Abstract
Background:
Consumption of hot substances may harm the surrounding bone around a dental implant. High temperatures at the bone-implant interface (BII) interferes with local cellular activities involved in the osteointegration.Objectives:
The present study was aimed at calculating the temperature distribution through the BII and the jaw bone under application of a transient cyclic thermal load.Methods:
In this numerical simulation, finite element method was employed in a commercialized dental implant model drawn by computer-aided design tools based on CT data to find the temperature in superficial and deep bone regions near the BII. The heat load was applied cyclically during the intake time.Results:
Results showed that the highest temperature was occurred at the top regions of the interface by magnitude of 48 C. Removal of the thermal loads also was followed by rapid decrease in the bone temperature.Conclusions:
Routine beverages of a hot liquid can increase the temperature of the bone beyond the biological thresholds of the bone cells vitality or remodeling functionality, specifically in the delayed loading types of implantation.Keywords
Dental Implant Thermal Analysis Finite Element Bone Remodeling
1. Background
Mechanical stability of dental implants is a prerequisite for successful implantation [1-3]. Disregarding the immediately- or delayed-loading types, dislocation of the implant may lead to failure to the replacement procedure and imposes clinical and financial costs to the patients. The stability of a dental implant closely relies on either the endogenic (like bone quality, vasculature, infective or inflammatory diseases, etc) or the exogenic (loading type, implant design, life style, etc) conditions [4-10]. Low bone quality is a most relevant reason that challenges the implantation success [11], and can reduce the implant stability due to the overall osteoporosis specifically for the aged patients or local lack of osteogenic cells at the bone-implant interface (BII) [12, 13].
Osteointegration around the implant plays an important role in provision of the stability. An increase in the bone density near the implantation site by activation of osteoblast cells results in higher anchorage of the implant and enhances the chance of survival. Naturally, the cells are recruited to form the bone unless in disorders [14], or severe external conditions e.g. mechanical over- or unloading of the implant [9], extreme heat exposing [15], etc. The heat exposing to the implant is daily and repetitive by beverage of hot liquids or eating hot foods which may adversely affect the osteointegration process. It was stated that the temperature threshold for necrosis of the bone is 47°C [16]. Also, the osteoblasts may be severely damaged by even a thermal impulse of 42°C [17]. Furthermore, an in vitro research has been performed to measure the generated heat at the BII caused by an exothermic setting reaction of two impression plasters and indicated that the temperature at the implant cervix increases more rather than the apex of the implant [18]. An experimental investigation performed by Feuerstein et al. (2007) to first established the maximum temperature produced intra-orally while consuming hot substances as 61.4 to 76.3°C and then applied these values constantly to an ex vivo model to find the changes in the BII temperature using temperature sensors. They found that the maximum temperature occurs near the abutment and the apical and mid-length BII receive relatively lower temperatures but exceed the vitality thresholds [19]. Rabbani Arshad et al. (2016) used a transient FE method to analyze the distribution of temperature through the dental implants with immediately- and delayed-loaded approaches. The former model assumed two materials (zirconia and ceramic) and the latter had no crown. Exposing these FE models to heat loads showed that the ceramic crown exhibits the diffusion of the heat through the deeper implant parts and the surrounding bone [15]. However, their calculated temperature magnitudes, specifically at the BII in delayed-loading implantation type (without crown), were considerably low in comparison with the experiments that applied the same condition [15].
2. Objectives
Therefore, the aim of the present study is to numerically analyze the time-dependent transfer of heat, originated from daily consumption of the hot substances, through the BII and then the surrounding bone. It was hypothesized that the usual intake of hot substances may impair the embedding bone and affect the osteointegration.
3. Methods
3.1. Model Reconstruction
It was assumed that one dental implant is inserted into the lower human jaw bone, the mandible, to replace the missed tooth. A commercialized screw-type dental implant based on 3.0 mm one-piece Zimmer implant was chosen to replace the first molar tooth using a three-dimensional computer-aided design (CAD). The abutment and the root was made of a same material because the implant type was one-piece. Therefore, the overall design incorporated two distinct parts i.e. the root and the occlusal part. Moreover, the mandibular part was reconstructed using a CAD software based on the images taken by CT. Two components of the cortical and trabecular bone which were primarily separated, have been united because the analysis considered the same thermal properties for both of them. Figure 1 demonstrates the meshed models of each part in an exploded view. The whole model included 139,483 elements and 28,014 nodes as well.
Meshed Model of the Mandibular Bone, the Merged Root and Abutment, and the Occlusal Part
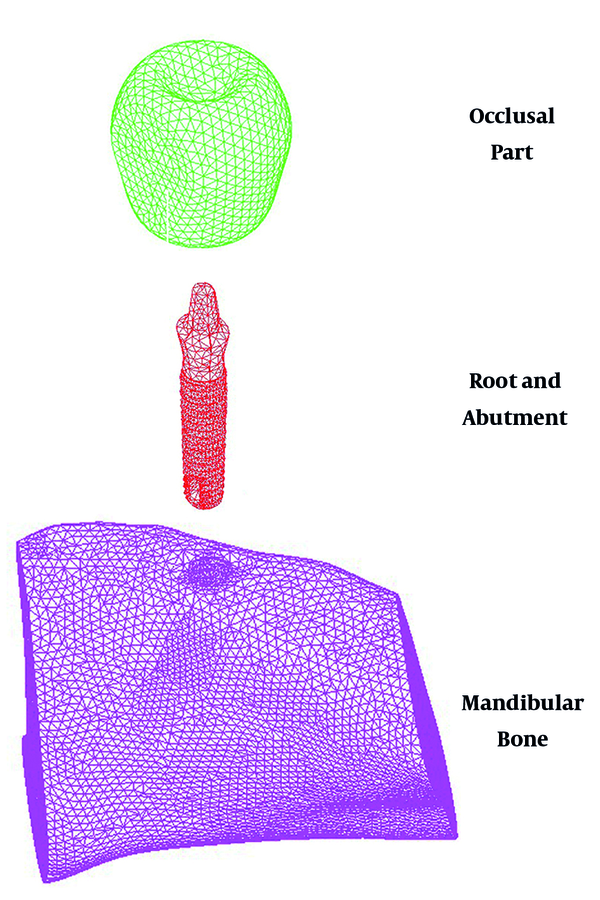
3.2. Governing Equations and Boundary Conditions
The thermal intake energy was conveyed to the dental implant by convection between the substance and outer surface of the occlusal part according to the equation

where q is the thermal flux, A the area of contact and h the convection coefficient. Also, T∞ stands for the temperature of oral cavity when the hot substance is consumed, and TS represents the temperature of the surface of interest, i.e. the outer surface of the occlusal part. The amount of the h was assumed to be constant, independent of the temperature changes equal to 50 × 103 J/(m2. s.°C) [20]. The term TS is also constant equal to the body temperature, 37°C. However, T∞ is the loading type as three 10-second duration saw-tooth pulses from 60 to 37°C with 5-second interval, as plotted in Figure 2. After these three cycles, the implant was relieved thermally. The heat transfers through the implant components and the bone by conduction following the equation

where x, y and z are components of the Cartesian coordinates, and, k, ρ and Cp represent the coefficient of thermal conductivity, density and specific heat for each material, respectively. In addition, T denotes the temperature and is a function of time and space, i.e. T ≡ T (x, y, z, t). The term q ̇ is the heat produced or absorbed and is zero because of no heat generation or dissipation. It should be noted that like the convection coefficient, other thermal properties appeared in equation 2 are all independent of the temperature changes.
Variation of the Oral Cavity Temperature Against Time
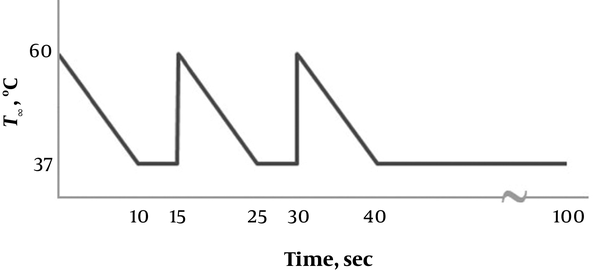
The temperature values of all the parts and the mandibular bone are initially held constant at 37°C. The analysis is carried out using finite element method in ADINA solver with transient solution criterion and Euler forward integration mode. Furthermore, in order to reach a reliable certainty about network-independent results, the solution process is repeated for different measures of elements and it was stated that the results are network-free.
3.3. Materials
The threaded root and the abutment were assumed to be made of titanium alloy. The occlusal part of the implant was also of bioceramic. The thermal properties of two bone components of cortical and trabecular were the same as well. Table 1 presents the thermal properties of these materials which are presumed to be homogeneous.
Thermo-Physical Properties of the Used Materials in the FE Analysisa
Material | ρ (Kg.m-3) | k (W.m-1.s-1) | Cp (J.kg-1.°C-1) |
---|---|---|---|
Bone | 1300 | 0.586 | 1350 |
Root | 4420 | 6.7 | 520 |
Occlusal Part | 3600 | 1.5 | 1070 |
4. Results
4.4. Temperature at the BII
In order to reach a justifiable peculiarity to assess the deteriorating effects of heat on the implant stability, the temperature variations at the BII is shown in Figure 3 for three marked points at the superficial (B1), middle (B2) and deep (B3) levels of the interface. Each cycle of the loading starts by a sharp rising in accordance with the loading pattern while the decrease is delayed for some seconds. Also, two curves of the superior points are roughly coincided. Finally, accumulative effect of the cycles is obvious for all the points. The deeper regions (middle and apical locations) gained lower amounts of the temperature than the coronal one due the distance to the heating source and also the capacitance of the passing materials.
Marked Points at Symmetric Cut of the Bone-Implant Interface, and, Temperature Changes by Time for the Three Marked Points
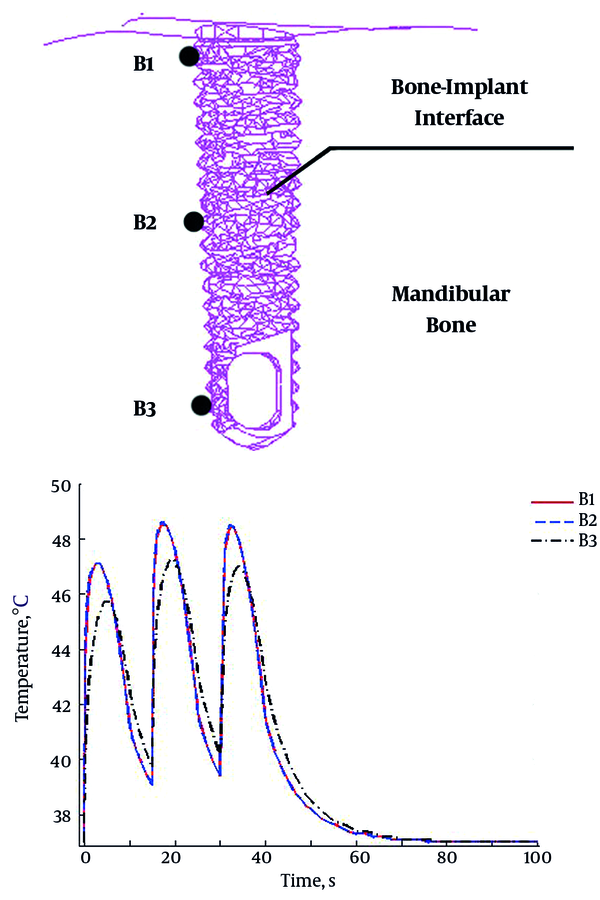
4.5. Heat Transfer to the Bone
Transfer of the thermal energy through the surrounding mandibular bone is depicted in Figure 4. The regions located in the vicinity to the BII receive higher amounts of the temperature. Temperature of the occlusal part decreases after 3 seconds and finally returns to the initial temperature i.e. 37°C. The bone-implant interface experiences maximal temperature a little bit more than 48°C.
Temperature Distribution in a Cut View of the Model at Six Selected Times in the First Cycle of Thermal Loading.
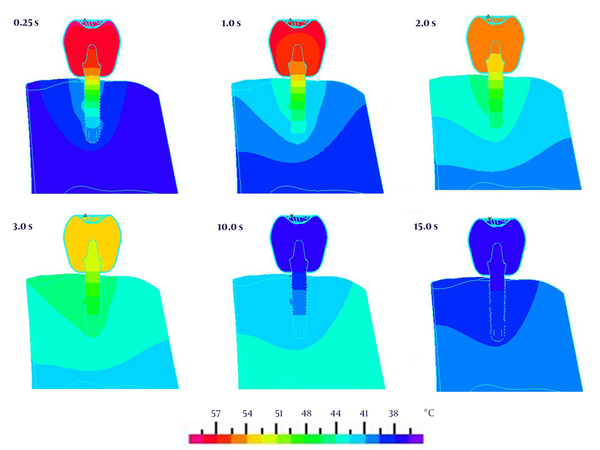
5. Discussion
The present study investigated the transfer of heat from repetitive hot substance consumption through a commercialized dental implant using the finite element analysis. The main goal was to inspect that whether the temperature rise in the surrounding bone exceeds the cell mortality threshold to interfere with the osteointegration.
It was established that the bone quality specifically around the interface is closely related to the implant stability [21]. Bone remodeling by recruitment of the osteoblasts can enhance the density of cortical or trabecular parts of the jaw [9]. Besides the mechanical stimulation or deactivation of the osteoblasts, the thermal loads like beverage of a hot liquid may extremely increase in the temperature values received by the bone. The implant titanium root which is thermally conductive in nature, rapidly transferred the heat fluxed from the heat sources i.e. the upper occlusal part. But the input heat flux to the root is not necessarily the same as it was in the ceramic occlusal part. The high specific heat coefficient and low conductivity of the ceramic serve as a barrier against the transfer of heat into the lower parts. The Point B1 and B2 which were located on the coronal and middle region of the BII receive the same rise in the temperature and pass over the 48°C limit. It should be noted that the occlusal part was thermally loaded by 60°C peak temperatures. Such a difference between the top and bottom of the occlusal part was due to the isolative character of the implant ceramic crown. It is noteworthy to mention that in the cases of delayed loading implantation in which the occlusal part is placed above the abutment after few months, the thermal loads may menace the cellular vitality, and hence, the osteointegration. Although the immediate loading type of the implantation may impose early and extreme forces to the interface, presence of a ceramic crown above the metal root can prohibit the bone damages due to the heat loads.
There was a great deal in the literature that have investigated the transfer of the heat through the natural tooth structure (see review paper [22], restorative materials [23-26] or effects of drilling or laser heat generation [27, 28], but the studies on the implant heat transfer due to hot beverage was limited. These previous studies, though different in the boundary conditions, concluded controversial results. For instance, Rabbani Arshad et al. (2016) calculated the temperature of the deeper regions lower than the vitality limits. Even in the delayed-loaded cases that the occlusal part was not mounted which the heat source was directly in contact to the abutment and root, their FE model showed temperature as 38.8°C. This value seemed an underestimation of the heating load’s effect. An ex vivo study performed by Feuerstein et al. (2008) measured the implant apical temperature with a roughly same heat load near 45°C. The present study also found the maximum temperature equal to 47°C at maximum for the implant apical region (point B3). Ormianer et al. (2009) also measured the maximum temperatures at three points on the implant. But the in vivo nature of the experiment could not allow the researchers to measure the temperature at the interface. Some of the temperature sensors registered values above 42°C but only on the abutment of the implant [29]. In an experimental study with an implant within a plastic replicate of the bone, Livne et al. (2014) exposed a 60°C hot liquid to the model and measured different points on the implant. They found that the temperature at the interface may be increased near 48°C [30], which is well-coincided with the present results. The main reason for this agreement could be the same heat source conditions, same measuring location or even the similar implant design.
Both the occlusal part and the upper regions of the root returned to the initial temperature after 10 seconds which is the end point of first loading cycle. The next loading cycle starts after 15 seconds in which the upper parts are relaxed at 37°C while the deeper regions were still more than 37°C. This residual temperature in the bone may be the reason underlies the increase in temperatures of the next cycles. The cyclic loads with small rest time between them could increase the temperature at the BII more than 48°C but rapidly decreased after unloading. The marked points at the BII after the third load cycle were confronted with a dramatic fall to 37°C. Repetitive exposure of the dental implant to the heat has kept the temperature in a high level. Having immediately removed the heat source, the BII temperature descended to 37°C. The higher frequency of the beverage could further deteriorate the osteointegration conditions by keeping the bone temperature level up.
The deeper regions of the interface received lower amounts of the temperature below 48°C but greater than 42°C. All three points may be at risk of interference with the osteointegration (> 42°C) which threatened the overall implant stability. Two upper points experienced higher temperature levels due to their vicinity to the heat source. These locations which revealed similar behaviors were also at risk of the bone cell death (> 47°C). The deeper point (B3 in Figure 3) also had a time delay with respect to the superficial ones. The transient transfer of heat into the deeper bone regions took a few seconds. The main obstacle may be the occlusal part which possesses approximately 4.5 times smaller heat conductivity than the titanium root.
The present research was confronted with some limitations. The overriding was the absence of the neighbor teeth or implants. The jaw was modeled as a bulk of bone which receives the heat flux merely from one thermally-loaded prosthesis. Consideration of the flux of other teeth even in an intact form could further increase the bone temperature. Also the thermal or physical properties of the model were assumed for the whole components while these are not homogenous in reality.
In conclusion, finite element analysis on cyclic heat transfer in a commercialized model of dental implant showed that the routine beverage of hot substances near 60°C may induce higher temperatures at the bone-implant interface. The cyclic heat loads increased the bone temperature beyond the vital and remodeling functional limits. Consumption of hot liquids in delayed loading implantations before placement of the occlusal part can reduce the implant stability.
Acknowledgements
References
-
1.
Branemark PI, Svensson B, van Steenberghe D. Ten-year survival rates of fixed prostheses on four or six implants ad modum Branemark in full edentulism. Clin Oral Implants Res. 1995;6(4):227-31. [PubMed ID: 8603114].
-
2.
Dixon DL, Breeding LC, Sadler JP, McKay ML. Comparison of screw loosening, rotation, and deflection among three implant designs. J Prosthet Dent. 1995;74(3):270-8. https://doi.org/10.1016/s0022-3913(05)80134-9.
-
3.
Pye AD, Lockhart DE, Dawson MP, Murray CA, Smith AJ. A review of dental implants and infection. J Hosp Infect. 2009;72(2):104-10. [PubMed ID: 19329223]. https://doi.org/10.1016/j.jhin.2009.02.010.
-
4.
Jansen VK, Conrads G, Richter EJ. Microbial leakage and marginal fit of the implant-abutment interface. Int J Oral Maxillofac Implants. 1997;12(4):527-40. [PubMed ID: 9274082].
-
5.
Mombelli A, Lang NP. The diagnosis and treatment of peri-implantitis. Periodontol 2000. 1998;17:63-76. [PubMed ID: 10337314].
-
6.
Brunski JB, Puleo DA, Nanci A. Biomaterials and biomechanics of oral and maxillofacial implants: current status and future developments. Int J Oral Maxillofac Implants. 2000;15(1):15-46. [PubMed ID: 10697938].
-
7.
O'Sullivan D, Sennerby L, Meredith N. Influence of implant taper on the primary and secondary stability of osseointegrated titanium implants. Clin Oral Implants Res. 2004;15(4):474-80. [PubMed ID: 15248883]. https://doi.org/10.1111/j.1600-0501.2004.01041.x.
-
8.
Schouten C, Meijer GJ, Van Den Beucken JJ, Spauwen PH, Jansen JA. The quantitative assessment of peri-implant bone responses using histomorphometry and micro-computed tomography. Biomaterials. 2009;30(27):4539-49. [PubMed ID: 19500840]. https://doi.org/10.1016/j.biomaterials.2009.05.017.
-
9.
Lin D, Li Q, Li W, Duckmanton N, Swain M. Mandibular bone remodeling induced by dental implant. J Biomech. 2010;43(2):287-93. [PubMed ID: 19815211]. https://doi.org/10.1016/j.jbiomech.2009.08.024.
-
10.
Bahrami B, Shahrbaf S, Mirzakouchaki B, Ghalichi F, Ashtiani M, Martin N. Effect of surface treatment on stress distribution in immediately loaded dental implants a 3D finite element analysis. Dent Mater. 2014;30(4):89-97. [PubMed ID: 24559526]. https://doi.org/10.1016/j.dental.2014.01.012.
-
11.
Molly L. Bone density and primary stability in implant therapy. Clin Oral Implants Res. 2006;17 Suppl 2:124-35. [PubMed ID: 16968388]. https://doi.org/10.1111/j.1600-0501.2006.01356.x.
-
12.
Soncini M, Rodriguez y Baena R, Pietrabissa R, Quaglini V, Rizzo S, Zaffe D. Experimental procedure for the evaluation of the mechanical properties of the bone surrounding dental implants. Biomaterials. 2002;23(1):9-17. [PubMed ID: 11762859].
-
13.
Ikebe K, Wada M, Kagawa R, Maeda Y. Is old age a risk factor for dental implants? Jpn Dent Sci Rev. 2009;45(1):59-64. https://doi.org/10.1016/j.jdsr.2009.02.001.
-
14.
Wood MR, Vermilyea SG, Committee on Research in Fixed Prosthodontics of the Academy of Fixed P. A review of selected dental literature on evidence-based treatment planning for dental implants: report of the Committee on Research in Fixed Prosthodontics of the Academy of Fixed Prosthodontics. J Prosthet Dent. 2004;92(5):447-62. [PubMed ID: 15523334]. https://doi.org/10.1016/S0022391304005207.
-
15.
Rabbani Arshad S, Zoljanahi Oskui I, Hashemi A. Thermal analysis of dental implants in mandibular premolar region: 3D FEM study. J Prosthodont. 2016. [PubMed ID: 27078043]. https://doi.org/10.1111/jopr.12486.
-
16.
Eriksson AR, Albrektsson T. Temperature threshold levels for heat-induced bone tissue injury: a vital-microscopic study in the rabbit. J Prosthet Dent. 1983;50(1):101-7. [PubMed ID: 6576145].
-
17.
Li S, Chien S, Branemark PI. Heat shock-induced necrosis and apoptosis in osteoblasts. J Orthop Res. 1999;17(6):891-9. [PubMed ID: 10632456]. https://doi.org/10.1002/jor.1100170614.
-
18.
Nissan J, Assif D, Gross MD, Yaffe A, Binderman I. Effect of low intensity laser irradiation on surgically created bony defects in rats. J Oral Rehabil. 2006;33(8):619-924. [PubMed ID: 16856960]. https://doi.org/10.1111/j.1365-2842.2006.01601.x.
-
19.
Feuerstein O, Zeichner K, Imbari C, Ormianer Z, Samet N, Weiss EI. Temperature changes in dental implants following exposure to hot substances in an ex vivo model. Clin Oral Implants Res. 2008;19(6):629-33. [PubMed ID: 18371098]. https://doi.org/10.1111/j.1600-0501.2007.01502.x.
-
20.
Oskui IZ, Ashtiani MN, Hashemi A, Jafarzadeh H. Thermal analysis of the intact mandibular premolar: a finite element analysis. Int Endod J. 2013;46(9):841-6. [PubMed ID: 23480124]. https://doi.org/10.1111/iej.12069.
-
21.
McCracken M, Lemons JE, Jeffcoat M, Koth DL, Fritz ME. Histomorphological evaluation of loaded plate-form and root-form implants in Macaca mulatta monkeys. Clin Oral Implants Res. 2002;13(2):214-20. [PubMed ID: 11952742].
-
22.
Lin M, Xu F, Lu TJ, Bai BF. A review of heat transfer in human tooth--experimental characterization and mathematical modeling. Dent Mater. 2010;26(6):501-13. [PubMed ID: 20303579]. https://doi.org/10.1016/j.dental.2010.02.009.
-
23.
Gale MS, Darvell BW. Thermal cycling procedures for laboratory testing of dental restorations. J Dent. 1999;27(2):89-99. https://doi.org/10.1016/s0300-5712(98)00037-2.
-
24.
Toparli M, Sasaki S. Finite element analysis of the temperature and thermal stress in a postrestored tooth. J Oral Rehabil. 2003;30(9):921-6. [PubMed ID: 12950974].
-
25.
Huang MS, Li MT, Huang FM, Ding SJ. The effect of thermocycling and dentine pre-treatment on the durability of the bond between composite resin and dentine. J Oral Rehabil. 2004;31(5):492-9. [PubMed ID: 15140176]. https://doi.org/10.1111/j.1365-2842.2004.01272.x.
-
26.
Ayatollahi MR, Yahya MY, Karimzadeh A, Nikkhooyifar M, Ayob A. Effects of temperature change and beverage on mechanical and tribological properties of dental restorative composites. Mater Sci Eng C Mater Biol Appl. 2015;54:69-75. [PubMed ID: 26046269]. https://doi.org/10.1016/j.msec.2015.05.004.
-
27.
Gehrke SA, Bettach R, Taschieri S, Boukhris G, Corbella S, Del Fabbro M. Temperature Changes in Cortical Bone after Implant Site Preparation Using a Single Bur versus Multiple Drilling Steps: An In Vitro Investigation. Clin Implant Dent Relat Res. 2015;17(4):700-7. [PubMed ID: 24215668]. https://doi.org/10.1111/cid.12172.
-
28.
Monzavi A, Shahabi S, Fekrazad R, Behruzi R, Chiniforush N. Implant Surface Temperature Changes during Er:YAG Laser Irradiation with Different Cooling Systems. J Dent (Tehran). 2014;11(2):210-5. [PubMed ID: 24910697].
-
29.
Ormianer Z, Feuerstein O, Assad R, Samet N, Weiss EI. In vivo changes in dental implant temperatures during hot beverage intake: a pilot study. Implant Dent. 2009;18(1):38-45. [PubMed ID: 19212236]. https://doi.org/10.1097/ID.0b013e318192e143.
-
30.
Livne S, Harel N, Piek D, Ormianer Z. Evaluation of heat conduction in dental implants after exposure to hot beverages. J Prosthet Dent. 2014;111(3):228-33. [PubMed ID: 24262947]. https://doi.org/10.1016/j.prosdent.2013.10.003.