Abstract
Background:
The emergence of antibiotic resistance and the continuing evolution of resistance even to newer drugs have always been a global challenge. In the pursuit of innovative solutions, there is growing interest in using nanomaterials with antibacterial potentials to combat this menace.Objectives:
In this study, plant extract cocktail-mediated silver nanoparticles (PAgNPs) was used to stimulate the antibacterial effect of ampicillin (AMP) against multidrug-resistant (MDR) bacterial pathogens.Methods:
The agar well diffusion technique was used to investigate the potentials of PAgNPs in activating the antibacterial activity of AMP in MDR bacteria, including Escherichia coli, Klebsiella pnuemoniae, Micrococcus luteus, and Clostridium difficile. Plant extract cocktail-mediated silver nanoparticles were synthesized by reacting 9 mL of 1 mM of silver nitrate with 0.5 mL each of Citrus limon juice and aqueous Newbouldia laevis extract under bright sunlight for 10 minutes. Plant extract cocktail-mediated silver nanoparticles were characterized using UV-visible spectroscopy (UV-Vis) and higher resolution transmission electron microscopy (HRTEM) with energy dispersive x-ray spectroscopy (EDS).Results:
Plant extract cocktail-mediated silver nanoparticles colloidal solution was reddish-brown with a 441-nm surface plasmon resonance (SPR) absorption peak. The HRTEM micrograph showed the varied shaped silver nanoparticles (AgNPs) with the average size of 23.49 ± 10.49 nm. Similarly, EDS showed a strong typical metallic silver nanocrystallites absorption peak at 3.0 keV. The PAgNPs activate the AMP activity in MDR E. coli, M. luteus, K. pnuemoniae, and C. difficile.Conclusions:
The present findings suggest that PAgNPs can be considered a suitable candidate for developing novel antibacterial drugs to combat the growing threat of antibiotic resistance.Keywords
Newbouldia laevis Plant extract cocktail (PAgNPs) Nanotechnology Nanocrystallites Citrus limon
1. Background
Multidrug-resistant bacteria (MDRB) are dreadfully dangerous and pose a serious threat to the treatment of infectious diseases as they can survive the effect of many antibiotics (1, 2). However, the number of pathogens presenting multidrug resistance has increased exponentially, and this is considered a major public health concern (3, 4). The MDRB infections pose high management costs as affected individuals spend more time in hospitals and require therapies involving two or more antibiotics, highlighting the need for the development of novel and/or new antimicrobials (5).
Although the broad-spectrum antimicrobial activity of silver has long been established (6-8), its use as a therapy declined with the advent of antibiotics and their associated toxicity (9). Nowadays, the widespread use and in-depth studies of biogenic nanomaterial, particularly nanosilver (AgNPs) (10), presents a paradigm shift in using silver as a therapeutic agent (1, 11).
Chemical and physical methods have been successfully employed for the synthesis of AgNPs worldwide. These methods are often environmentally unsafe, laborious, and expensive (12). Accordingly, biosynthesis or bio-based synthesis using plants, microorganisms, and enzymes have emerged as alternatives (13). In general, plant-mediated nano synthesis is cost-effective and pro-environmental with a simple nanoparticle biosynthesis process that can be used for human therapeutic and food-based applications (14).
Plants encompass biomolecules that can reduce and stabilize metal ions to nanoparticles. They include co-enzymes and diverse water-soluble metabolites such as alkaloids, phenolic compounds, and terpeniods (14). Citrus limon belongs to the family Rutaceae (15). It is an elliptically shaped berry fruit, and its juice exhibits a range of protective health benefits, including antioxidative, anti-inflammatory, antitumor, and antimicrobial (15-17). Newbouldia laevis is a ubiquitous angiosperm in the family Bignoniaceae (18). In Nigeria, it is popularly known as the tree of life or fertility tree owing to its wide uses in traditional folklore (19, 20). The decoction of leaves, stem, bark, and root are used to treat diarrhea, dysmenorrhoea convulsions, chest pains, constipation piles, dysentery, ophthalmia, and conjunctivitis (19-22).
Thus far, the use of diverse species of plants in the synthesis of AgNPs has been well documented (23-26). However, the combination of two plants which may provide a myriad of bioactive components to further decrease the stability potentials of individual plants has not been addressed in previous research.
2. Objectives
The present study examined the synergistic effect of C. limon juice, and N. laevis leave extracts in nanosilver synthesis. The effect of the PAgNPs and the combinatorial effect of AMP on the MDR bacteria were also investigated.
3. Methods
3.1. Collection and Identification of Plant Material
Fresh C. limon fruits (lemon) were purchased from Kure Market, Minna, Nigeria, while N. laevis leaves were collected from Maikunkele, Minna, Niger State. Both plant materials were taken to the Department of Plant Biology, Federal University of Technology Minna, Nigeria, for further examination by botanists. A Voucher speciman number (FUTMIN_M107) was given, and the specimen was deposited in the herbarium.
3.2. Processing and Extraction of Plant Materials
The fresh C. limon fruits were rinsed compeletly with distilled water and were cut into halves. The juice was extracted from the fruits using a juice extractor. The extracted juice was then subjected to double filtration with Whatman No. 1 (Germany) filter paper (pore size 25 μm). The leaves of N. laevis were carefully washed with distilled water and air-dried at 28 ± 2°C for seven days. The dried leaves were then pulverized using an electric blender. Five grams (5g) of the powdered N. laevis leaves were added to 100 mL of deionized water in a conical flask and placed in a water bath at 60°C for 30 minutes. The mixture was filtered with Whatman No. 1 (Germany) filter paper (pore size 25 μm). The filtrate of N. laevis and C. limon fruits extracts was centrifuged at 8000 rpm for 10 minutes. The supernatants were decanted and stored at -4°C for further usage (14).
3.3. Qualitative Determination of Phytochemicals Present in Citrus limon Juice and Newbouldia laevis Extracts
Citrus limon juice and N. laevis extracts were screened using the standard methods described by Kuta et al. to detect the presence or absence of various secondary metabolites such as saponins, flavonoids, alkaloids, steroids, anthraquinones, cardiac glycosides, tannins, phenols, terpenes, and resins (27).
3.4. Biosynthesis of Silver Nanoparticles
Nine milliliters (9 mL) of 10-3 M silver nitrate solution were added to four separate 20 mL specimen bottles labelled A - C. One milliliter (1 mL) of both C. limon juice extract and N. laevis was added to A and B; however, a cocktail (0.5 mL of both C. limon juice and N. laevis leaf extract) was added to C. In this study, 9 mL of 10-3 M of silver nitrite solution was only used as a control (28). The resulting solutions were kept under sunlight. The experiment was visually observed for possible colour.
3.5. Characterization of Biosynthesized Nanoparticles
The synthesis of the AgNPs was evaluated using a UV-Vis spectrophotometer to determine the absorption spectra of synthesized AgNPs. The size, shape, crystal, and lattice structure of the biosynthesized silver nanoparticles were measured using a high-resolution transmission electron microscope (Carl Zeiss AG, Germany). The elemental composition of the synthesized AgNPs was determined by energy-dispersive x-ray spectroscopy (EDS) attached to the HR-SEM. The sample size was determined by transmission electron micrographs using the ImageJ software (Version 2.0).
3.6. Source of Bacterial Cultures
Six multidrug-resistant bacterial pathogens from the diarrheic and wound swabs of patients attending the General Hospital, Minna, Nigeria, were collected to investigate the antibacterial effect of biosynthesized AgNPs. The bacteria isolates, including Escherichia coli, Salmonella typhi, Micrococcus luteus, and Clostridium difficile, were obtained from the stock culture in the Microbiology Laboratory General Hospital, Minna, Nigeria. A biochemical test was then carried out to confirm the identity of the isolates using methods described by Cheesebrough (29).
3.7. Antibiotic Susceptibility Test
Kirby Bauer’s agar disc diffusion method was used for the antibiotic susceptibility test described in the Clinical and Laboratory Standards Institute (CLSI) guidelines (30). The discs of antibiotics (Oxoid) commonly used for treating bacterial infection (Gram-negative and positive) were tested. Individual colonies were suspended in normal saline to 0.5 McFarland standards and using sterile swabs. The suspensions were inoculated on Muller Hinton agar for 24 hours at 37°C. Sterile 6 mm Whatman filter paper No. 3 (Germany) impregnated with sterile distilled water was used as a control.
3.8. Antibacterial Activity of Biosynthesized Silver Nanoparticles
The agar well diffusion method was used as described in the guidelines of the Clinical and Laboratory Standards Institute (30) to determine the antibacterial effect of the AgNPs. Twenty milliliters (20 mL) of sterilized Muller agar was dispensed into petri dishes. The agar was allowed to set, and 5 mm wells were bored on each plate using a sterile cork borer (29). Each of the test isolates was suspended in normal saline to 0.5 McFarland standards. A swab stick was used to inoculate culture suspension on the Muller Hinton agar plates. Accordingly, the wells were filled with 160 μL of lemon juice, and N. laevis leaf extract, a mixture of the two plant extract (0.5 mL each of both lemon juice and N. laevis extract), cocktail mediated AgNPs, and AMP mixed with the cocktail mediated AgNPs using microtiter-pipette. This allowed to diffuse at room temperature for one hour. All the plates were incubated at 37°C for 24 hours aerobically, except for C. difficile, which was incubated anaerobically (31). All tests were conducted in triplicate.
3.9. Data Analysis
Data were analyzed with statistical analysis software (SAS) (Version: SAS 9.4, SAS Institute Inc., Cary, NC, USA) and presented as means ± standard error of the mean. The mean values of the inhibition zones were comparied using one-way analysis of variance (ANOVA) and Duncan’s Multiple Range Test (DMRT). In this study, P < 0.05 was set as the significance level.
4. Results
4.1. Phytochemical Constituents of Citrus limon Juice and Newbouldia laevis Extracts
The phytochemical analyses showed the presence of saponins, resins, glycosides, cardiac steroids, steroids, tannins, phenols, and terpenes in C. limon juice and N. laevis leave extracts. Moreover, flavonoids, alkaloids, volatile oils, anthraquinone, and reduced sugar were detected in C. limon juice extract (Table 1).
Phytochemicals | Citrus limon Juice Extract | Newbouldia laevis Leaves |
---|---|---|
Flavonoids | + | - |
Phenols | + | + |
Alkaloids | + | - |
Tannins | + | + |
Steroids | + | + |
Cardiac steroids | + | + |
Glycosides | + | + |
Volatile oil | + | - |
Anthraquinone | + | - |
Resins | + | + |
Saponins | + | + |
Terpenes | + | + |
Reducing sugar | + | - |
4.2. Biosynthesis of Silver Nanoparticles
The biosynthesis of AgNPs was indicated by gradually emerging a reddish-brown colour in the reaction solution after 10 minutes of incubation under solar irradiation, followed by an increase in color intensity in the course of the reaction.
4.3. Characterization of Silver Nanoparticles
Figure 1 shows the UV-Vis spectra of the N. laevis silver nanoparticles (NAgNPs), C. limon juice extract silver nanoparticles (CAgNPs), and C. limon juice-N. laevis silver nanoparticles (CNAgNPs). The absorbance peaks (421, 435, and 441 nm) indexed as different colours indicated the reduction of AgNO3 by N. laevis leave extract, C. limon juice extract, and C. limon juice-N. laevis leave extract within 10 minutes under solar irradiation (Figure 1).
UV-visible spectroscopy absorption spectrum of AgNO3 and biosynthesized silver nanoparticles; A, AgNO3 solution; B, Newbouldia laevis extract nanosilver (NAgNPs); C, Citrus limon juice extract nanosilver (CAgNPs); D, C. limon juice and N. laevis extract nanosilver (PAgNPs)
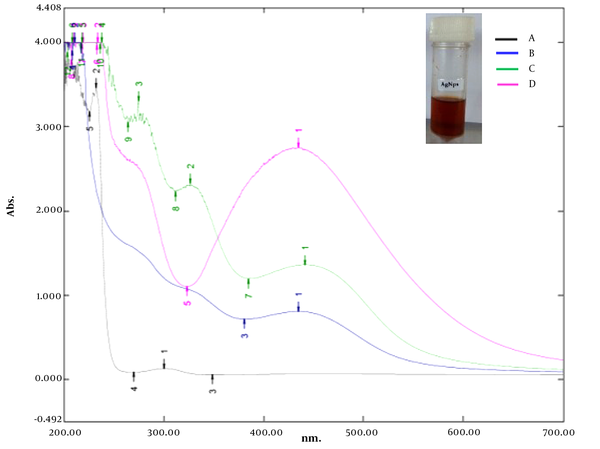
4.4. Morphology of Synthesized Plant Extract Cocktail-Mediated Silver Nanoparticles
The HRTEM micrograph of NAgNPs and CAgNPs reveals the formation of aggregated and partially-distributed spherical particles with an average size of 30.50 ± 8.6 and 17.5 ± 4.31 nm, respectively (Figures 2 and 3). The PAgNPs HRTEM image shows uneven-shaped aggregate particles with an average size of 23.49 ± 10.49 nm (Figure 3). The SAED patterns for NAgNPs, CAgNPs, and PAgNPs show a clear circular ring, suggesting the polycrystalline nature of the AgNPs (Figure 2B, 3B and 4B). The EDS spectra confirmed that AgNPs were composed of silver with a strong typical metallic silver nanocrystallites absorption.
Peak at 3.0 keV (Figure 2C, 3C and 4C).
Characteristics of synthesized Newbouldia laevis silver nanoparticles (NAgNPs); A, transmission electron microscopy image of NAgNPs; B, selected area electron diffraction of NAgNPs; C, energy-dispersive x-ray analysis of silver nanoparticles
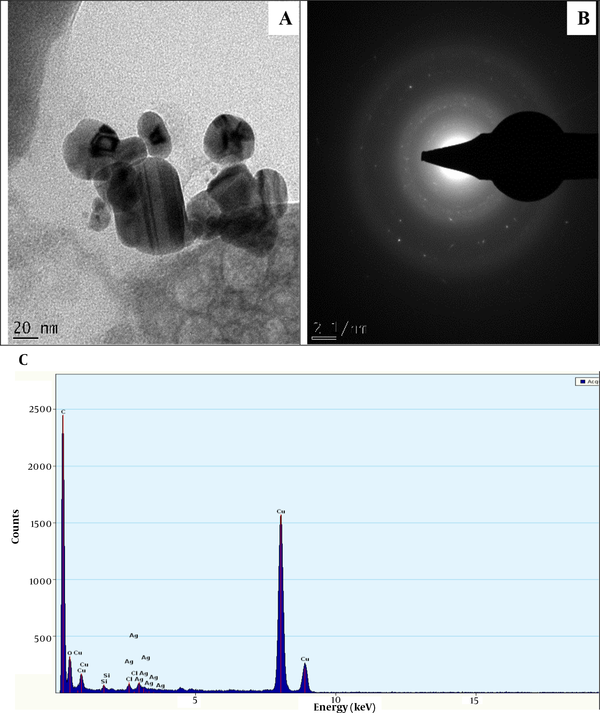
Characteristics of synthesized Citrus limon juice extract silver nanoparticles (CAgNPs); A, transmission electron microscopy image of CAgNPs; B, selected area electron diffraction of CAgNPs; C, energy-dispersive x-ray analysis of CAgNPs
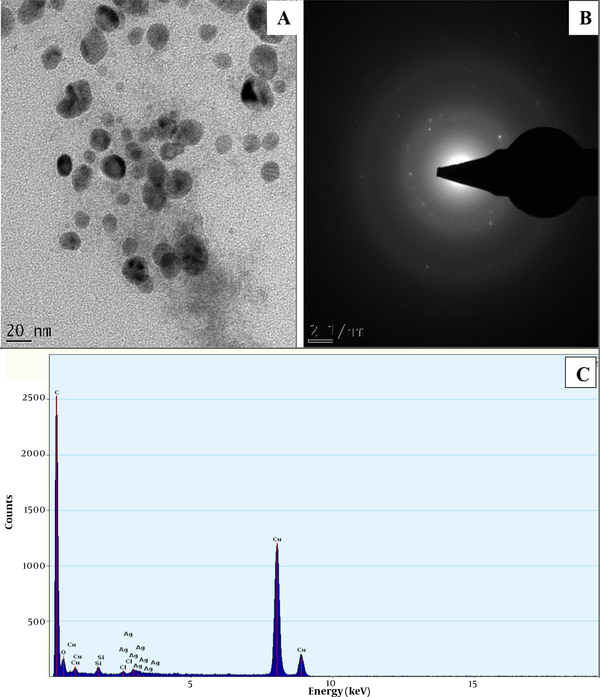
Characteristics of synthesized plant extract cocktail-mediated silver nanoparticles (PAgNPs); A, transmission electron microscopy image of PAgNPs; B, selected area electron diffraction of PAgNPs; C, energy-dispersive x-ray analysis of PAgNPs
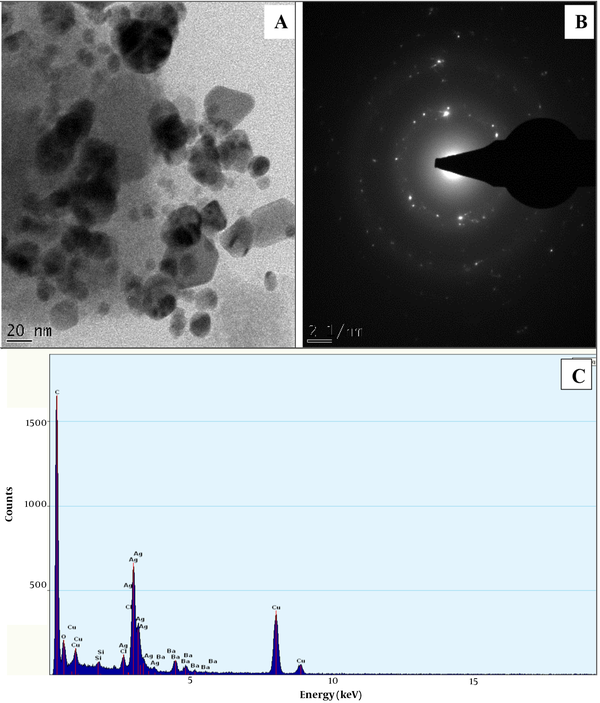
4.5. Biochemical Characteristics of the Test Isolates
The bacterial isolates were identified biochemically, as presented in Table 2.
Biochemical Test Results Obtained for the Test Isolates
G-R | Sh | Cat | Cit | Pig | Glu | Man | Indole | Urease | MR | VP | Lactose | H2S | Slant | Butt | Inference |
---|---|---|---|---|---|---|---|---|---|---|---|---|---|---|---|
- | Rod | + | + | NA | + | + | - | + | - | + | + | - | NC | NC | Klebsiella pnuemoniae |
+ | Cocci | + | + | Yellow | - | - | - | + | NA | + | - | - | NA | NA | Micrococcus luteus |
+ | Rod | - | NA | + | - | - | - | NA | - | - | NA | NA | NA | Clostridium difficile | |
- | Rod | + | - | NA | + | + | + | - | + | - | + | - | Acid | Acid | Escherichia coli |
4.6. Antibiotic Susceptibility Profile of Test Bacterial Pathogens
Escherichia coli, Klebsiella pnuemoniae, M. luteus, and C. difficile were resistant to ciprofloxacin; however, K. pneumoniae, M. luteus, and C. difficile showed resistance to AMP, erythromycin, and ofloxacin. Similarly, E. coli, K. pneumoniae, and M. luteus were susceptible to augmentin, cefalexin, and nalidixic acid (Table 3).
Antibiotic Susceptibility Test of the Test Isolate
Antibiotic (ug) | Escherichia coli | Klebsiella pnuemoniae | Micrococcus luteus | Clostridium difficile |
---|---|---|---|---|
Ciprofloxacin (10) | ++ | ++ | ++ | ++ |
Gentamycin (10) | + | ++ | - | ++ |
Streptomycin (30) | - | + | - | ++ |
Nalidixic acid (30) | + | - | - | ++ |
Ampicillin (30) | - | ++ | ++ | ++ |
Erythromycin (30) | - | ++ | ++ | ++ |
Cefalexin (10) | - | - | - | ++ |
Ofloxacin (5) | + | ++ | ++ | ++ |
Augmentin (30) | - | - | - | ++ |
Reflacine (10) | - | ++ | - | + |
4.7. Antibacterial Susceptibility Pattern of Multidrug-Resistant Pathogens to Newbouldia laevis Silver Nanoparticles, Citrus limon Juice Extract Silver Nanoparticles, and Plant Extract Cocktail-Mediated Silver Nanoparticles Singly and in Combination with Ampicillin
The NAgNPs induced the lowest zones of inhibition against E. coli, M. luteus, K. pnuemoniae, and C. difficile, and the highest zones of inhibition against these isolates were produced by CAgNPs. The synergistic effect of biosynthesized AgNPs was the largest when PAgNPs were combined with AMP, and the inhibition zones produced ranged from 26.0 ± 1.4 to 40.5 ± 0.71 (Table 4).
Zones of Inhibition Produced by Each of Newbouldia laevis Silver Nanoparticles, Citrus limon Juice Extract Silver Nanoparticles, Plant Extract Cocktail-mediated Silver Nanoparticles in Combination with Ampicillin a, b
Zones of Inhibition (mm) (n = 3) | ||||||||
---|---|---|---|---|---|---|---|---|
Isolates | CAgNPs | NAgNPs | PAgNPs | CAgNPs + A | NAgNPs + A | PAgNPs + A | AgNO3 | C-N Extract |
Escherichia coli | 26.5 A ± 3.54 | 22.0 B ± 0.00 | 24.5 C ± 3.54 | 31.0 D ± 1.41 | 24.0 C ± 0.00 | 34.0 D ± 1.41 | 19.0 E ± 0.00 | 0.0 F ± 0.00 |
Micrococcus luteus | 23.0 E ± 4.24 | 22.0 E ± 0.00 | 24.0 B ± 1.41 | 29.5 A ± 0.71 | 25.0 C ± 0.00 | 40.5 D ± 0.71 | 18.0 D ± 0.00 | 0.0 F ± 0.00 |
Klebsiella pnuemoniae | 28.0 D ± 1.41 | 21.0 B ± 0.00 | 21.5 B ± 0.71 | 27.0 A ± 1.41 | 23.0 C ± 0.00 | 29.0 D ± 1.41 | 20.0 E ± 0.00 | 0.0 F ± 0.00 |
Clostridium difficile | 28.0 A ± 2.83 | 20.0 F ± 0.00 | 18.0 E ± 5.66 | 31.0 D ± 1.41 | 24.0 B ± 0.00 | 31.0 D ± 1.41 | 18.0 G ± 0.00 | 0.0 H ± 0.00 |
5. Discussion
Plant extract-mediated nanoparticles are undoubtedly the most explored route in the biosynthesis of nanometals due to their simplicity and the wide availability of species to be explored (32). The reddish-brown appearance of the reaction solution (Figure 1, inset) signaled the formation of AgNPs in the reaction mixture within 10 minutes. A similar conclusion was made by some other researchers (24, 33-37).
The surface plasmon resonance (SPR) absorption maximum peaks of 421, 435, and 441 nm for NAgNPs, CAgNPs, and CNAgNPs revealed by UV-Vis spectral analysis match the characteristic AgNPs SPR at 400 - 480 nm wavelengths (24, 36, 37). An increase in the abundance of bioactive metabolites in the extract of both plants (Table 1) increased the intensity and wavelength of the absorption (421 - 441 nm). The observed variation in the values of absorbance peaks shows a change in the particle size and shape (24, 37), confirming the findings described in a previous study (32). This is in line with the HRTEM micrograph, as presented in Figures 2A, 3A and 4A.
The HRTEM analysis revealed the surface morphology of the biogenic AgNps (Figures 2A, 3A and 4A). The comparison of the findings showed that the diameter of prepared nanoparticles in the solution was about 18 - 31 nm (Figures 2A, 3A and 4A). This might be associated with the difference in the compositions of bioactive metabolites in both plant extracts, as presented in Table 1. The unevenly or irregularly -shaped particle obtained for CNAgNPs compared to NAgNPs and CAgNPs singly could be due to the interaction of the myriads of bioactive metabolites offered by both C. limon juice, and N. laevis extracts with silver ion. Different plant extracts with different bioactive metabolites have demonstrated different potentials in preparing AgNPs (25, 37-39); hence, the result of the present study is comparable with previous reports.
Recently, the optimization of nanomaterial surface chemistries has received serious attention due to the increasing demand of stable and target-specific nanomaterials for biological applications (9, 16). The size and shape of AgNPs play a significant role in their antibacterial activity (40); hence, our study offers an ecofriendly method for synthesizing nanosilver with varied physical properties (e.g., shape and size) for possible use in a biological system.
The EDS spectrum revealed the elemental composition of the biogenic AgNps (Figures 2C, 3C and 4C). The synthesized AgNPs were crystalline due to reduced silver ions, as shown by a strong typical metallic silver nanocrystallites absorption peak at 3.0 keV 30 (Figures 2C, 3C and 4C). Comparable results (25, 37-39) for EDS analysis of biosynthesized AgNPs have been reported; therefore, our finding is in line with previous reports.
The size of AgNPs has been linked to significant nanoparticle antibacterial activities (40); CAgNPs synthesized by C. limon juice extra (17.5 ± 4.31 nm in size) exhibited higher antibacterial activity against MDR bacterial clinical isolates when compared to 30.50 ± 8.6- and 23.49 ± 10.49-nm NAgNPs and PAgNPs, respectively (Table 4).
Consistent with the findings of Rai et al. (41), Potara et al. (42), and Panacek et al. (43), this study showed that the combination of AMP with AgNPs stimulated its antibacterial effect (Table 4). This finding reiterates the multi-level mode of antibacterial action of AgNPs (41, 44). Since the AMP mode of action is the obstruction of cell wall synthesis, the stimulation of AMP sensitivity against the test bacteria isolates upon its combination with PAgNPs may lead to severe damage to their cell walls (41). Furthermore, PAgNPs may facilitate the transport of AMP to the cell surface resulting in the increased permeability of the membrane AMP into the cells more easily (41).
One possible explanation for the high susceptibility of E. coli to AgNPs + AMP is the susceptibility of the test E. coli strain to AMP (Table 1). Furthermore, all AMP -resistant-MDR bacteria in this study were susceptible to biogenic AgNPs (Table 4). The high sensitivity of these bacteria strains to AgNPs explained their stronger synergistic effects.
5.1. Conclusions
The combination of bioactive metabolites offered by C. limon juice enhanced the reduction and stability potential of N. laevis extracts and resulted in varied shaped AgNPs. The combination of AMP with AgNPs reinstates its antibacterial effect against MDR of E. coli, M. luteus, K. pnuemoniae, and C. difficile. Accordingly, our synthesis protocol should be optimized to synthesize the varied shaped AgNPs of superior physicochemical properties for downstream applications such as the delivery of antibiotics to target sites to combat the menace of antibiotic resistance.
References
-
1.
Barros CHN, Fulaz S, Stanisic D, Tasic L. Biogenic Nanosilver against Multidrug-Resistant Bacteria (MDRB). Antibiotics (Basel). 2018;7(3). [PubMed ID: 30072622]. [PubMed Central ID: PMC6163489]. https://doi.org/10.3390/antibiotics7030069.
-
2.
Ayukekbong JA, Ntemgwa M, Atabe AN. The threat of antimicrobial resistance in developing countries: causes and control strategies. Antimicrob Resist Infect Control. 2017;6:47. [PubMed ID: 28515903]. [PubMed Central ID: PMC5433038]. https://doi.org/10.1186/s13756-017-0208-x.
-
3.
World Health Organization. WHO Publishes List of Bacteria for Which New Antibiotics Are Urgently Needed. Geneva, Switzerland: World Health Organization; 2017, [cited 10 June]. Available from: http://www.who.int/en/news-room/detail/27-02-2017-who-publishes-listof-bacteria-for-which-new-antibiotics-are-urgently-needed.
-
4.
Roca I, Akova M, Baquero F, Carlet J, Cavaleri M, Coenen S, et al. The global threat of antimicrobial resistance: science for intervention. New Microbes New Infect. 2015;6:22-9. [PubMed ID: 26029375]. [PubMed Central ID: PMC4446399]. https://doi.org/10.1016/j.nmni.2015.02.007.
-
5.
Lara HH, Ayala-Núnez NV, Ixtepan Turrent LDC, Rodríguez Padilla C. Bactericidal effect of silver nanoparticles against multidrug-resistant bacteria. World J Microbiol Biotechnol. 2010;26(4):615-21.
-
6.
Dastjerdi R, Montazer M. A review on the application of inorganic nano-structured materials in the modification of textiles: focus on anti-microbial properties. Colloids Surf B Biointerfaces. 2010;79(1):5-18. [PubMed ID: 20417070]. https://doi.org/10.1016/j.colsurfb.2010.03.029.
-
7.
Ballottin D, Fulaz S, Cabrini F, Tsukamoto J, Duran N, Alves OL, et al. Antimicrobial textiles: Biogenic silver nanoparticles against Candida and Xanthomonas. Mater Sci Eng C Mater Biol Appl. 2017;75:582-9. [PubMed ID: 28415502]. https://doi.org/10.1016/j.msec.2017.02.110.
-
8.
Ertem E, Gutt B, Zuber F, Allegri S, Le Ouay B, Mefti S, et al. Core-Shell Silver Nanoparticles in Endodontic Disinfection Solutions Enable Long-Term Antimicrobial Effect on Oral Biofilms. ACS Appl Mater Interfaces. 2017;9(40):34762-72. [PubMed ID: 28922597]. https://doi.org/10.1021/acsami.7b13929.
-
9.
Khatoon N, Alam H, Khan A, Raza K, Sardar M. Ampicillin Silver Nanoformulations against Multidrug resistant bacteria. Sci Rep. 2019;9(1):6848. [PubMed ID: 31048721]. [PubMed Central ID: PMC6497658]. https://doi.org/10.1038/s41598-019-43309-0.
-
10.
Salomoni R, Leo P, Montemor AF, Rinaldi BG, Rodrigues M. Antibacterial effect of silver nanoparticles in Pseudomonas aeruginosa. Nanotechnol Sci Appl. 2017;10:115-21. [PubMed ID: 28721025]. [PubMed Central ID: PMC5499936]. https://doi.org/10.2147/NSA.S133415.
-
11.
Radetić M. Functionalization of textile materials with TiO2 nanoparticles. J. Photochem. Photobiol. C: Photochem. Rev. 2013;16:62-76. https://doi.org/10.1016/j.jphotochemrev.2013.04.002.
-
12.
Wang L, Hu C, Shao L. The antimicrobial activity of nanoparticles: present situation and prospects for the future. Int J Nanomedicine. 2017;12:1227-49. [PubMed ID: 28243086]. [PubMed Central ID: PMC5317269]. https://doi.org/10.2147/IJN.S121956.
-
13.
Gopinath B, Hickson L, Schneider J, McMahon CM, Burlutsky G, Leeder SR, et al. Hearing-impaired adults are at increased risk of experiencing emotional distress and social engagement restrictions five years later. Age Ageing. 2012;41(5):618-23. [PubMed ID: 22591986]. https://doi.org/10.1093/ageing/afs058.
-
14.
Mohanapriya M, Ramaswamy L, Rajendran R. Health and medicinal properties of lemon (Citrus limonum). International Journal of Ayurvedic and Herbal Medicine. 2013;3(1):1095-100.
-
15.
Kumar V, Yadav SK. Plant-mediated synthesis of silver and gold nanoparticles and their applications. J Chem Technol Biotechnol. 2009;84(2):151-7. https://doi.org/10.1002/jctb.2023.
-
16.
Kumari M, Pandey S, Giri VP, Bhattacharya A, Shukla R, Mishra A, et al. Tailoring shape and size of biogenic silver nanoparticles to enhance antimicrobial efficacy against MDR bacteria. Microb Pathog. 2017;105:346-55. [PubMed ID: 27889528]. https://doi.org/10.1016/j.micpath.2016.11.012.
-
17.
Mittal AK, Chisti Y, Banerjee UC. Synthesis of metallic nanoparticles using plant extracts. Biotechnol Adv. 2013;31(2):346-56. [PubMed ID: 23318667]. https://doi.org/10.1016/j.biotechadv.2013.01.003.
-
18.
Adu F, Antwi Apenteng J, Kuntworbe N, Gariba Akanwariwiak W, Appiah T, Ntinagyei Mintah D. Antimicrobial and antioxidant activities of Citrus sinensis var. late Valencia fruits at various stages of development. Afr J Microbiol Res. 2016;10(3):73-8. https://doi.org/10.5897/ajmr2015.7725.
-
19.
Pandey A, Kaushik A, Tiwari SK. Evaluation of antimicrobial activity and phytochemical analysis of Citrus limon. J Pharm Biomed Sci. 2011;13(13):1-7.
-
20.
Cotelle N, Bernier JL, Catteau JP, Pommery J, Wallet JC, Gaydou EM. Antioxidant properties of hydroxy-flavones. Free Radic Biol Med. 1996;20(1):35-43. [PubMed ID: 8903677]. https://doi.org/10.1016/0891-5849(95)02014-4.
-
21.
Burkill HM; Royal Botanic Gardens Kew. Newbouldia laevis Seem, family Bignoniaceae. In: Burkill HM, editor. The Useful Plants of West Tropical Africa. 1. Virginia, United States: University Press of Virginia; 1985.
-
22.
Bafor E, Sanni U. Uterine contractile effects of the aqueous and ethanol leaf extracts of Newbouldia Laevis (Bignoniaceae) in vitro. Indian J Pharm Sci. 2009;71(2):124-7. [PubMed ID: 20336206]. [PubMed Central ID: PMC2839394]. https://doi.org/10.4103/0250-474X.54274.
-
23.
Sakande J, Kabre E, Lompo M, Pale E, Nikiema JB, Nacoulma OG, et al. Anti-inflammatory and Antioxidant Activities of a Fraction I1 of Male Inflorescences of Borassus aethiopum Mart (Arecaceae). Am J Biochem Mol Biol. 2012;3(1):101-9. https://doi.org/10.3923/ajbmb.2013.101.109.
-
24.
Egba SI, Sunday GI, Anaduaka EG. The effect of oral administration of aqueous extract of Newbouldia laevis leaves on fertility hormones of male albino rats. J Pharm Biol Sci. 2014;9(3):61-4.
-
25.
Akerele JO, Ayinde BA, Ngiagah J. Phytochemical and Antibacterial Evaluations of the Stem Bark of Newbouldia laevis against Isolates from Infected Wounds and Eyes. Trop J Pharm Res. 2011;10(2):211-8. https://doi.org/10.4314/tjpr.v10i2.66566.
-
26.
Patra JK, Baek KH. Antibacterial Activity and Synergistic Antibacterial Potential of Biosynthesized Silver Nanoparticles against Foodborne Pathogenic Bacteria along with its Anticandidal and Antioxidant Effects. Front Microbiol. 2017;8:167. [PubMed ID: 28261161]. [PubMed Central ID: PMC5309230]. https://doi.org/10.3389/fmicb.2017.00167.
-
27.
Kuta FA, Oyedum UM, Alimi GS, David BJ, Saheed AA. Antibacterial activities of Vitelleria paradoxa on some enteric bacteria. Nigerian Journal of Microbiology. 2017;31(1):3882-92.
-
28.
Valli JS, Vaseeharan B. Biosynthesis of silver nanoparticles by Cissus quadrangularis extracts. Materials Letters. 2012;82:171-3. https://doi.org/10.1016/j.matlet.2012.05.040.
-
29.
Cheesbrough M. District Laboratory Practice in Tropical Countries, Part 2. 2nd ed. New York, USA: Cambridge University Press; 2006.
-
30.
Das AJ, Kumar R, Goutam SP. Sunlight Irradiation Induced Synthesis of Silver Nanoparticles using Glycolipid Bio-surfactant and Exploring the Antibacterial Activity. J Bioengineer & Biomedical Sci. 2016;6(5). https://doi.org/10.4172/2155-9538.1000208.
-
31.
Clinical Laboratory Standard Institutes. M100 Performance Standards for Antimicrobial Susceptibility Testing. 28th ed. Illinois, United States: Clinical Laboratory Standard Institutes; 2018.
-
32.
Idu M. Antimicrobial Activity and Phytochemistry of Khaya Senegalensis Roots. International Journal Of Ayurvedic And Herbal Medicine. 2012;2(3). https://doi.org/10.47191/ijahm.
-
33.
Kora AJ, Manjusha R, Arunachalam J. Superior bactericidal activity of SDS capped silver nanoparticles: Synthesis and characterization. Mater Sci Eng C. 2009;29(7):2104-9. https://doi.org/10.1016/j.msec.2009.04.010.
-
34.
Ahmed S, Ahmad M, Swami BL, Ikram S. A review on plants extract mediated synthesis of silver nanoparticles for antimicrobial applications: A green expertise. J Adv Res. 2016;7(1):17-28. [PubMed ID: 26843966]. [PubMed Central ID: PMC4703479]. https://doi.org/10.1016/j.jare.2015.02.007.
-
35.
Wu XW, Liu C, Li L, Jones P, Chantrell RW, Weller D. Nonmagnetic shell in surfactant-coated FePt nanoparticles. Journal of Applied Physics. 2004;95(11):6810-2. https://doi.org/10.1063/1.1667803.
-
36.
Kumar SA, Peter YA, Nadeau JL. Facile biosynthesis, separation and conjugation of gold nanoparticles to doxorubicin. Nanotechnology. 2008;19(49):495101. [PubMed ID: 21730661]. https://doi.org/10.1088/0957-4484/19/49/495101.
-
37.
Meena Kumari M, Philip D. Facile one-pot synthesis of gold and silver nanocatalysts using edible coconut oil. Spectrochim Acta A Mol Biomol Spectrosc. 2013;111:154-60. [PubMed ID: 23624042]. https://doi.org/10.1016/j.saa.2013.03.076.
-
38.
Pal S, Tak YK, Song JM. Does the antibacterial activity of silver nanoparticles depend on the shape of the nanoparticle? A study of the Gram-negative bacterium Escherichia coli. Appl Environ Microbiol. 2007;73(6):1712-20. [PubMed ID: 17261510]. [PubMed Central ID: PMC1828795]. https://doi.org/10.1128/AEM.02218-06.
-
39.
Nazeruddin GM, Prasad NR, Waghmare SR, Garadkar KM, Mulla IS. Extracellular biosynthesis of silver nanoparticle using Azadirachta indica leaf extract and its anti-microbial activity. J Alloys Compd. 2014;583:272-7. https://doi.org/10.1016/j.jallcom.2013.07.111.
-
40.
Bhakya S, Muthukrishnan S, Sukumaran M, Muthukumar M. Biogenic synthesis of silver nanoparticles and their antioxidant and antibacterial activity. Appl Nanosci. 2015;6(5):755-66. https://doi.org/10.1007/s13204-015-0473-z.
-
41.
Rai MK, Deshmukh SD, Ingle AP, Gade AK. Silver nanoparticles: the powerful nanoweapon against multidrug-resistant bacteria. J Appl Microbiol. 2012;112(5):841-52. [PubMed ID: 22324439]. https://doi.org/10.1111/j.1365-2672.2012.05253.x.
-
42.
Potara M, Jakab E, Damert A, Popescu O, Canpean V, Astilean S. Synergistic antibacterial activity of chitosan-silver nanocomposites on Staphylococcus aureus. Nanotechnology. 2011;22(13):135101. [PubMed ID: 21343644]. https://doi.org/10.1088/0957-4484/22/13/135101.
-
43.
Panacek A, Smekalova M, Kilianova M, Prucek R, Bogdanova K, Vecerova R, et al. Strong and Nonspecific Synergistic Antibacterial Efficiency of Antibiotics Combined with Silver Nanoparticles at Very Low Concentrations Showing No Cytotoxic Effect. Molecules. 2015;21(1). E26. [PubMed ID: 26729075]. [PubMed Central ID: PMC6273824]. https://doi.org/10.3390/molecules21010026.
-
44.
Velusamy P, Das J, Pachaiappan R, Vaseeharan B, Pandian K. Greener approach for synthesis of antibacterial silver nanoparticles using aqueous solution of neem gum (Azadirachta indica L.). Ind Crops Prod. 2015;66:103-9. https://doi.org/10.1016/j.indcrop.2014.12.042.