Abstract
Background:
Plants have been the basis for medical treatments throughout much of human history. Although the active compounds of many herbal drugs are unknown, they have been widely prescribed by traditional medicine practitioners due to their beneficial effects and low cost. Although the primary feature of diabetes is hyperglycemia, diabetic disease can cause damage in several organs, such as the liver, over the long term.Objectives:
The present study was conducted to determine the hepatoprotective activity of Lavandula officinalis L. ethanolic extract (LOE) in alloxan-induced diabetic rats.Materials and Methods:
Twenty-eight male Wistar rats (weight 200 - 250 g) were randomly divided into four groups as follows: the healthy control group (HC) received saline (0.9% intraperitoneally [i.p.]) on all experimental days, the diabetic control group (DC) received a single dose of alloxan (120 mg/kg i.p.), the first treatment group (FT) received a single dose of alloxan and then 100 mg/kg of LOE i.p. for 21 days, and the second treatment group (ST) received a single dose of alloxan and then 200 mg/kg LOE i.p. for 21 days. After completion of the experimental period, liver tissue was dissected to measure the activity of certain antioxidant enzymes, including superoxide dismutase (SOD), catalase (CAT) and lipid peroxidation (MDA) by spectrophotometry. Blood samples were collected to measure aspartate transaminase (AST) and alanine transaminase (ALT) in the serum, using commercially available diagnostic kits.Results:
This study revealed that intraperitoneal administration of LOE for 21 days provided significant hepatoprotection against alloxan-induced elevation in the serum marker enzymes AST (38%) and ALT (38.65%, P < 0.05), the liver antioxidant enzyme SOD (68.61%, P < 0.01), CAT (41.53%), and liver lipid peroxidation (44.74%, P < 0.05) compared to the DC group.Conclusions:
The present study indicated a hepatoprotective effect of LOE in diabetic rats.Keywords
Alloxan Diabetes Hepatoprotective Lavandula Officinalis Oxidative Stress
1. Background
Plants have been the basis for medical treatments throughout much of human history, and such traditional medicine is still widely practiced today. Drugs of natural origins are less toxic and are considered to have fewer side effects than synthetic drugs (1). Natural remedies from medicinal plants are considered to be effective and safe alternative treatments for hyperglycemia and liver toxicity. The world health organization (WHO) estimates that for certain aspects of primary health care, 80% of the population in some countries uses herbal medicine. During the last several years, alternative treatments for diabetes have become popular, including medicinal herbs and nutritional completion (2). Diabetes is a common problem in modern society, with adverse effects on nearly all of the organs. Therefore, finding a natural treatment with the fewest side effects is necessary. Although the primary feature of diabetes is hyperglycemia, diabetic disease can cause damage in several organs, including hepatic tissues, over the long term. Increased production of reactive oxygen species (ROS) has a primary effect on the pathogenesis of tissue damage, as well as on the toxicity of an extensive range of compounds.
Lavandula officinalis (Lamiaceae), commonly known in Iran as “Ostokhoddous”, is a plant that has traditionally been used in medicine. In recent years, LO flowers have been shown to possess several biological and pharmacological properties, such as antitumor, antihistaminic, anti-inflammatory, antidiabetic, and antimicrobial potential, as well as central nervous system modification (3-8). However, no detailed scientific study seems to have been performed to discern the hepatoprotective activity of LO in diabetic diseases.
2. Objectives
Therefore, the present study was performed to assess the hepatoprotective activity of LO extract (LOE) in alloxan-induced oxidative stress in a diabetic model.
3. Materials and Methods
3.1. Chemicals
Ethylenediaminetetraacetic acid (EDTA) and trichloroacetic acid (TCA) were obtained from Sigma-Aldrich Chemical Co., Ltd. (USA). Thiobarbituric acid (TBA) was obtained from Merck Co. (Germany). Alloxan monohydrate was obtained from Acros Organics (NJ, USA).
3.2. Plant Materials
LO plants were obtained from the suburbs of Shiraz (Iran) at the beginning of the summer of 2015. The areal parts of the plants were air-dried and then ground into powder. During all steps, the components were protected from direct sunlight. The powder was stored at 8°C.
3.3. Extraction
The air-dried LO was ground into a powder, 200 g of which was mixed with an ethanol:water (5:1) solution at 25 ± 2 °C. The solvent was completely removed with a rotary vacuum evaporator at 50°C. Afterwards, the LOE was frozen at -20°C until use.
3.4. Animals and Treatment
The animals (Wistar male albino rats [200 - 250 g]) were obtained from the Pasteur Institute (Tehran, Iran). The animals were kept in a room with a temperature of 22 ± 2°C and a 12/12 hour light/dark cycle for one week before the experiment, and during the experiment. The animals were fed with a standard rodent pellet diet and clean drinking water ad libitum. All animal procedures were performed according to the national institute of health’s guide for the care and use of laboratory animals.
The animals were divided into four groups (n=7) as follows:
Group I: The healthy control group (HC), which received (saline 0.9% i.p.) once a day for 21 consecutive days; Group II: the diabetic control group (DC), which received a single dose of alloxan (120 mg/kg i.p.); Group III: the first treatment group (FT), which received a single dose of alloxan and then LOE 100 mg/kg i.p. for 21 days; Group IV: the second treatment group (ST), which received a single dose of alloxan and then LOE 200 mg/kg i.p. for 21 days.
3.5. Preparation of Liver Homogenate
After the treatment days, on day 22, the animals were sacrificed. The hepatic tissues were homogenized in KCl (10 mM) and phosphate buffer (1.15%) with EDTA (pH 7.4) and centrifuged at 3,000 rpm for 30 minutes. The supernatants were collected for measurements of malondialdehyde (MDA), catalase (CAT), and superoxide dismutase (SOD). The total protein content was determined based on Lowry’s method (9).
3.6. Designation of Lipid Peroxidation
Lipid peroxidation was measured based on the formation of thiobarbituric acid reactive substance (TBARS) from the liver homogenates. For preparation of thiobarbituric acid reagent, 2 mL (15% w/v TCA, 0.375% w/v TBA, and 0.25 M HCl) was added to 2 mL of the supernatant. The dilution was heated for 15 minutes in boiling water. After cooling, it was centrifuged at 1000g for 10 minutes, and the precipitate was removed. The MDA forms were mixed with TBA, which was measured with a spectrophotometer at 532 nm. The concentration of MDA was computed based on the absorbance coefficient of the TBA-MDA complex (ε = 1.56 × 105/M/cm), and was presented as nmol/mg of protein (10).
3.7. Antioxidant Enzyme Activity
For the measurement of catalase activity, one unit of catalase was required to decompose 1 µM of H2O2 in 1 minute. By adding 1.0 mL of 20 mM H2O2 (freshly prepared), the reaction was initiated. The decomposition level of H2O2 was determined with a spectrophotometer at 240 nm for 2 minutes. The enzyme activity was presented as U/mg of protein (11).
The SOD activity was assayed as described by Winterbourn et al. (1975). The reaction mixture contained 3 mL of 0.067 M potassium phosphate buffer (pH 7.8), 0.2 mL of 0.1 MEDTA, 0.3 mM of sodium cyanide (NaCN), and 0.1 mL of 1.5 mM nitroblue tetrazolium (NBT). One unit of enzyme activity (as the amount of SOD) was determined to produce a 50% inhibition of NBT reduction, and the specific enzyme activity was presented as units per milligram of total protein.
3.8. Assessment of Liver Function
Plasma aspartate aminotransferase (AST) and alanine aminotransferase (ALT) were measured using commercially available diagnostic kits (Biomaghreb, Ariana, Tunisia).
3.9. Histopathological Study
After the termination of the experimental days, the animals were sacrificed and their livers were immediately removed. The liver tissues were fixed in 10% formalin, the paraffin blocks of liver were created, and then histological sections 5 - 6 µm thick were prepared. The samples were stained with a hematoxylin-eosin solution and observed under a light microscope.
3.10. Statistical Analysis
The data are presented as mean ± SE. One-way analysis of variance (ANOVA), followed by multiple comparisons with the Tukey post-hoc test, was used to compare parameters between the groups. The significance levels were P < 0.05 and P < 0.01 compared to the diabetic control group.
4. Results
4.1. Effect of LOE on Lipid Peroxidation
The malondialdehyde (MDA) contents in the rat liver homogenates are shown in Figure 1. MDA in the liver homogenates were significantly increased (0.675 ± 0.078) in the DC groups compared with the HC group (0.43 ± 0.035) (P < 0.05). MDA levels in the LOE-treated groups (100 and 200 mg/kg) were significantly decreased (0.373 ± 0.0399) compared to the DC group (P < 0.05).
#, Indicates the significance at P < 0.05 for the control group; *, Indicates the significance at P < 0.05 for the diabetic group; Values are mean ± SE (n = 7 in each group).
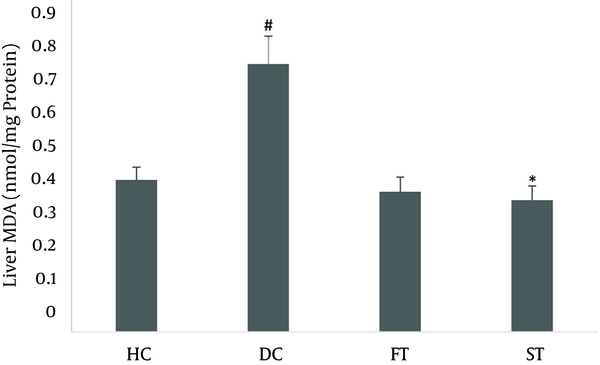
4.2. Effect of LOE on Antioxidant Enzyme Activity in Liver Tissue
The effects of LOE on SOD and CAT activity in the liver are shown in Figures 2 and 3. Antioxidant enzyme activity in the diabetic group, based on SOD (5.361 ± 0.406) and CAT (154.84 ± 6.11), was lower than in the normal controls (13.581 ± 0.21 and 180.31 ± 10.63, respectively; P < 0.05). The SOD activity (17.083 ± 0.66) and the CAT activity (264.83 ± 12.74) in the LOE-treated groups were significantly increased compared with the diabetic group (P < 0.01 and P < 0.05, respectively).
#, Indicates the significance at P < 0.05 for the control group; **, Indicates the significance at P < 0.01 for the diabetic group; Values are presented as mean ± SE (n = 7 in each group).
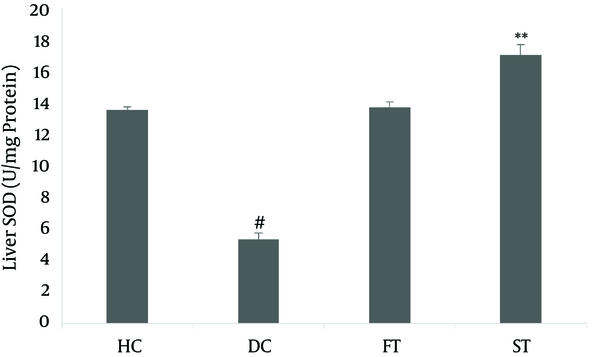
#, Indicates the significance at P < 0.05 for the control group; *, Indicates the significance at P < 0.05 for the diabetic group; Values are mean ± SE (n = 7 in each group).
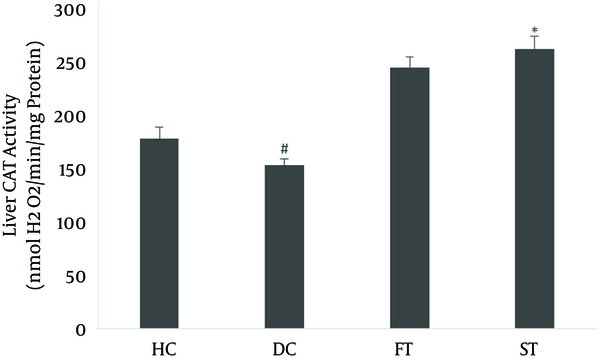
4.3. Effect of LOE on Liver Function
The exposure of rats to alloxan led to liver dysfunction, as indicated by the AST (185.5 ± 15.30) and ALT (119 ± 6.87) levels (Table 1). The activities of these enzymes in the LOE-treated group, with AST of 73.83 ± 5.52 and ALT of 115.83 ± 9.76, were significantly lower than in the diabetic controls (P < 0.05).
Group | ALT | AST |
---|---|---|
HC | 51.33 ± 5.31 | 134.5 ± 32.27 |
DC | 119 ± 6.87A | 185.5 ± 15.30C |
FT | 112.33 ± 12.25B | 142.66 ± 11.46 |
ST | 73.83 ± 5.52B | 115.83 ± 9.76D |
4.4. Effect of LOE on Liver Histopathology
Histological studies of the livers from the control, diabetic control, and LOE-treated groups showed normal hepatic architecture with distinct hepatic cells, as well as sinusoidal and portal spaces.
5. Discussion
This study was performed to investigate the hepatoprotective effects of LOE against liver injury in diabetic rats. Increased production of ROS has a primary effect on the pathogenesis of tissue damage, as well as toxicity, in an extensive range of compounds. Alloxan has been commonly used in rat experimental models to induce type I diabetes by oxidative stress that can cause damage in various organs, such as the liver.
Oxidative stress induces lipid peroxidation of the cell membrane’s polyunsaturated fatty acids (12). Lipid peroxidation is one of the most common reactions resulting from free-radical damage to biological structures, as well as accumulation of oxidized lipids in the cell membrane (13). Our results showed the depletion effect of LOE on TBARS production. In this study, alloxan administration significantly increased the hepatic MDA content, probably revealing the increased lipid peroxidation. The significant decrease in the hepatic MDA content, as a marker of lipid peroxidation, confirmed that treatment with LOE could have a significant protective effect against alloxan-induced hepatic lipid peroxidation.
Intracellular ROS concentration is a result of their production and elimination by diverse antioxidants. Major components of the antioxidant system in mammalian cells include SOD and CAT. These enzymes play important roles in eliminating superoxide anions and H2O2 in cells (14). SOD, an important antioxidant enzyme, catalyzes the highly reactive toxic superoxide radicals to H2O2 (15), and H2O2 decomposition to oxygen and water are catalyzed by catalase (16, 17). Our results indicated that the levels of the antioxidant enzymes SOD and CAT decreased in the alloxan-treated group, and recovered with LOE treatment. The protective effects of LOE in maintaining these enzymes close to the control level increased the capacity of endogenous antioxidant defenses, as well as their steady state. These effects can also enhance the enzymes’ synthesis rates, conferring enhanced protection against oxidative stress. The histopathological evaluations of rat livers treated with LOE, following administration of alloxan, showed a prophylactic effect of LOE on the nearly normal structures of the liver. This protection mechanism provides resistance for the liver against alloxan-induced damage, through hepatic regeneration stimulation and liver lipid peroxidation inhibition (18). The increased plasma AST and ALT levels indicated that diabetes may induce hepatic dysfunction, as supported by previous findings of necrotic livers (19). Therefore, the increased transaminase activity in plasma may be mainly due to the leakage of these enzymes from the liver (20). On the other hand, treatment of the alloxan-diabetic rats with LOE restored the transaminase activities. Several studies have shown the hepatoprotective effect of medical plants in diabetes (21), and these results are in line with those obtained by Ohaeri (22), illustrating the hepatoprotective effects of LOE against alloxan-induced toxicity.
The antioxidant components of LOE may cause membrane stabilization and reverse the normalization of fluctuating biochemical profiles induced by alloxan exposure. Therefore, plant extract compounds affect the liver by maintaining its normal function and decreasing the derangements of the cell membranes. Purification of LOE’s active components to determine their exact protective effects on hepatocytes is recommended for future studies. The present study indicated the presence of a hepatoprotective effect of LOE in diabetic rats.
References
-
1.
Valiathan MS. Healing plants. Curr Sci. 1998;75:1122-6.
-
2.
Sinha A, Formica C, Tsalamandris C, Panagiotopoulos S, Hendrich E, DeLuise M, et al. Effects of Insulin on Body Composition in Patients with Insulin‐dependent and Non‐insulin‐dependent Diabetes. Diabetic Med. 1996;13(1):40-6. https://doi.org/10.1002/(sici)1096-9136(199601)13:1<40::aid-dia991>3.3.co;2-l.
-
3.
Tahraoui A, El-Hilaly J, Israili ZH, Lyoussi B. Ethnopharmacological survey of plants used in the traditional treatment of hypertension and diabetes in south-eastern Morocco (Errachidia province). J Ethnopharmacol. 2007;110(1):105-17. [PubMed ID: 17052873]. https://doi.org/10.1016/j.jep.2006.09.011.
-
4.
Bellakhdar J, Claisse R, Fleurentin J, Younos C. Repertory of standard herbal drugs in the Moroccan pharmacopoea. J Ethnopharmacol. 1991;35(2):123-43. [PubMed ID: 1809818].
-
5.
Haloui M, Louedec L, Michel JB, Lyoussi B. Experimental diuretic effects of Rosmarinus officinalis and Centaurium erythraea. J Ethnopharmacol. 2000;71(3):465-72. [PubMed ID: 10940584].
-
6.
Tawaha K, Alali F, Gharaibeh M, Mohammad M, Elelimat T. Antioxidant activity and total phenolic content of selected Jordanian plant species. Food Chem. 2007;104(4):1372-8. https://doi.org/10.1016/j.foodchem.2007.01.064.
-
7.
Munchid K, Sadiq F, Tissent A. P 214 Cytotoxicity Of Essential Oil From Rosmarinus officinalis. Transfus Clin Biol. 2005;12(S1):5135-6.
-
8.
Hajhashemi V, Ghannadi A, Sharif B. Anti-inflammatory and analgesic properties of the leaf extracts and essential oil of Lavandula angustifolia Mill. J Ethnopharmacol. 2003;89(1):67-71. [PubMed ID: 14522434].
-
9.
Lowry OH, Rosebrough NJ, Farr AL, Randall RJ. Protein measurement with the Folin phenol reagent. J Biol Chem. 1951;193(1):265-75. [PubMed ID: 14907713].
-
10.
Buege JA, Aust SD. Microsomal lipid peroxidation. Methods Enzymol. 1978;52:302-10. https://doi.org/10.1016/s0076-6879(78)52032-6.
-
11.
Fowler WE, Buhle EL, Aebi U. Tubular arrays of the actin-DNase I complex induced by gadolinium. Proc Natl Acad Sci U S A. 1984;81(6):1669-73. [PubMed ID: 6584900].
-
12.
Halliwell B, Gutteridge JMC. Oxygen radicals and the nervous system. Trends Neurosci. 1985;8:22-6. https://doi.org/10.1016/0166-2236(85)90010-4.
-
13.
Janero DR. Malondialdehyde and thiobarbituric acid-reactivity as diagnostic indices of lipid peroxidation and peroxidative tissue injury. Free Radic Biol Med. 1990;9(6):515-40. [PubMed ID: 2079232].
-
14.
Marathe GK, Harrison KA, Murphy RC, Prescott SM, Zimmerman GA, McIntyre TM. Bioactive phospholipid oxidation products. Free Radic Biol Med. 2000;28(12):1762-70. [PubMed ID: 10946218].
-
15.
Nagata H, Takekoshi S, Takagi T, Honma T, Watanabe K. Antioxidative action of flavonoids, quercetin and catechin, mediated by the activation of glutathione peroxidase. Tokai J Exp Clin Med. 1999;24(1):1-11. [PubMed ID: 10530620].
-
16.
Reiter RJ, Tan DX, Osuna C, Gitto E. Actions of melatonin in the reduction of oxidative stress. A review. J Biomed Sci. 2000;7(6):444-58. [PubMed ID: 11060493].
-
17.
Baudrimont I, Ahouandjivo R, Creppy EE. Prevention of lipid peroxidation induced by ochratoxin A in Vero cells in culture by several agents. Chem Biol Interact. 1997;104(1):29-40. [PubMed ID: 9158693].
-
18.
Sadasivan S, Latha PG, Sasikumar JM, Rajashekaran S, Shyamal S, Shine VJ. Hepatoprotective studies on Hedyotis corymbosa (L.) Lam. J Ethnopharmacol. 2006;106(2):245-9. [PubMed ID: 16495024]. https://doi.org/10.1016/j.jep.2006.01.002.
-
19.
Whitehead MW, Hawkes ND, Hainsworth I, Kingham JG. A prospective study of the causes of notably raised aspartate aminotransferase of liver origin. Gut. 1999;45(1):129-33. [PubMed ID: 10369716].
-
20.
Larcan A, Lambert H, Laprevote-Heully MC, Delorme N. [Light and electron microscopic study of hepatic lesions in the course of hyperlactatemia in diabetic patients (author's transl)]. Diabete Metab. 1979;5(2):103-12. [PubMed ID: 113262].
-
21.
Nayak BS, Marshall JR, Isitor G, Adogwa A. Hypoglycemic and Hepatoprotective Activity of Fermented Fruit Juice of Morinda citrifolia (Noni) in Diabetic Rats. Evid Based Complement Alternat Med. 2011;2011:875293. [PubMed ID: 20981320]. https://doi.org/10.1155/2011/875293.
-
22.
Concepcion Navarro M, Pilar Montilla M, Martin A, Jimenez J, Pilar Utrilla M. Free radical scavenger and antihepatotoxic activity of Rosmarinus tomentosus. Planta Med. 1993;59(4):312-4. [PubMed ID: 8372145].