564099].
-
22.
Cutler RG, Kelly J, Storie K, Pedersen WA, Tammara A, Hatanpaa K, et al. Involvement of oxidative stress-induced abnormalities in ceramide and cholesterol metabolism in brain aging and Alzheimer's disease. Proc Nat Acad Sci. 2004;101(7):2070-5. [PubMed ID: 14970312]. https://doi.org/10.1073/pnas.0305799101.
-
23.
Garcia-Ruiz C, Colell A, Mari M, Morales A, Fernandez-Checa JC. Direct effect of ceramide on the mitochondrial electron transport chain leads to generation of reactive oxygen species. Role of mitochondrial glutathione. J Biol Chem. 1997;272(17):11369-77. [PubMed ID: 9111045].
-
24.
Schwarz E, Prabakaran S, Whitfield P, Major H, Leweke FM, Koethe D, et al. High throughput lipidomic profiling of schizophrenia and bipolar disorder brain tissue reveals alterations of free fatty acids, phosphatidylcholines, and ceramides. J Proteome Res. 2008;7(10):4266-77. [PubMed ID: 18778095]. https://doi.org/10.1021/pr800188y.
-
25.
Bigdeli MR. Neuroprotection caused by hyperoxia preconditioning in animal stroke models. ScientificWorldJournal. 2011;11:403-21. [PubMed ID: 21336456]. https://doi.org/10.1100/tsw.2011.23.
-
26.
Jaspers RMA, Block F, Heim C, Sontag KH. Spatial learning is affected by transient occlusion of common carotid arteries (2VO): Comparison of behavioural and histopathological changes after ‘2VO’ and ‘four-vessel-occlusion’ in rats. Neurosci Let. 1990;117(1-2):149-53. [PubMed ID: 2290611]. https://doi.org/10.1016/0304-3940(90)90135-v.
-
27.
Kato H, Kogure K, Nakata N, Araki T, Itoyama Y. Facilitated recovery from postischemic suppression of protein synthesis in the gerbil brain with ischemic tolerance. Brain Res Bul. 1995;36(2):205-8. https://doi.org/10.1016/0361-9230(94)00191-3.
-
28.
Chavko M, Nemoto EM. Regional differences in rat brain lipids during global ischemia. Stroke. 1992;23(7):1000-4. [PubMed ID: 1615531].
-
29.
Saddik M, Lopaschuk GD. Myocardial triglyceride turnover during reperfusion of isolated rat hearts subjected to a transient period of global ischemia. J Biol Chem. 1992;267(6):3825-31. [PubMed ID: 1740430].
-
30.
Kurban S, Mehmetoglu I, Yilmaz G. Effect of diet oils on lipid levels of the brain of rats. Indian J Clin Biochem. 2007;22(2):44-7. [PubMed ID: 23105681]. https://doi.org/10.1007/BF02913312.
-
31.
Olguner CG, Koca U, Altekin E, Ergur BU, Duru S, Girgin P, et al. Ischemic preconditioning attenuates lipid peroxidation and apoptosis in the cecal ligation and puncture model of sepsis. Exp Ther Med. 2013;5(6):1581-8. [PubMed ID: 23837035]. https://doi.org/10.3892/etm.2013.1034.
-
32.
Fraga CG, Leibovitz BE, Tappel AL. Lipid peroxidation measured as thiobarbituric acid-reactive substances in tissue slices: characterization and comparison with homogenates and microsomes. Free Radic Biol Med. 1988;4(3):155-61. [PubMed ID: 3356355].
-
33.
Huber JD, Egleton RD, Davis TP. Molecular physiology and pathophysiology of tight junctions in the blood-brain barrier. Trends Neurosci. 2001;24(12):719-25. [PubMed ID: 11718877].
-
34.
Farkas E, Luiten PG, Bari F. Permanent, bilateral common carotid artery occlusion in the rat: a model for chronic cerebral hypoperfusion-related neurodegenerative diseases. Brain Res Rev. 2007;54(1):162-80. [PubMed ID: 17296232]. https://doi.org/10.1016/j.brainresrev.2007.01.003.
-
35.
Adibhatla RM, Hatcher JF, Dempsey RJ. Effects of citicoline on phospholipid and glutathione levels in transient cerebral ischemia. Stroke. 2001;32(10):2376-81. [PubMed ID: 11588329].
-
36.
Rao AM, Hatcher JF, Dempsey RJ. Lipid alterations in transient forebrain ischemia: possible new mechanisms of CDP-choline neuroprotection. J Neurochem. 2000;75(6):2528-35. [PubMed ID: 11080206].
-
37.
Adibhatla RM, Hatcher JF. Citicoline mechanisms and clinical efficacy in cerebral ischemia. J Neurosci Res. 2002;70(2):133-9. [PubMed ID: 12271462]. https://doi.org/10.1002/jnr.10403.
-
38.
Novgorodov SA, Gudz TI. Ceramide and mitochondria in ischemic brain injury. Int J Biochem Mol Biol. 2011;2(4):347-61. [PubMed ID: 22187669].
-
39.
DeGirolami U, Zivin JA. Neuropathology of experimental spinal cord ischemia in the rabbit. J Neuropathol Exp Neurol. 1982;41(2):129-49. [PubMed ID: 7062084].
-
40.
Guan Z, Wang Y, Cairns NJ, Lantos PL, Dallner G, Sindelar PJ. Decrease and structural modifications of phosphatidylethanolamine plasmalogen in the brain with Alzheimer disease. J Neuropathol Exp Neurol. 1999;58(7):740-7. [PubMed ID: 10411344].
reply