Abstract
Background:
The current study aims to investigate the impacts of aerobic exercise with pumpkin seed oil and white pea extract supplementation on the gene expression of the liver zinc transporters of healthy male Wistar rats.Methods:
In this experimental study, 60 male Wistar rats (130 - 150 g, aged 4-6 weeks old) were divided into 6 groups (n = 10/groups) by randomization. These groups include (1) saline control, (2) saline training, (3) pumpkin seed oil control, (4) pumpkin seed oil training, (5) control-pea, and (6) training-pea. The aerobic exercise protocol consisted of running at a velocity of 25 m/min, 5 days a week and for 6 weeks. Supplement groups received 4 ml/kg/day of pumpkin seed oil and 2 g/kg/day of white pea extract through oral gavage. Gene expression levels of ZnT5, ZnT9, and ZIP14 of liver tissue were determined through real-time PCR, and serum levels of Zn were measured using the ICP-EOS technique approximately 48 hours after the final session of the training program. Data were analyzed by one-way ANOVA and Tukey post-hoc tests (P < 0.05).Results:
Regarding the results of one-way ANOVA, there were no significant changes in serum Zn levels (P = 0.418) as well as gene expression levels of ZnT5 and ZIP14 among intervention groups (P = 0.586 and 0.677, respectively), but ZnT9 gene expression level was significantly changed among the study groups (P = 0.029). Also, the results of the Tukey test showed that six weeks of aerobic exercise along with pumpkin seed oil supplementation had the greatest effect on ZnT5 gene expression.Conclusions:
Findings showed that aerobic training along with pumpkin seed oil supplementation had the greatest impact on the up-regulation of ZnT5 as a Zn transporter to retain the blood Zn levels in healthy trained rats, suggesting that pumpkin seed oil can be an effective ergogenic aid for improving circulatory Zn levels.Keywords
1. Background
In addition to being an essential micronutrient (1), zinc is the second most abundant trace element (after iron) that contributes to a wide range of biological, physiological, and developmental processes in humans and animals, including gene expression, differentiation, proliferation, and signal transmission. Additionally, there is a close relationship between plasma zinc levels and energy availability since zinc is required for the activity of several enzymes in energy metabolism. Meanwhile, several factors, including exercise, influence zinc concentration in the circulation. A drop in plasma zinc level may result in a decrease in concentration in muscle zinc content (2) that might lead to the reduction of endurance capacity (3), impaired performance, and biological function perturbance (4).
Zinc absorption starts from intestinal cells, where enterocytes absorb zinc, which is then processed by the liver as a primary organ for its metabolism. The liver is integral to systemic zinc metabolism by managing a zinc reservoir that can be quickly exchanged with plasma and other tissues (5). Due to the central function of the liver, changes in zinc status directly alter the gene expression of zinc transporters, which in turn affects other organs' metabolisms (6). Zn entry and exit processes occur through cell zinc transporters under steady-state conditions, preventing zinc deficiencies and excesses from affecting physiological functions (7). Each zinc transporter has a specific tissue expression pattern, growth model, and cellular response to various stimuli, such as zinc deficiency or surplus. The zinc transporters in mammals are classified into SLC39A (also known as Zrt and Irt or ZIP proteins) and SLC30A (ZnT). Currently, 14 ZIP transporters have been identified in mammals that increase cytosolic zinc content by facilitating extracellular absorption or release of zinc. The liver is the major organ where ZIP14 is primarily expressed (7). The metabolism of zinc is adversely affected by liver inflammation (8, 9). Inflammation-induced hypozincemia caused by inflammatory cytokines affects several metabolic and nutritional functions. (8). ZIP14 is one of the key mediators of hypozincemia associated with acute inflammation. Under pro-inflammatory conditions, ZIP14 increases in response to IL-6 and nitric oxide. It is reported that IL-6 down-regulates ZnT-10 expression while increasing ZIP14 expression (10, 11). Also, Activated ZnT9 is one of the 10 members of the SLC30A family so far identified in mammals (3, 12). This transporter reduces cytoplasmic zinc by leaving zinc outside of the cell or inserting zinc into vesicles (3). It is important to understand the physiological significance of ZnT9 despite the lack of research on it. Another essential milestone of zinc transport that is a primary component of the secretory pathway is ZnT5. A growing body of evidence suggests that zinc-oriented intracellular events, such as transcription, translation, enzyme activity, and some signaling pathways, are handled by the ZnT5 transporter. The ZnT5-ZnT6 heterodimers play a key role in zinc biosynthesis by transferring zinc to the primary secretory pathway, which activates zinc-dependent enzymes such as alkaline phosphatase (13).
Zinc concentrations in plasma and serum increase immediately following short-term and high-intensity activities, but hypozincemia associated with long-term exercise and heat exposure might lead to eliminating Zn through sweating or urination (14). Therefore, it has been suggested that physically active people should consume a balanced diet to ensure adequate zinc intake for optimal health and physical performance. In this regard, consuming edible seeds and legumes, considering the possible side effects of zinc supplements, might safely replenish Zn pools. Chickpeas, scientifically known as Cicer arietinum, are among legume families of foods with low glycemic index, along with dietary fiber, antioxidants, phytochemicals, and mineral content (15) that contains approximately (0.5 mg/100 g) iron, (1.4 mg/g100) zinc, (138mg/100 g) magnesium and (160 mg/100 g) calcium. It has been reported that daily consumption of 100 grams of chickpea seeds might supply daily zinc requirements (16). Pumpkin seed oil, scientifically known as (Cucurbita pepo L.), is another food supplement made from pumpkin seeds. There is extensive ethnopharmacological evidence that pumpkin seeds have anti-diabetic and antioxidant properties. Proteins, phytosterols, polyunsaturated fatty acids, zinc, and selenium can be found in pumpkin seeds and oil. Additionally, pumpkin seeds are abundant in magnesium, iron, calcium, phosphorus, vitamins A, B, and E, and folic acid (17).
2. Objectives
Despite all new knowledge on complex Zn dynamics in tissues and body fluids, linking exercise training with oxidative stress-induced zinc deficiency, the puzzle is still incomplete, and several pieces of information about transporters and their roles in the liver seem missing. We hypothesize that adopting white pea extraction and pumpkin seed oil may replenish Zn deficiency. Therefore, we aimed to investigate the effects of aerobic exercise and supplementation with pumpkin seed and white peas on serum Zn levels and liver tissue gene expression of ZnT9,5 and ZIP14.
3. Methods
In this experimental study, 60 male Wistar rats aged 4 - 6 weeks and weighing 130 - 150 g were obtained from Babol University of Medical Sciences. Animals had free access to water and standard chow (comprising 54% mixed carbohydrate, 19% protein, and 3% lipid) before and during the experiment. After being delivered to our laboratory animal experiment site, rats were given a week to acclimate to their new environment (24 ± 1 °C, humidity of 45% to 55%, 12:12 dark/light cycle). Following this period, the rodents were assigned into 6 groups (n = 10/groups), including (1) saline control, (2) saline training, (3) pumpkin seed oil control, (4) pumpkin seed oil training, (5) control-pea, and (6) training-pea. The body weight and food intake were recorded every week. The Guide for the Care and Use of Laboratory Animals (18) authorized by the University of Mazandaran Ethics Committee (IR.UMZ.REC.1401.078) served as the foundation for the care of animals and the ethical standards that governed animal treatment.
3.1. Training Protocol
Before starting the training protocol, the rats underwent one week of adaptation to running on the treadmill thrice weekly, lasting 10 minutes per session. After the familiarization period, aerobic exercise groups performed 60 minutes of running at a velocity of 25 m/min on a motorized treadmill (Iranian Model, 14 lanes, designed by Prof. Abbass Ghanbari-Niaki, Faculty of Sports, University of Mazandaran, Mazandaran, Iran), five days a week and for six weeks. Running was implemented all weekdays except for Thursday and Friday. The subjects started running at a speed of 10 m/min and gradually reached a speed of 25 m/min. Apart from the main activity time, 5 minutes for warm-up and cool-down were considered. The training protocol in the present study was a moderate-intensity training program originating from two modified protocols of Howarth et al. (19) and Ghanbari Niaki (20). The intensity of the training protocol was considered based on the maximum oxygen consumption of 65% at a medium-intensity level (21). To prevent the possible effect of electric shock on the research findings, during the familiarization stage, the animals were taught to avoid approaching and resting at the end part of the treadmill by conditioning to sound an alarm. The exercise training program was done in the active (dark cycle) cycle. To reduce the stress of the cages, the sedentary rats were kept out of the cages on a stationary treadmill for the same period of exercise as the training groups.
3.2. Pumpkin Seed Oil and White Pea Supplementation
3.2.1. Extraction
Briefly, the huskless pumpkin seeds were grounded and poured into the decanter funnel with Hexane solvent with a ratio of 50% water. After 12 hours of solvent contact with pumpkin seeds and white peas, the solvent’s oil phase and water were removed from the funnel, and the de-oiled residue was poured into the percolator for extraction. Then, the required amount of solvent (60% alcohol) was poured to cover the entire surface of the oiled material. The solvent was in contact with pumpkin seeds for 72 hours. Then, by filtering the mixture through a clean Whatman paper, the hydro-alcoholic extract was separated, and the solvent was removed by a rotary at a mild temperature (below 60 degrees Celsius) (15). Weighing up the concentrated extract allowed us to determine the pure quantity of material.
3.2.2. Dosage
Supplement groups received 4 ml/kg/day of pumpkin seed (cold-pressed) oil and 2 g/kg/day of white pea flour extraction through oral gavage. The control groups also received saline once a week with the same calculated ratio to equalize the gavage stress. Re-weighting was performed weekly to determine the exact dose per kilogram of body weight (22, 23).
3.2.3. Tissue Extraction and Blood Collection
Following a six-week treatment period, the rats were subjected to a fasting condition lasting 12 hours. Subsequently, they were anesthetized with an intraperitoneal injection containing a mixture of ketamine and xylazine, administered at doses of 80 and 12 mg/kg, respectively. This anesthesia was administered 48 hours after the final exercise session. Blood samples were collected from the heart and placed under centrifugation at a speed of 2500 revolutions per minute for 15 minutes at a temperature of 4 degrees Celsius. The resulting plasma was then stored at a temperature of -20 degrees Celsius until measurements for zinc were conducted. The liver tissue was surgically removed and promptly preserved by freezing with liquid nitrogen. It was then maintained at -80°C until it was ready for real-time polymerase chain reaction (PCR) analysis (24).
3.2.4. RT-PCR Analysis
According to the supplier's methodology, total RNA was briefly extracted from the liver tissue using a total RNA extraction micro kit (Denazist, Iran). After that, the materials were stripped of any potential DNA using a DNase I Kit from Sinaclon, Iran. Using MMLV reverse transcriptase (YTA, Iran), oligo (dT) primer, and dNTP mix in accordance with the manufacturer's instructions, first-strand cDNA was produced from RNA samples. Using Primer Premier 5, specific primers (ZnT9, ZnT5, ZIP14, GAPDH, and -actin) were created for this study. Table 1 lists the primer sequences that were utilized in this study. Real-time polymerase chain reaction (PCR) was carried out using Rotor-Gene 6000 (Corbett Research, Australia) and RealQ Plus 2x Master Mix Green (Ampliqon, Denmark). The thermal cycling protocol consisted of an initial denaturation step at 95 °C for 15 minutes, followed by 40 cycles of denaturation at 95°C for 30 seconds, annealing at 57 °C for 30 seconds, and extension at 72°C for 20 seconds. The expression level of each sample was standardized by comparing it to the expression of β-actin, which served as an appropriate internal reference. The relative expression level was determined by the comparative CT method 2−ΔΔCT (25).
The Sequences of Forward and Reverse Primer of RT-PCR
Gene | Reverse and Forward Primer (5’-3’) | NCBI Accession Number | Amplicon Size |
---|---|---|---|
ZnT9 | NM_001130538.1 | 100 | |
F | GCCTGTAATCCCAGCGACC | ||
R | TGATGGTACTTTTTCCACTCTGA | ||
ZIP5 | NM_001011952.1 | 165 | |
F | ATAAAGAAGTCGTATTTCCCCAAGA | ||
R | GTAAAATCCACCAAACACAGCAAC | ||
ZIP14 | NM_030826.4 | 146 | |
F | AGTGCGAGGTGAATGGTGAGA | ||
R | CCAGGAAATGTCGTTGCG | ||
GAPDH | NM_012520.2 | 147 | |
F | ATCAGGTTACTTTCTTGTTCAGCG | ||
R | TGATGCCCTGGTCAGTCTTG | ||
β-Actin | NM_031144.3 | 119 | |
F | GTGTGACGTTGACATCCGTAAAGAC | ||
R | TGCTAGGAGCCAGGGCAGTAAT |
3.2.5. Serum Zinc Assessment
Water and nitric acid solution were mixed in the proportion of 93% to 7%, and then 20-fold of the prepared solution was added to the serum. Finally, serum values were measured using the ICP-EOS technique in the laboratory (26).
3.2.6. Statistical Analysis
All data were given as means ± standard deviations. One-way ANOVA was used to compare groups, followed by the Tukey post-hoc test. The threshold for statistical significance was set at P < 0.05. SPSS statistical software (Version 22) was used for the statistical analysis.
4. Results
The analysis of variance (ANOVA) indicated that six weeks of white pea and pumpkin seed oil supplementation combined with aerobic exercise resulted in a statistically significant difference in the ZnT9 gene expression levels (P = 0.029) among the study groups. Still, there was no significant difference between the study groups in the gene expression of ZIP14 and ZnT5 (Table 2 and Figure 1).
Control | Training | Pea | Training + Pea | Pumpkin | Training + Pumpkin | P-Value | |
---|---|---|---|---|---|---|---|
ZnT9 | 1.008 ± 0.291 ** | 1.280 ± 0.458 | 1.310 ± 0.505 | 1.018 ± 0.865 ** | 1.440 ± 0.450 | 1.981 ± 0.265 ** | 0.029 * |
ZnT5 | 1.007 ± 0.385 | 1.002 ± 0.330 | 0.761 ± 0.452 | 1.146 ± 0.605 | 1.125 ± 0.232 | 1.180 ± 0.310 | 0.586 |
ZIP14 | 1.007 ± 0.269 | 1.143 ± 0.401 | 1.300 ± 1.094 | 1.576 ± 0.864 | 1.376 ± 0.162 | 1.520 ± 0.430 | 0.677 |
Zinc | 0.117 ± 0.054 | 0.100 ± 0.026 | 0.085 ± 0.011 | 0.090 ± 0.011 | 0.085 ± 0.004 | 0.095 ± 0.029 | 0.418 |
Tukey results for liver ZnT9, ZnT5, and ZIP14 gene expression and serum Zn levels. According to Tukey, data is expressed as Mean ± SD. a versus the control group; b versus the training group; c versus the pumpkin and pea group; d versus training-pea and training-pumpkin.
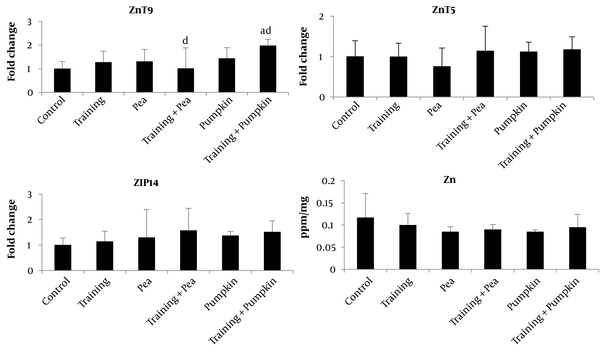
The results of the Tukey post-hoc test showed that six weeks of pumpkin seed oil supplementation combined with aerobic exercise led to an increase in gene expression of ZnT9 in comparison to the control group (P = 0.031), but other interventions (Training: P = 0.834; Pea: P = 0.451; Trainoing + pea: P = 1; Punpkin: P = 0.134) did not alter the ZnT9 gene expression (Figure 1). Overall, exercise training accompanied by pumpkin seed oil supplementation up-regulated the Znt9 gene expression in the liver tissue (Figure 1).
5. Discussion
According to the results, aerobic exercise combined with pumpkin seed oil and white pea supplementation had no significant effect on ZIP14 and ZnT5 gene expression in liver tissue and serum zinc levels. Pumpkin oil + training increased ZIP14 and ZnT5 gene changes compared to the other groups, and serum zinc levels almost decreased. However, these changes were not significant. Unfortunately, due to the lack of studies in the field of current research and liver tissue, we refer to the study of Liu et al., who examined the expression indices of SLC39A/ZIP7, ZIP8, and ZIP14 in response to exercise-induced skeletal muscle injury and found that zinc transporters including SLC39A/ZIP7, ZIP8, and ZIP14 shown different expression levels in eccentric exercises and may take part in the processes of muscle growth or differentiation via many cellular mechanisms of pathways after damage caused by exercise (7) that is inconsistent with the results of the present research. The reason for this difference might be due to the differences in the type of muscle tissue and the amount of exercise-induced stress on the muscle.
Liu et al. examined muscle tissue as the target tissue of their research. They found that muscles responded acutely to a single-session training protocol, but the present study included 6 weeks of training adaptation, which may explain the inconsistency between the results. While studies reported increased plasma zinc immediately after exercise, a decrease in plasma zinc concentration occurred during exercise recovery (7). The zinc clearance observed during exercise recovery was attributed to local exercise-induced muscle inflammation and its consequences (3). Furthermore, as part of peroxidase and anti-inflammatory peptides, zinc might be key in decreasing exercise-induced oxidative stress and inflammation. According to one study, after aerobic exercise in which the subjects reached maximum aerobic capacity, zinc was transferred from plasma to interstitial fluid and the liver, possibly due to the acute phase response or changes in osmotic pressure (27). Additionally, many cells do not follow the same pattern. For example, certain types of neurons and prostate cells accumulate higher levels of zinc than other tissues, suggesting a specialized role for zinc in these particular cell types (28).
Unlike other studies with an acute training phase, the results of our study showed that serum Zn content wasn’t eliminated in response to six weeks of sole aerobic training protocol or supplementation with pumpkin/pea seed. This would be due to several mechanisms, such as a decreased rate of muscle degradation due to muscle adaptation to exercise that can prevent Zn excretion and perturbation of mineral balance in body fluids. Results from a study by Dressendorfer and Sokolov showed that twenty-three percent of the runners had zinc concentrations less than 11.5 pmol/L, the lower limit of the range of normal serum zinc values. Also, serum zinc concentrations were inversely related to training mileage (29). According to a meta-analysis study, serum zinc levels exhibited a decline during the recovery process following aerobic activity. Apart from fitness level, training protocol, the timing of blood collection, and the alterations in serum levels observed throughout the recovery period following exercise may potentially be associated with cellular processes (30). Muscle damage and inflammation after exercise change osmotic pressure. This leads to zinc ions being transferred from the plasma into the interstitial fluid and liver while inflammation develops in the body. The liver plays a crucial role in controlling zinc sources in the body and regulating zinc exchange between tissues. Zinc may have been sequestered in the liver due to the simultaneous appearance of inflammatory cytokines and myokines. Even though our study did not reveal any significant decreases in zinc levels in the serum, this trend may be due to a redistribution of zinc in the blood due to the increased zinc requirements for the entire body because of inflammation and recovery after exercise (7). As inflammatory cytokines and myokines, such as interleukin-1 and IL-6, are released simultaneously, zinc accumulates in the liver, and circulating zinc decreases (30). Inflammatory cytokines such as IL-6 are produced during acute exercise stress, and these cytokines increase hepatic metallothionein and differentially regulate zinc transporters (3). According to research, pro-inflammatory cytokines such as IL-6 regulate ZIP-14, a zinc transporter that most likely contributes to hypoxemia (31).
There is a close relationship between metallothionein, an acute phase protein, interleukin-6 (IL-6), and zinc. In the acute-phase response, IL-6, the most important proinflammatory cytokine, contributes to zinc deficiency by upregulating ZIP14 in the liver (31). It has been shown that the signaling pathways activated by nitric oxide contribute to the upregulation of ZIP14, which leads to hepatic zinc accumulation and hypozincemia (32). The specific physiological functions of ZIP14 are still uncertain. It was observed that seven days of psychological stress (PS) significantly increased ZIP14 expression in a way that PS increased IL-6 levels. According to these findings, ZIP14, an IL-6-responsive zinc transporter that facilitates the transport of extracellular zinc into the cytosol, is upregulated by IL-6 in response to PS, resulting in an increase in zinc concentration in the liver. During 14 days of PS exposure, ZIP14 levels reached normal levels, indicating that Zn accumulated in the liver due to PS exposure (32). Therefore, the expression of ZIP14 is increased in pro-inflammatory states, especially when IL-6 and nitric oxide levels are elevated (31). In the acute phase, IL-6 increases ZIP14 expression in the liver, which increases zinc uptake. As a result, we found that ZIP14 did not significantly increase in adaptation to training with supplements compared to the control group. It then returned to its stable and normal initial state, consistent with regulating pro-inflammatory stimuli (7).
In this study, two natural zinc-rich food supplements were used to increase the amount of zinc in the body and, hence, the expression of zinc transporters. As compared to the saline group, pumpkin seed oil increased the expression of this transporter. The pumpkin control and pumpkin training groups showed an increase in ZnT5 expression compared to the control and training groups, but this increase was not significant. In addition, pumpkin training demonstrated a non-significant increase compared to pumpkin control. In addition, this increase was not significant in the training-pea group compared to the control group. Due to the zinc-rich contents of both supplements, zinc circulatory levels increased, and consequently, the intracellular transporter ZnT5 increased, but it was not significant. Pumpkin supplementation was more effective than chickpea supplementation in enhancing ZnT5 gene expression. These two supplements have not been studied for their effect on zinc transporters, and only limited studies have found their effect on the amount of zinc and other ZnT transporters (33). An experiment on rats with low zinc intake (30 mg zinc/kg body weight) found that ZnT expression in the kidney and small intestine was lower than in rats with high zinc intake (35 mg zinc/kg). The 180 mg/kg dietary Zn increased ZnT1 and ZnT2 in these tissues, while ZnT4 remained unchanged. According to these findings, metal-responsive elements (MREs) in ZnT1 and ZnT2 promoters are involved in metal-responsive gene regulation. Metallothionein induction by metals is carried out by MREs located in the promoter region of MT genes that recruit the metal response element transcription factor (MTF-1) and regulate its transcription. Many metal-responsive elements (MREs) in the ZnT1 promoter and several MREs in the ZnT5 promoter region mediate zinc-induced transcriptional activation (33).
In an experiment that examined the effect of pumpkin seed flour biscuits on serum zinc concentration and body weight in malnourished Wistar rats, the researchers concluded that preparing biscuits from pumpkin seed flour increased weight gain in malnourished Wistar rats but did not affect zinc serum levels (34). Compared to the control group and saline training, pumpkin seed oil significantly influenced the expression of the ZnT5 transfer gene. Pumpkin supplement affects ZnT5 gene expression in liver tissue more than pea supplement. Human diets often contain phytate, a zinc chelator that forms insoluble complexes with zinc. Furthermore, zinc could not be absorbed into the digestive tract due to the absence of the phytate hydrolyzing enzyme. As a result, zinc absorption is affected by other minerals in food, such as iron, calcium, and copper. Zinc is bound to non-digestible plant ligands, such as phytate, some dietary fibers, lignin, and products of Maillard reactions, which inhibit its absorption and affect the risk of (primary) dietary zinc deficiency. The pumpkin control group had a greater increase in zinc expression in the liver following zinc supplementation in each tissue, but it was not significant. Zinc concentrations in some cells are very high compared to other cell types. Other factors, such as the prevention of hypozinsemia or hyperzinsemia, a six-month training protocol, or a low supplement intake, may have contributed to the non-significant results in this study.
A significant difference in ZnT9 gene expression was found among the groups. The inter-group comparison showed a significant increase in ZnT9 levels in the training group + pumpkin oil compared to the control, training, and pea groups. No study has examined the effect of these supplements on ZnT9, and only a few studies have examined its effect on zinc and other ZnT family transporters. To date, no studies have conclusively demonstrated the cellular mechanisms of zinc transport under exercise-induced stress. It has not yet been proven that the zinc transporter ZnT9 plays a role in zinc homeostasis (35, 36) or functions as a zinc transporter. Evidence shows that low levels of systemic zinc contribute to several disorders, such as immune system dysfunction, diabetes, hepatitis, and related cancers (37). Several studies have analyzed the expression of zinc transporters in the context of liver and immune cell diseases, and they are also associated with cancer (38). There is scattered and scarce information about ZnT9 and related liver pathologies. Obesity has a secondary effect on the liver, resulting in fatty liver disease, in a way that the ZnT9 transcript level in leukocytes of obese Korean women is decreased in association with ZnT4, ZnT5, ZIP1, ZIP4, and ZIP6 mRNA levels. No data on ZnT9 expression at the RNA or protein level in the liver has emerged (37). However, the available data indicates that ZnT9 (SLC30A9) exports Zn under normal conditions. The mitochondrial proton gradient does not operate in the same direction as other membrane organelles, such as lysosomes and the Golgi apparatus, even though this appears to be in contrast with the known function of other members of the SLC30A family (13), e.g., zinc transport to organelles. It has also been proposed that components of mitochondrial ATP synthase's peripheral stalk contribute to proton channel formation, suggesting SLC30A9 also plays a role in proton transport. The function of ZnT9, however, may not be limited to zinc transport but may also involve micronutrient transport and other cellular functions. A possible reason for the increase in ZnT9 may be that the need for ATP production is increased due to the transfer of H to mitochondria, which releases zinc (33). However, more research is required in this area.
Finally, zinc transporters act as zinc sensors and maintain intracellular zinc homeostasis by responding to zinc availability. Extracellular and intracellular zinc concentrations appear to regulate the expression and distribution of ZIPS and ZnTs (34). Therefore, factors such as exercise and zinc supplements largely determine zinc homeostasis and zinc transporters in the human body. Zinc bioavailability is strongly influenced by diet and zinc absorption (39). As a result, it has been difficult to provide an adequate explanation for the expression of zinc transporters under exercise stress due to a lack of studies. Nevertheless, further research is needed on the effects of training protocol and duration on exercise performance in humans and cellular zinc transporters.
The advantage of this study is the simultaneous assessment of zinc and its transporters, which allowed us to make more confident statements regarding the zinc fluctuations due to the use of supplements and aerobic exercise. Among the limitations of the present study, we can point out the lack of measurement of zinc urine levels, which could have increased the generalizability of the study results. This indicated that future research should also measure urine zinc levels and the zinc content of other tissues, such as muscle.
5.1. Conclusions
The results of the present study suggest that adaptation to moderate-intensity aerobic exercise might prevent perturbation of mineral balance in body fluids and tissues. On the other hand, consumption of zinc-rich food may increase serum zinc levels through zinc transporters. This study showed that ZnT9, the most probable zinc transporter, would alter the transcriptional and post-transcriptional expression in response to zinc fluctuations. Pumpkin supplementation, however, showed the greatest effect in enhancing ZnT9 gene expression and avoiding zinc elimination from the body. Zinc-rich supplements are, therefore, effective in expressing zinc transporters. Since mutations and disorders in zinc transporters cause health problems in the body, this can lead to developing new health-promoting interventions and sports training in line with the changes in the expression of zinc transporters. This indicates the balance of cytoplasmic zinc in normal conditions, which probably supports the theory that only a moderate increase in zinc transporters by the liver may contribute to moderate absorption of zinc, and the increase or decrease of zinc maintains its homeostasis.
Acknowledgements
References
-
1.
Husain H, Ahmad R. Role of zinc in liver pathology. In: Khan ST, Malik A, editors. Microbial Biofertilizers and Micronutrient Availability: The Role of Zinc in Agriculture and Human Health. Cham: Springer International Publishing; 2022. p. 101-13. https://doi.org/10.1007/978-3-030-76609-2_5.
-
2.
Baltaci AK, Gokbel H, Mogulkoc R, Okudan N, Ucok K, Halifeoglu I. The effects of exercise and zinc deficiency on some elements in rats. Biol Trace Elem Res. 2010;134(1):79-83. [PubMed ID: 19618134]. https://doi.org/10.1007/s12011-009-8452-4.
-
3.
Chu A, Foster M, Samman S. Determinants of zinc transport in humans: Zinc status, exercise, inflammation and chronic diseases. In: Sergej MO, editor. Human Health and Nutrition: New Research. New York: Nova Science Publishers; 2015. p. 17-48.
-
4.
Speich M, Pineau A, Ballereau F. Minerals, trace elements and related biological variables in athletes and during physical activity. Clin Chim Acta. 2001;312(1-2):1-11. [PubMed ID: 11580904]. https://doi.org/10.1016/s0009-8981(01)00598-8.
-
5.
Chu A, Petocz P, Samman S. Zinc status at baseline is not related to acute changes in serum zinc concentration following bouts of running or cycling. J Trace Elem Med Biol. 2018;50:105-10. [PubMed ID: 30262266]. https://doi.org/10.1016/j.jtemb.2018.06.004.
-
6.
Grungreiff K, Reinhold D, Wedemeyer H. The role of zinc in liver cirrhosis. Ann Hepatol. 2016;15(1):7-16. [PubMed ID: 26626635]. https://doi.org/10.5604/16652681.1184191.
-
7.
Liu J, Xu C, Yu X, Zuo Q. Expression profiles of SLC39A/ZIP7, ZIP8 and ZIP14 in response to exercise-induced skeletal muscle damage. J Trace Elem Med Biol. 2021;67:126784. [PubMed ID: 34015658]. https://doi.org/10.1016/j.jtemb.2021.126784.
-
8.
Fukada T, Kambe T. Zinc signaling in physiology and pathogenesis. Int J Mol Sci. 2018.
-
9.
Liu X, Qu H, Zheng Y, Liao Q, Zhang L, Liao X, et al. Mitochondrial glycerol 3-phosphate dehydrogenase promotes skeletal muscle regeneration. EMBO Mol Med. 2018;10(12). [PubMed ID: 30389681]. [PubMed Central ID: PMC6284384]. https://doi.org/10.15252/emmm.201809390.
-
10.
Alluri K, Nair KPM, Ghosh S. Differential expression of zinc transporters in functionally contrasting tissues involved in zinc homeostasis. Nucleosides Nucleotides Nucleic Acids. 2020;39(4):615-29. [PubMed ID: 31852371]. https://doi.org/10.1080/15257770.2019.1670838.
-
11.
Fujishiro H, Yoshida M, Nakano Y, Himeno S. Interleukin-6 enhances manganese accumulation in SH-SY5Y cells: implications of the up-regulation of ZIP14 and the down-regulation of ZnT10. Metallomics. 2014;6(4):944-9. [PubMed ID: 24576911]. https://doi.org/10.1039/c3mt00362k.
-
12.
Lichten LA, Cousins RJ. Mammalian zinc transporters: nutritional and physiologic regulation. Annu Rev Nutr. 2009;29:153-76. [PubMed ID: 19400752]. https://doi.org/10.1146/annurev-nutr-033009-083312.
-
13.
Alghadir AH, Gabr SA, Al-Eisa ES, Alghadir MH. Correlation between bone mineral density and serum trace elements in response to supervised aerobic training in older adults. Clin Interv Aging. 2016;11:265-73. [PubMed ID: 27013870]. [PubMed Central ID: PMC4778779]. https://doi.org/10.2147/CIA.S100566.
-
14.
Ganapathy S, Volpe SL. Zinc, exercise, and thyroid hormone function. Crit Rev Food Sci Nutr. 1999;39(4):369-90. [PubMed ID: 10442272]. https://doi.org/10.1080/10408699991279213.
-
15.
Aisa HA, Gao Y, Yili A, Ma Q, Cheng Z. Beneficial role of chickpea (Cicer arietinum l.) functional factors in the intervention of metabolic syndrome and diabetes mellitus. Bioactive Food as Dietary Interventions for Diabetes. Elsevier; 2019. p. 615-27. https://doi.org/10.1016/b978-0-12-813822-9.00039-4.
-
16.
Jukanti AK, Gaur PM, Gowda CL, Chibbar RN. Nutritional quality and health benefits of chickpea (Cicer arietinum L.): a review. Br J Nutr. 2012;108 Suppl 1:S11-26. [PubMed ID: 22916806]. https://doi.org/10.1017/S0007114512000797.
-
17.
El-Ghany A, Hafez AS, EL-SAFTY MS, editors. Biological study on the effect of pumpkin seeds and zinc on reproductive potential of male rats. 2010. 2010.
-
18.
National Research Council. Guide for the care and use of laboratory animals: Eighth edition. Washington, DC: The National Academies Press; 2011.
-
19.
Howarth FC, Almugaddum FA, Qureshi MA, Ljubisavljevic M. Effects of varying intensity exercise on shortening and intracellular calcium in ventricular myocytes from streptozotocin (STZ)-induced diabetic rats. Mol Cell Biochem. 2008;317(1-2):161-7. [PubMed ID: 18553174]. https://doi.org/10.1007/s11010-008-9844-z.
-
20.
Ghanbari Niaki A. Effect of an interval progressive (pyramidal) aerobic training on lipid and lipoprotein profiles in San Shou Athletes. J Humanities. 2005;12(1):97-110.
-
21.
Hoydal MA, Wisloff U, Kemi OJ, Ellingsen O. Running speed and maximal oxygen uptake in rats and mice: practical implications for exercise training. Eur J Cardiovasc Prev Rehabil. 2007;14(6):753-60. [PubMed ID: 18043295]. https://doi.org/10.1097/HJR.0b013e3281eacef1.
-
22.
Attar T. A mini-review on importance and role of trace elements in the human organism. Chem Rev Lett. 2020;3(3):117-30. https://doi.org/10.22034/crl.2020.229025.1058.
-
23.
Radic I, Nestorovic V, Mijovic M, Tatalovic N, Joksimovic B, Lukic V, et al. The effects of whey and pumpkin seed oil on blood biochemical parameters of liver function and lipid profile in rats chronically drinking low concentrations of ethanol. Arch Biol Sci. 2018;70(3):531-41. https://doi.org/10.2298/abs180320014r.
-
24.
Fathi R, Nasiri K, Akbari A, Ahmadi-KaniGolzar F, Farajtabar Z. Exercise protects against ethanol-induced damage in rat heart and liver through the inhibition of apoptosis and activation of Nrf2/Keap-1/HO-1 pathway. Life Sci. 2020;256:117958. [PubMed ID: 32553929]. https://doi.org/10.1016/j.lfs.2020.117958.
-
25.
Livak KJ, Schmittgen TD. Analysis of relative gene expression data using real-time quantitative PCR and the 2(-Delta Delta C(T)) Method. Methods. 2001;25(4):402-8. [PubMed ID: 11846609]. https://doi.org/10.1006/meth.2001.1262.
-
26.
Hall AG, King JC, McDonald CM. Comparison of serum, plasma, and liver zinc measurements by AAS, ICP-OES, and ICP-MS in diverse laboratory settings. Biol Trace Elem Res. 2022;200(6):2606-13. [PubMed ID: 34453311]. [PubMed Central ID: PMC9132797]. https://doi.org/10.1007/s12011-021-02883-z.
-
27.
Volpe SL, Lowe NM, Woodhouse LR, King JC. Effect of maximal exercise on the short-term kinetics of zinc metabolism in sedentary men. Br J Sports Med. 2007;41(3):156-61. [PubMed ID: 17138634]. [PubMed Central ID: PMC2465216]. https://doi.org/10.1136/bjsm.2006.030346.
-
28.
Eide DJ. Zinc transporters and the cellular trafficking of zinc. Biochim Biophys Acta. 2006;1763(7):711-22. [PubMed ID: 16675045]. https://doi.org/10.1016/j.bbamcr.2006.03.005.
-
29.
Dressendorfer RH, Sockolov R. Hypozincemia in Runners. Phys Sportsmed. 1980;8(4):97-100. [PubMed ID: 27432710]. https://doi.org/10.1080/00913847.1980.11710918.
-
30.
Chu A, Petocz P, Samman S. Plasma/Serum Zinc Status During Aerobic Exercise Recovery: A Systematic Review and Meta-Analysis. Sports Med. 2017;47(1):127-34. [PubMed ID: 27260681]. https://doi.org/10.1007/s40279-016-0567-0.
-
31.
Liuzzi JP, Lichten LA, Rivera S, Blanchard RK, Aydemir TB, Knutson MD, et al. Interleukin-6 regulates the zinc transporter Zip14 in liver and contributes to the hypozincemia of the acute-phase response. Proc Natl Acad Sci U S A. 2005;102(19):6843-8. [PubMed ID: 15863613]. [PubMed Central ID: PMC1100791]. https://doi.org/10.1073/pnas.0502257102.
-
32.
Tian X, Zheng Y, Li Y, Shen Z, Tao L, Dou X, et al. Psychological stress induced zinc accumulation and up-regulation of ZIP14 and metallothionein in rat liver. BMC Gastroenterol. 2014;14:32. [PubMed ID: 24548602]. [PubMed Central ID: PMC3931483]. https://doi.org/10.1186/1471-230X-14-32.
-
33.
Myers SA, Nield A, Myers M. Zinc transporters, mechanisms of action and therapeutic utility: implications for type 2 diabetes mellitus. J Nutr Metab. 2012;2012:173712. [PubMed ID: 23304467]. [PubMed Central ID: PMC3530793]. https://doi.org/10.1155/2012/173712.
-
34.
Syam A, Burhan FK, Hadju V, Citrakesumasari C, Akhmar AM. The effect of biscuits made from pumpkin seeds flour on serum zinc levels and weight in malnutrition wistar rats. Open Access Maced J Med Sci. 2020;8(A):428-33. https://doi.org/10.3889/oamjms.2020.4402.
-
35.
Perez Y, Shorer Z, Liani-Leibson K, Chabosseau P, Kadir R, Volodarsky M, et al. SLC30A9 mutation affecting intracellular zinc homeostasis causes a novel cerebro-renal syndrome. Brain. 2017;140(4):928-39. [PubMed ID: 28334855]. [PubMed Central ID: PMC5837213]. https://doi.org/10.1093/brain/awx013.
-
36.
Hogstrand C, Maret W. Genetics of human zinc deficiencies. . Encyclopedia of Life Sciences; 2016. p. 1-8. https://doi.org/10.1002/9780470015902.a0026346.
-
37.
Gartmann L, Wex T, Grungreiff K, Reinhold D, Kalinski T, Malfertheiner P, et al. Expression of zinc transporters ZIP4, ZIP14 and ZnT9 in hepatic carcinogenesis-An immunohistochemical study. J Trace Elem Med Biol. 2018;49:35-42. [PubMed ID: 29895370]. https://doi.org/10.1016/j.jtemb.2018.04.034.
-
38.
Kang X, Chen R, Zhang J, Li G, Dai PG, Chen C, et al. Expression profile analysis of zinc transporters (ZIP4, ZIP9, ZIP11, ZnT9) in gliomas and their correlation with IDH1 mutation status. Asian Pac J Cancer Prev. 2015;16(8):3355-60. [PubMed ID: 25921144]. https://doi.org/10.7314/apjcp.2015.16.8.3355.
-
39.
Lu D, Peng M, Yu M, Jiang B, Wu H, Chen J. Effect of enzymatic hydrolysis on the zinc binding capacity and in vitro gastrointestinal stability of peptides derived from pumpkin (Cucurbita pepo L.) seeds. Front Nutr. 2021;8:647782. [PubMed ID: 33869265]. [PubMed Central ID: PMC8044297]. https://doi.org/10.3389/fnut.2021.647782.