Abstract
Background:
In our previous study, we demonstrated that oak hydroalcoholic extract improves memory impairment in a postpartum depression model due to its antioxidant properties. The purpose of this study is to further investigate the effect of oak on another amnesia model and explore the mediating role of cholinergic muscarinic receptors.Objectives:
This study investigated the effect of hydroalcoholic extract of oak fruit on amnesia induced by morphine in the presence and absence of cholinergic muscarinic receptors, as well as changes in serum biochemical factors.Methods:
In this experimental study, adult male mice were divided into groups: Control and morphine-receiving (5 and 7.5 mg/kg) groups to induce amnesia after training. In the groups receiving 7.5 mg/kg morphine, oak extract (1 and 10 mg/kg) was administered one day after training and continued for one week. Atropine (0.1 and 1 mg/kg) was injected only on the first day after training and before the oak extract injection. Passive avoidance memory was evaluated 1, 3, and 7 days after training using a step-down device. Motor activity was assessed using the open field test. Blood biochemical factors, including triglycerides, cholesterol, high-density lipoprotein (HDL), low-density lipoprotein (LDL), and total antioxidant capacity (TAC), were measured after one week of injections.Results:
Morphine (7.5 mg/kg) impaired memory on days 1 and 7 (P < 0.05), while oak extract (1 and 10 mg/kg) improved memory (P < 0.001). Additionally, the oak extract maintained its enhancing effect even in the presence of atropine, a cholinergic muscarinic system antagonist. Oak extract significantly increased HDL and TAC levels but did not affect LDL, cholesterol, and triglyceride levels.Conclusions:
These findings suggest that oak extract can improve morphine-induced amnesia due to its antioxidant effect, without involving the cholinergic system. Additionally, oak extract can increase beneficial lipids without affecting harmful lipids.Keywords
1. Background
Learning and memory are key brain functions, with the brain’s primary task being to process and store different types of information (1). Memory disorders can affect cognitive abilities and, consequently, quality of life at all stages of life. Despite the increasing prevalence of memory disorders, researchers are continually seeking ways to prevent these disorders and develop strategies to strengthen memory (2). In this context, due to the side effects associated with chemical drugs, the effects of many medicinal plants on learning and memory have been investigated because of their effective compounds and fewer side effects (2, 3).
Quercus brantii (Oak) is a member of the Rashian family of trees. This oak is a native plant of Iran, found from the northwest to the southeast of the Zagros Mountain range. It has various medicinal effects in both traditional and modern treatments (4). Quercus brantii fruit contains several antioxidant compounds, including gallic acid, ellagic acid, malic acid, galloyl derivatives, hexahydroxydiphenyl, tannic acid, quercetin, mucilage, and pectin (5). There are very few studies related to the effect of oak plants on cognitive functions. Although there is limited evidence about the effect of oak on memory, the mechanism of its action remains unknown. However, due to its antioxidant properties, this plant could be a promising candidate for the treatment of cognitive dysfunctions (6, 7). In our previous study, we showed that oak hydroalcoholic extract improves memory impairment in a postpartum depression model due to its antioxidant properties (6). More research is needed to clarify the cognitive and memory-enhancing effects of oak.
On the other hand, the cholinergic system plays an essential role in cognitive functions such as attention, thinking, learning, and memory (8). Cholinergic neurons are widely distributed in brain areas involved in cognitive functions, and cholinergic signaling, which depends on acetylcholine, is related to memory and learning (8). Muscarinic acetylcholine receptors (mAChRs), which are expressed in the central nervous system, mediate functions such as cognition, memory, and reward (9). It has been shown that mAChR agonists and acetylcholinesterase inhibitors improve learning and memory, whereas cholinergic receptor antagonists impair these functions (10). It has also been reported that the use of anticholinergic drugs is associated with cognitive impairment and an increased risk of dementia (11).
Some studies have shown that opioid compounds and their receptors, which are widely distributed in the central nervous system and peripheral tissues, are involved in multiple functions, including reward, pain, emotions, and cognition (12, 13). Opioid receptors affect the regulation of neurotransmitters involved in the pathogenesis of Alzheimer's disease, including cognitive impairment and neuroinflammation (14).
Moreover, the cholinergic system plays a role in several functions of morphine, including memory modulation and analgesia (15). Morphine disrupts the release of acetylcholine and is known as one of the modulators of cholinergic signaling for the treatment of Alzheimer's disease (15).
2. Objectives
According to the information above, this study aims to investigate the mechanism of the biochemical effects of oak hydroalcoholic extract on amnesia induced by morphine in the presence and absence of cholinergic muscarinic receptors.
3. Methods
3.1. Animals and Treatments
In this experimental research, a total of 66 adult male NMRI mice (25 - 30 g) were obtained from the laboratory animal reproduction and maintenance center of Jundishapur University of Medical Sciences, Ahvaz. The mice were 8 weeks old and were kept for one week before the experiments in the animal house of the Biology Department of Shahid Chamran University of Ahvaz under controlled conditions, including a temperature of 25 ± 1°C and a suitable lighting cycle (12 hours light/12 hours dark). Experiments were conducted at specific hours of the day (8:00 - 14:00).
The mice were randomly assigned into eleven groups, with each group consisting of 6 animals. The groups included: A control group (receiving 0.9% saline), groups receiving different doses of morphine (5 and 7.5 mg/kg subcutaneously) to induce amnesia (16), groups receiving the hydroalcoholic extract of oak fruit (1 and 10 mg/kg intraperitoneally) with morphine 7.5 mg/kg, groups receiving atropine (0.1 and 1 mg/kg intraperitoneally) with morphine 7.5 mg/kg (17, 18), groups receiving the hydroalcoholic extract of oak fruit (1 and 10 mg/kg) with atropine (0.1 mg/kg) and morphine (7.5 mg/kg), and groups receiving the hydroalcoholic extract of oak fruit (1 and 10 mg/kg) with atropine (1 mg/kg) and morphine (7.5 mg/kg).
Morphine was injected on the day of training for memory impairment immediately after training. To observe the effect of oak extract or atropine on memory, they were injected 30 minutes before the test, with an interval of 15 minutes between the two injections. All compounds were injected in a volume of 5 mL/kg. Figure 1 shows the study protocol.
The study protocol. A, preparation of hydroalcoholic extract of Quercus brantii fruit; B, step-down training and subcutaneous injection of morphine; C, components injection, step-down and open field tests. Animals were tested 1, 3 and 7 days after the training. The components were injected 30 minutes before the test and for one week and interval time between two injections was 15 minutes; D, serum biochemical parameters were measured in the saline and oak treated groups after the last test.
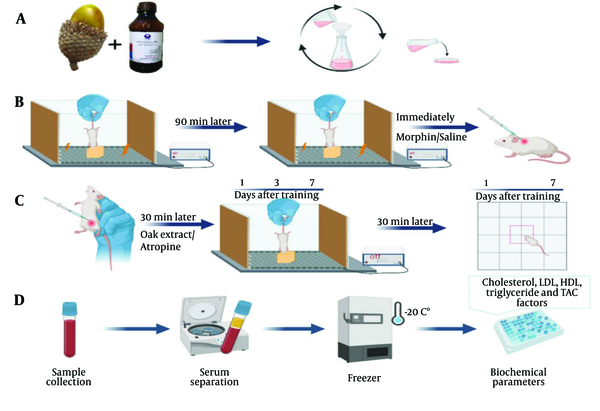
3.2. Quercus brantii Hydroalcoholic Extract Preparation
The required oak fruit was collected from the Shurab and Qala Gol areas around Khorramabad, where the only oak species present is Oak The extraction was performed using the maceration method. Quercus brantii powder (100 g) was mixed with 70% ethanol (1000 mL) and kept for three days at room temperature, with frequent stirring. The mixture was then filtered using Whatman filter paper, and the obtained solution was poured into the bottom of plastic Petri dishes and left at room temperature for two days. After the alcohol evaporated, a thin solid layer remained at the bottom of the Petri dishes, which was stored in the refrigerator at 4°C for further experiments (Figure 1A).
3.3. Behavioral Tests
3.3.1. Step-Down Test
The step-down device was used to investigate passive avoidance memory. This device consists of a plexiglass box (40 × 30 × 30 cm) with a floor covered by steel bars and a wooden platform in the center. An electrical stimulator delivers electric shocks (1 Hz, 50 volts, 15 seconds), transmitting the shock to the animal's paws through the bars on the floor (19). On the training day, the animal is placed on the wooden platform, and when it descends from the platform, an electric shock is delivered to its legs. The animal is placed back on the platform after 90 minutes; if it stays on the platform for 60 seconds, learning is considered to have occurred. If the animal descends from the platform, it will be shocked again (Figure 1B). Tests were conducted on the animals 1, 3, and 7 days after training, with components injected 30 minutes before each test. The delay in stepping down from the platform over a 5-minute period was recorded as the step-down latency (Figure 1C).
3.3.2. Open Field Test
The open field device was a Plexiglas box (30 × 30 × 30 cm) placed on a screen divided into sixteen sections by lines. Each animal was placed in the box for 5 minutes, and the number of times the animal's front and hind limbs crossed the lines was recorded as the motor activity index (Figure 1C) (18).
3.4. Biochemical Analysis
Mice were anesthetized with chloroform, and blood was collected from the heart after the last behavioral experiment. The whole blood was centrifuged at 3000 rpm for 15 minutes, and the serum was stored in a -20°C freezer for biochemical analysis (Figure 1D).
Triglycerides assay: A total of 1000 µL of the solution was mixed with 10 µL of the sample or calibrator, and the complex absorbance was read at 505 nm using a photometer instrument. The results were expressed as mmol/L of serum (Man Company kit).
Cholesterol assay: A total of 1000 µL of the solution was mixed with 10 µL of the sample or calibrator, and the complex absorbance was read at 505 nm using a photometer instrument. The results were expressed as mmol/L of serum (Man Company kit).
low-density lipoprotein (LDL) assay: A total of 900 µL of solution number 1 was mixed with 10 µL of the sample or calibrator, then 300 µL of solution number 2 was added to the previous mixture. The complex absorbance was read at 578 nm using a photometer instrument, and the results were expressed as mmol/L of serum (Man Company kit).
high-density lipoprotein (HDL) assay: A total of 1000 µL of solution number 1 was mixed with 10 µL of the sample or calibrator, then 250 µL of solution number 2 was added to the previous mixture. The complex absorbance was read at 600 nm using a photometer instrument, and the results were expressed as mmol/L of serum (Pars Azmoon kit, Karaj, Iran).
Total antioxidant capacity (TAC) assay: TAC was evaluated in the serum using ferric reducing antioxidant power (FRAP). In this method, 10 µL of the serum was mixed with 250 µL of the FRAP reagent, which contained 2.5 mL of 2,4,6-tris(2pyridyl)-s-triazine (TPTZ) 10 mM in 40 mM HCl and 2.5 mL of 20 mM FeCl3 in 25 mL of 0.1 M acetate buffer (pH = 3.6). The mixture was brought up to 1 mL with distilled water. After ten minutes of incubation at 37°C, the mixture was centrifuged at 10,000 × g. The absorbance of the colored TPTZ complex was read at 593 nm, and the results were expressed as µMol/L of serum.
3.5. Data Analysis
Data were analyzed using Instat3 statistical software, employing one-way analysis of variance (ANOVA) and Tukey's post hoc test to compare groups. In all statistical analyses, a P-value of < 0.05 was considered to indicate a significant difference. All data are presented as mean ± standard error of the mean (SEM).
4. Results
4.1. Behavioral Tests
4.1.1. Step-Down Test
Figure 2 shows that the injection of morphine at 7.5 mg/kg significantly decreased step-down latency on both the 1st and 7th days after training (P < 0.05).
The effects of morphine on memory. * Is P < 0.05 in comparison to the control group.
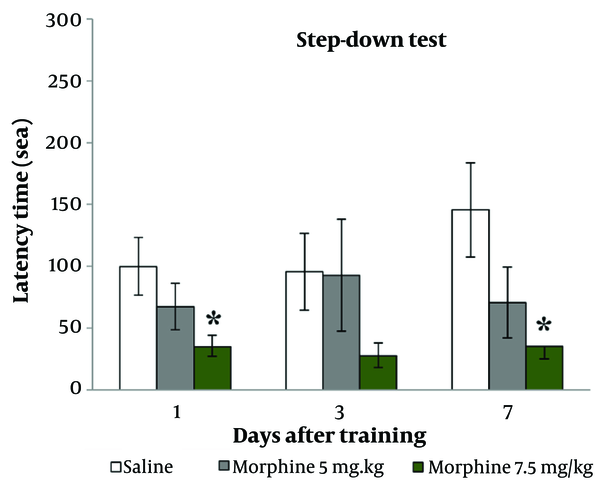
Figure 3 shows that the injection of oak at 1 mg/kg increased step-down latency compared to the saline group, with the increase being significant only on the first day after training (P < 0.05). The injection of oak at 10 mg/kg significantly increased step-down latency on the 1st, 3rd, and 7th days after training (P < 0.001). Additionally, a significant difference was observed between the oak 1 mg/kg and 10 mg/kg groups (P < 0.05 and P < 0.001, respectively).
The effects of Quercus brantii on memory impairment induced by morphine. + is P < 0.05 and +++ is P < 0.001 in comparison to the saline group, # is P < 0.05 and ### is P < 0.001 in comparison to the oak 1 mg/kg group.
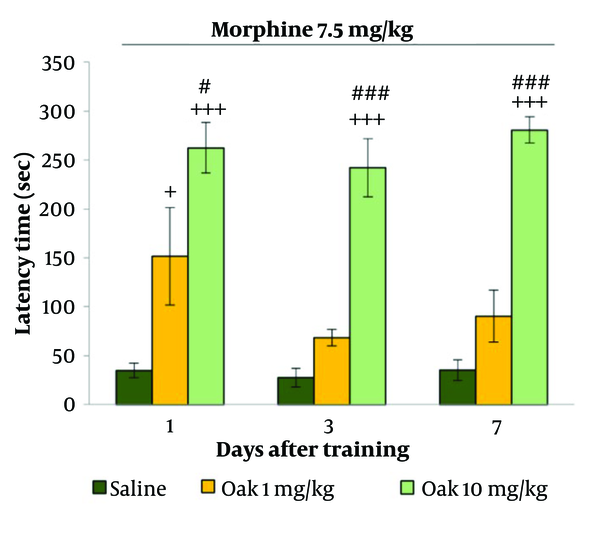
Figure 4 shows the effect of the hydroalcoholic extract of oak fruit (1 and 10 mg/kg) on morphine-induced amnesia in the presence of atropine (0.1 and 1 mg/kg) during the first and third days after training. Atropine (0.1 and 1 mg/kg) did not affect step-down latency (P > 0.05) (Figure 4A, B).
Oak at 1 mg/kg and 10 mg/kg improved memory in the presence of atropine (0.1 and 1 mg/kg), with the improvement being significant in the oak 10 mg/kg treated group (P < 0.01 & P < 0.001) (Figure 4A, B).
The effect of oak injection on memory impairment induced by morphine in the presence of atropine. $$ is P < 0.01 and $$$ is P < 0.001 in comparison to oak 10 mg/kg group, ++ is P < 0.01 in comparison to oak 1 mg/kg group, # is P < 0.05 in comparison to oak 10 mg/kg group + Atropine 0.1 mg/kg.
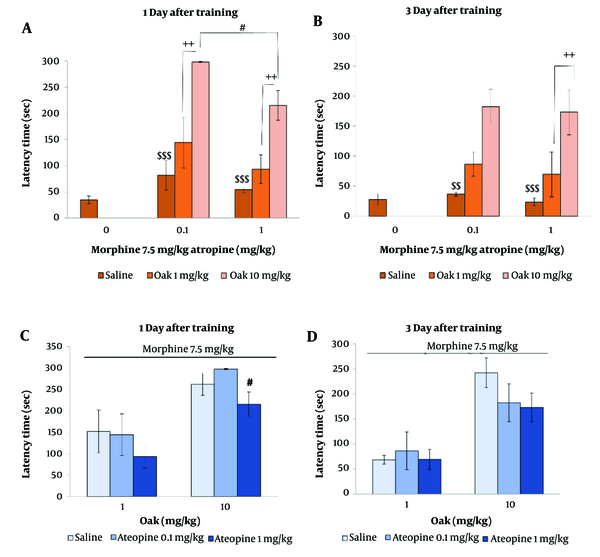
However, the results show that atropine at 1 mg/kg significantly decreased the effect of oak at 10 mg/kg on memory compared to atropine at 0.1 mg/kg (P < 0.05) (Figure 4C, D).
4.1.2. Open Field Test
Table 1 presents the effects of oak fruit extract, morphine, and atropine on the motor activity of mice. No significant differences were observed in the motor activities across all groups.
Motor Activity Index in All Groups
Groups | Days After Training (Number of Crossing) | |
---|---|---|
1 | 7 | |
Control | 67.75 ± 9.215 | 72.375 ± 11.268 |
Morphine 5 mg/kg | 59.125 ± 10.711 | 57.65 ± 10.852 |
Morphine 7.5 mg/kg | 53.8 ± 3.876 | 63.6 ± 10.923 |
Morphine 7.5 mg/kg + Oak 1 mg/kg | 32 ± 9.641 | 38.28 ± 7.668 |
Morphine 7.5 mg/kg + Oak 10 mg/kg | 44.2 ± 4.895 | 43.2 ± 4.743 |
Morphine 7.5 mg/kg + Atropine 0.1 mg/kg | 49.16 ± 7.855 | Did not measure |
Morphine 7.5 mg/kg + Atropine 1 mg/kg | 32.5 ± 5.282 | Did not measure |
Morphine 7.5 mg/kg + Atropine 0. 1 mg/kg + Oak 1 mg/kg | 29 ± 6.288 | Did not measure |
Morphine 7.5 mg/kg + Atropine 0. 1 mg/kg + Oak 10 mg/kg | 42.83 ± 6.395 | Did not measure |
Morphine 7.5 mg/kg + Atropine 0. 1 mg/kg + Oak 1 mg/kg | 39.6 ± 7.319 | Did not measure |
Morphine 7.5 mg/kg + Atropine 0. 1 mg/kg + Oak 10 mg/kg | 29.4 ± 4.477 | Did not measure |
4.2. Biochemical Analysis
Table 2 shows the effect of hydroalcoholic extract of oak fruit (1 and 10 mg/kg) on serum biochemical parameters. Injection of oak at 10 mg/kg for one week significantly increased HDL and TAC levels (P < 0.05) but did not affect LDL, cholesterol, or triglyceride levels.
The Serum Biochemical Parameters
Groups | LDL (mg/dL) | HDL (mg/dL) | Triglyceride (mg/dL) | Cholesterol (mg/dL) | TAC (mmol Vit C Eq/L) |
---|---|---|---|---|---|
Morphine 7.5 mg/kg + saline | 76.166 ± 4.468 | 32.833 ± 4.037 | 92.833 ± 12.219 | 126.166 ± 7.125 | 1.0475 ± 0.063 |
Morphine 7.5 mg/kg + Oak 1 mg/kg | 74.8 ± 6.896 | 31.4 ± 3.21 | 125.8 ± 9.516 | 131.8 ± 9.847 | 1.1112 ± 0.117 |
Morphine 7.5 mg/kg + Oak 10 mg/kg | 57 ± 4.099 | 48.25 ± 2.761 a, b | 115.25 ± 8.372 | 128.75 ± 5.946 | 1.43475 ± 0.1119 a |
5. Discussion
The results of this experiment showed that morphine induced amnesia without affecting motor activity (Figure 2). Previous studies have demonstrated that morphine causes memory impairment (20, 21). Opioids may affect learning and memory by decreasing the release of acetylcholine in the brain via mu receptors (22, 23). Morphine triggers neuroinflammatory responses in the central nervous system (24). It is possible that neuroinflammation induced by morphine may be mediated by toll-like receptor 4 (TLR4), an innate immune receptor in the body, which is associated with the activation of the signaling pathway (NF/KB) involved in the release of neurotransmitters (24, 25). Morphine disrupts the opening of the calcium ion voltage-dependent channel in presynaptic neurons (15), thereby reducing the release of excitatory neurotransmitters, such as glutamate and acetylcholine, which are crucial for memory formation (15).
The results also showed that oak fruit could improve memory impairment in a dose-dependent manner (Figure 3). Quercus brantii fruit contains compounds with antioxidant effects (5, 6). Flavonoids can prevent neurodegeneration associated with neurological disorders and cognitive impairment by protecting neurons against damage caused by neurotoxins (26). Flavonoids may reduce neuroinflammation and memory disorders by modulating TLR4/NF-κB signaling (24).
Additionally, the results showed that oak at 10 mg/kg could improve amnesia caused by morphine even in the presence of atropine (Figure 4A, B). However, atropine at 1 mg/kg, compared to the dose of 0.1 mg/kg, significantly reduced the effect of oak at 10 mg/kg on memory improvement (Figure 4C, D).
Atropine is a competitive antagonist for mAChRs and is classified as an anticholinergic drug that can lead to memory impairment (27, 28). Acetylcholine activates muscarinic and nicotinic acetylcholine receptors, resulting in long-term potentiation of the postsynaptic membrane and subsequent activation of the downstream cellular pathway (ERK), which creates favorable conditions for neural plasticity (29). The breakdown of some cholinergic nuclei is involved in the development of cognitive disorders such as Alzheimer's (30). It is likely that the oak fruit extract strengthens its effect on memory impairment without interfering with the cholinergic system.
On the other hand, biochemical results showed that oak fruit extract has no significant effect on serum LDL, cholesterol, and triglycerides, while it increases HDL and TAC levels (Table 2). The beneficial effect of oak on the blood lipid profile may be related to the composition of its fatty acids (31). Phenolic compounds can reduce plasma total cholesterol levels as well as LDL cholesterol levels (31, 32). Quercus brantii is a source of neutral cholesterol, oleic acid, alpha-linolenic acid, and polyunsaturated fatty acids, which are important in eicosanoid synthesis and have beneficial effects on serum triglyceride and HDL levels (31). Martinez et al. have shown that small HDL particles reduce the risk of Alzheimer's disease due to their capacity to exchange lipids and affect neural membrane composition and synaptic functions (33). Additionally, Sanjana et al. reported that increased serum triglycerides are associated with decreased hippocampal integrity, while HDL-C may help maintain hippocampal integrity (34). Taghvimi et al. suggested that the antidepressant effect of Q. brantii extract and oil is linked to the stimulation of neurotrophic factors and the antioxidant activity of oak extract, indicating its neuroprotective benefits (6).
5.1. Limitations
Due to the side effects of atropine, such as increased heart rate and decreased gastrointestinal motility, we were limited in increasing its dose.
5.2. Conclusions
Based on the findings, it appears that the hydroalcoholic extract of oak fruit improves amnesia caused by morphine, likely due to its antioxidant properties, without the involvement of the cholinergic system. Additionally, since oak fruit can increase beneficial lipids without affecting harmful lipids, it may be a suitable candidate for improving memory disorders with positive effects and minimal side effects, at least on serum biochemical factors.
Acknowledgements
References
-
1.
Bisaz R, Travaglia A, Alberini CM. The neurobiological bases of memory formation: from physiological conditions to psychopathology. Psychopathol. 2014;47(6):347-56. [PubMed ID: 25301080]. [PubMed Central ID: PMC4246028]. https://doi.org/10.1159/000363702.
-
2.
Shahinfar J, Zeraati H, Ghorbanzadeh M, Nasimi F, Shojaei S. [Effect of medical Plants on memory and learning, A review article]. J Islamic Iran Traditional Med. 2017;8(3):347-62. FA.
-
3.
Ramezani M, Meymand AZ, Khodagholi F, Kamsorkh HM, Asadi E, Noori M, et al. A role for flavonoids in the prevention and/or treatment of cognitive dysfunction, learning, and memory deficits: a review of preclinical and clinical studies. Nutr Neurosci. 2023;26(2):156-72. [PubMed ID: 35152858]. https://doi.org/10.1080/1028415X.2022.2028058.
-
4.
Karimian M, Najafi R, Jaimand K, Hatami F, Abbasi N, Jalali Ghalousangh A. Extraction and Identification of phytochemicals in Iranian oak (Quercus brantii var. Persica) Collected in Arghavan Valley, Ilam County by HS-SPME and GC-MS. J Med Plants By-Products. 2020;9(Special):81-6. https://doi.org/10.22092/jmpb.2020.121754.
-
5.
Bahmani M, Forouzan SH, Fazeli-Moghadam E, Rafieian-Kopaei M, Adineh A, Saberianpour SH. Oak (Quercus branti): an overview. J chem pharm res. 2015;7(1):634-9.
-
6.
Taghvimi S, Kesmati M, Shahriari A, Fatemi Tabatabaei SR. Antioxidative Effects of Hydro-Alcoholic Extract and Essential Oil of Quercus brantii Exerts Anti-Depressant-Like Effect and Improvement of Memory Defect in Progesterone-Induced Postpartum Depression in Rats. Zahedan J Res Med Sci. 2024;26(2). https://doi.org/10.5812/zjrms-143020.
-
7.
Taib M, Rezzak Y, Bouyazza L, Lyoussi B. Medicinal Uses, Phytochemistry, and Pharmacological Activities of Quercus Species. Evid Based Complement Alternat Med. 2020;2020:1920683. [PubMed ID: 32802116]. [PubMed Central ID: PMC7415107]. https://doi.org/10.1155/2020/1920683.
-
8.
Bekdash RA. The Cholinergic System, the Adrenergic System and the Neuropathology of Alzheimer's Disease. Int J Mol Sci. 2021;22(3). [PubMed ID: 33525357]. [PubMed Central ID: PMC7865740]. https://doi.org/10.3390/ijms22031273.
-
9.
Szczurowska E, Szanti-Pinter E, Chetverikov N, Randakova A, Kudova E, Jakubik J. Modulation of Muscarinic Signalling in the Central Nervous System by Steroid Hormones and Neurosteroids. Int J Mol Sci. 2022;24(1). [PubMed ID: 36613951]. [PubMed Central ID: PMC9820491]. https://doi.org/10.3390/ijms24010507.
-
10.
Hampel H, Mesulam MM, Cuello AC, Farlow MR, Giacobini E, Grossberg GT, et al. The cholinergic system in the pathophysiology and treatment of Alzheimer's disease. Brain. 2018;141(7):1917-33. [PubMed ID: 29850777]. [PubMed Central ID: PMC6022632]. https://doi.org/10.1093/brain/awy132.
-
11.
Risacher SL, McDonald BC, Tallman EF, West JD, Farlow MR, Unverzagt FW, et al. Association Between Anticholinergic Medication Use and Cognition, Brain Metabolism, and Brain Atrophy in Cognitively Normal Older Adults. JAMA Neurol. 2016;73(6):721-32. [PubMed ID: 27088965]. [PubMed Central ID: PMC5029278]. https://doi.org/10.1001/jamaneurol.2016.0580.
-
12.
Meier IM, Eikemo M, Leknes S. The Role of Mu-Opioids for Reward and Threat Processing in Humans: Bridging the Gap from Preclinical to Clinical Opioid Drug Studies. Curr Addict Rep. 2021;8(2):306-18. [PubMed ID: 34722114]. [PubMed Central ID: PMC8550464]. https://doi.org/10.1007/s40429-021-00366-8.
-
13.
Liu Z, Udenigwe CC. Role of food-derived opioid peptides in the central nervous and gastrointestinal systems. J Food Biochem. 2019;43(1). e12629. [PubMed ID: 31353498]. https://doi.org/10.1111/jfbc.12629.
-
14.
Cai Z, Ratka A. Opioid system and Alzheimer's disease. Neuromolecular Med. 2012;14(2):91-111. [PubMed ID: 22527793]. https://doi.org/10.1007/s12017-012-8180-3.
-
15.
Trigo JM, Martin-Garcia E, Berrendero F, Robledo P, Maldonado R. The endogenous opioid system: a common substrate in drug addiction. Drug Alcohol Depend. 2010;108(3):183-94. [PubMed ID: 19945803]. https://doi.org/10.1016/j.drugalcdep.2009.10.011.
-
16.
Alizadeh-Makondi A, Khajeh-Pour L, Kasmati M. [Effect of hydro-alcoholic extract of Salvia officinalis on memory impaired by post-training administration of morphine using a passive avoidance paradigm in rat]. Feyz Med Sci J. 2011;15(4):302-9. FA.
-
17.
Ghassemi F, Momenzade M, Najafian M, Kargar Jahromy H. The effect of hydroalcoholic extract of Oak fruit husks on liver function in Rat (Wistar)The effect of hydroalcoholic extract of Oak fruit husks on liver in Rat (Wistar). Pars Jahrom Univ Med Sci. 2014;12(3):7-1. https://doi.org/10.29252/jmj.12.3.7.
-
18.
Sargholi Nootarki Z, Kesmati M, Poormehdi Borujeni M. Effect of Magnesium Oxide Nanoparticles on Atropine-Induced Memory Impairment in Adult Male Mice. Avicenna J Neuro Psych Physiol. 2015;2(4). https://doi.org/10.17795/ajnpp-36924.
-
19.
Narwal S, Saini DR, Kumaria K, Narwal S, Sing G, Singh Negi R, et al. Behavior & Pharmacological Animal Models for the Evaluation of Learning & Memory Condition. Indo Global J Pharmaceutical ScI. 2012;2(2):121-9. https://doi.org/10.35652/igjps.2012.15.
-
20.
Zarrindast MR, Khakpai F. State-dependent memory and its modulation by different brain areas and neurotransmitters. EXCLI J. 2020;19:1081-99. [PubMed ID: 33013265]. [PubMed Central ID: PMC7527511]. https://doi.org/10.17179/excli2020-2612.
-
21.
Mohammadkhani M, Gholami D, Riazi G. The effects of chronic morphine administration on spatial memory and microtubule dynamicity in male mice's brain. IBRO Neurosci Rep. 2024;16:300-8. [PubMed ID: 38390235]. [PubMed Central ID: PMC10881431]. https://doi.org/10.1016/j.ibneur.2024.02.002.
-
22.
Van der Jeugd A, Ahmed T, Burnouf S, Belarbi K, Hamdame M, Grosjean ME, et al. Hippocampal tauopathy in tau transgenic mice coincides with impaired hippocampus-dependent learning and memory, and attenuated late-phase long-term depression of synaptic transmission. Neurobiol Learn Mem. 2011;95(3):296-304. [PubMed ID: 21167950]. https://doi.org/10.1016/j.nlm.2010.12.005.
-
23.
Carrera MP, Carey RJ, Dias FR, de Matos LW. Reversal of apomorphine locomotor sensitization by a single post-conditioning trial treatment with a low autoreceptor dose of apomorphine: a memory re-consolidation approach. Pharmacol Biochem Behav. 2011;99(1):29-34. [PubMed ID: 21447355]. https://doi.org/10.1016/j.pbb.2011.03.018.
-
24.
Guan S, Jin T, Han S, Fan W, Chu H, Liang Y. Dihydroartemisinin alleviates morphine-induced neuroinflammation in BV-2 cells. Bioengineered. 2021;12(2):9401-10. [PubMed ID: 34854364]. [PubMed Central ID: PMC8810002]. https://doi.org/10.1080/21655979.2021.1982311.
-
25.
Malik JA, Agrewala JN. Morphine acts via TLR4 resulting in neuroinflammation and immunosuppression. Med Hypotheses. 2024;186. https://doi.org/10.1016/j.mehy.2024.111335.
-
26.
Spencer JP. The impact of fruit flavonoids on memory and cognition. Br J Nutr. 2010;104 Suppl 3:S40-7. [PubMed ID: 20955649]. https://doi.org/10.1017/S0007114510003934.
-
27.
Al B. The Source-Synthesis- History and Use of Atropine. J Acad Emerg Med. 2014;13(1):2-3. https://doi.org/10.5152/jaem.2014.1120141.
-
28.
Rafiq S, Batool Z, Liaquat L, Haider S. Blockade of muscarinic receptors impairs reconsolidation of older fear memory by decreasing cholinergic neurotransmission: A study in rat model of PTSD. Life Sci. 2020;256:118014. [PubMed ID: 32593712]. https://doi.org/10.1016/j.lfs.2020.118014.
-
29.
Giovannini MG, Lana D, Pepeu G. The integrated role of ACh, ERK and mTOR in the mechanisms of hippocampal inhibitory avoidance memory. Neurobiol Learn Mem. 2015;119:18-33. [PubMed ID: 25595880]. https://doi.org/10.1016/j.nlm.2014.12.014.
-
30.
Ferreira-Vieira TH, Guimaraes IM, Silva FR, Ribeiro FM. Alzheimer's disease: Targeting the Cholinergic System. Curr Neuropharmacol. 2016;14(1):101-15. [PubMed ID: 26813123]. [PubMed Central ID: PMC4787279]. https://doi.org/10.2174/1570159x13666150716165726.
-
31.
Salajpal K, Karolyi D, Dikic M, Kantura V, Kis G, Sinjer Z. Influence of acorn intake on blood lipid profile and longisimus muscle characteristics of black slavonian pig. Acta agriculturae Slovenica. 2008;2:99-105.
-
32.
Kan J, Cao M, Chen C, Gao M, Zong S, Zhang J, et al. In vitro antioxidant and lipid-lowering properties of free and bound phenolic compounds from buckwheat hulls. Food Bioscience. 2023;53. https://doi.org/10.1016/j.fbio.2023.102725.
-
33.
Martinez AE, Weissberger G, Kuklenyik Z, He X, Meuret C, Parekh T, et al. The small HDL particle hypothesis of Alzheimer's disease. Alzheimers Dement. 2023;19(2):391-404. [PubMed ID: 35416404]. [PubMed Central ID: PMC10563117]. https://doi.org/10.1002/alz.12649.
-
34.
Sanjana F, Delgorio PL, Hiscox LV, DeConne TM, Hobson JC, Cohen ML, et al. Blood lipid markers are associated with hippocampal viscoelastic properties and memory in humans. J Cereb Blood Flow Metab. 2021;41(6):1417-27. [PubMed ID: 33103936]. [PubMed Central ID: PMC8142125]. https://doi.org/10.1177/0271678X20968032.