Abstract
Context:
Nanotechnology, specifically nanoparticles, has revolutionized cancer therapy through enhanced drug delivery, precise imaging, and accurate diagnosis. Their ability to target cancer cells directly with minimal side effects makes them especially promising for treating colorectal cancer (CRC), allowing for more effective treatments.Methods:
A systematic literature review was conducted across multiple databases (PubMed, Scopus, Embase, Cochrane, Web of Science, Google Scholar) using MeSH terms such as nanoparticles, cancer, drug delivery, and target cells. The objectives of this review were to examine the effectiveness of nanoparticle-based drug delivery systems, evaluate their targeting mechanisms, and assess their impact on treatment outcomes for CRC. Study eligibility, participant inclusion criteria, and specific intervention types were critically analyzed to ensure a comprehensive understanding.Results:
Nanoparticle-based systems have demonstrated significant promise in shrinking tumors, improving drug accumulation at the tumor site, and facilitating easier surgical removal. These systems enhance drug efficacy, reduce toxicity, and overcome biological barriers in CRC, particularly through the use of pH-responsive and thermoresponsive nanoparticles.Conclusions:
The application of nanoparticles for drug delivery represents a safe and effective treatment option, demonstrating improved targeting, reduced side effects, and enhanced therapeutic outcomes. However, further research is necessary to evaluate the long-term safety, efficacy, and scalability of these systems, alongside their optimization for clinical use in personalized treatments.Keywords
1. Context
1.1. An Introduction to Nanoparticles
Nanoparticles are particles measured on a scale of 1 to 100 nanometers (1-3). These particles can consist of tens or hundreds of atoms or molecules with varying sizes and morphologies, including structures such as nanospheres, nanocapsules, liposomes, micelles, fullerenes, nanotubes, and polymers (1-3). Most commercially used nanoparticles are available as dry powder, liquid, or as suspensions or pastes in organic or aqueous solutions (1, 2). Their compatibility with biological environments, such as the human body, ability to traverse biological barriers, and degradability make nanoparticles highly suitable for biological and medical purposes (1, 2). The use of inorganic nanoparticles, such as quantum dots with magnetic core properties like spin, has proven valuable for cancer detection and imaging. Similarly, organic nanoparticle systems, including liposomes, solid lipid nanoparticles, aptamers, dendrimers, and nanobodies, have shown great potential for drug and ligand delivery (1-3). Aptamers are oligonucleotide or peptide molecules that bind to gene products or target cells and are utilized in diagnosis, treatment, molecular imaging, and drug delivery (1, 3). Nanobodies, as antibody molecules, have also become widely used in cancer diagnosis and treatment. In recent years, advancements in nanotechnology have enabled the application of nanoparticles across various fields, including industry, disease diagnosis, drug delivery, and cancer treatment (1, 2). These advancements have further enhanced their role in diagnosis, imaging, and drug delivery systems for cancer therapy (1-3).
1.2. An Introduction to Cancer
Cancer is one of the most common diseases affecting millions of people worldwide (2, 3). In all types of cancer, certain cells in the body begin to grow and divide uncontrollably, spreading to surrounding tissues (1-3). Some cancer cells can travel to distant parts of the body through the blood or lymphatic system, leading to the formation of tumors (1-3).
The tissue resulting from the abnormal growth of cancer cells is called a tumor, which is classified into two types: benign and malignant (4). Benign tumors typically do not spread to nearby tissues or metastasize to distant sites. However, in some cases, they can grow large enough to necessitate major surgical removal (4). Benign tumors generally do not recur after removal (4). Cancer is a genetic disease that may be inherited from parents or caused by environmental factors such as chemicals in tobacco or ultraviolet radiation from the sun (4). When cancer originates in one location and spreads to other parts of the body, it is referred to as metastatic cancer, and the process of its spread is called metastasis (4). Overall, cancer is considered a hereditary disease influenced by both genetic and environmental factors (4).
2. Objectives
This review aims to explore the expanding role of nanoparticles in advancing colorectal cancer (CRC) treatment. It focuses on assessing how nanoparticles enhance drug targeting, increase drug accumulation at tumor sites, and reduce side effects compared to traditional therapies. The review also examines how nanoparticles address challenges such as tumor resistance and limited drug uptake. Furthermore, it highlights the potential of these systems for personalized cancer therapies, emphasizing their clinical feasibility, barriers to widespread adoption, and the future direction of this innovative approach in oncology.
3. Method
A systematic literature search was performed in PubMed, Scopus, Embase, Cochrane, Web of Science, and Google Scholar using all available MeSH terms for nanoparticles, cancer, oncogene, and target cell. Studies were selected based on inclusion criteria focusing on CRC, nanoparticle-based therapies, and gene expression alterations. Data extraction involved reviewing each study for key outcomes, including treatment efficacy, gene expression changes, and tumor response. Studies were assessed for quality based on methodological rigor, with discrepancies resolved through consensus.
4. Results
4.1. Factors Affecting Cancer
Many factors can cause cell abnormalities and transform them into cancerous cells (5). These factors are categorized into three groups: Physical, chemical, and biological. Among the known contributors are environmental and lifestyle factors (5, 6). Examples include exposure to chemical or toxic compounds such as benzene, asbestos, nickel, vinyl chloride, cadmium, and aflatoxin, often found in tobacco smoke or cigarettes (6).
Physical factors include ionizing radiation, ultraviolet rays from the sun, uranium, radon, alpha, beta, and gamma rays, and X-rays. Biological factors encompass pathogens and viruses such as epstein-barr virus (EBV), human papillomavirus (HPV), Hepatitis B and C viruses, Kaposi's sarcoma virus, schistosoma, and Helicobacter pylori. Genetics and even dietary factors also play a significant role, particularly in their interaction with the body's immune system to prevent the growth of cancer cells (5, 6).
Additional contributors to cancer development include obesity, inactivity, chronic inflammation, and hormonal imbalances, all of which significantly impact the progression of the disease (5, 6).
4.2. Colorectal Cancer
Colorectal cancer is the third most common cancer in men and the second most common cancer in women. This type of cancer originates in the large intestine (colon) and primarily affects older individuals, although it can occur at any age (7). Colorectal cancer is a widespread disease, impacting hundreds of thousands annually and resulting in tens of thousands of deaths.
Most CRCs begin as small, benign cell masses called polyps, which form on the inner wall of the colon. Over time, some polyps can transform into malignant CRC (7). Since polyps are often small and asymptomatic, researchers emphasize the importance of regular screening tests to identify and remove polyps before they progress to cancer. This makes CRC one of the few cancers where early detection and prevention can significantly improve outcomes (7, 8).
Screening plays a crucial role in the early diagnosis and prevention of CRC. Techniques such as sigmoidoscopy, colonoscopy, and barium enema have significantly reduced the mortality rate of CRC patients (7, 8).
Recent advances in molecular medicine and genetics have brought a promising perspective to CRC management (7, 8). Genetic testing for hereditary CRC syndromes, such as hereditary non-polyposis colorectal cancer (HNPCC) and familial adenomatous polyposis (FAP), has enabled more precise prevention strategies. Modeling and analyzing CRC prevention programs in Western countries and the United States further demonstrate the potential of these advancements (7, 8).
The benefits of genetic testing include identifying high-risk, medium-risk, and even normal individuals predisposed to CRC. With the establishment of specific guidelines, the genetic background of CRC can be clarified before symptoms appear, facilitating preventive measures. Early diagnosis through genetic testing provides the opportunity for targeted prevention and personalized patient care (7, 8).
4.3. Common Cancer Treatment Methods
4.3.1. Surgery
Colorectal cancer treatment is more effective in the early stages and is often performed using minimally invasive procedures. These include the removal of polyps during colonoscopy (polypectomy), endoscopic mucosal resection (removing large polyps along with a small portion of the intestinal lining using specialized instruments), and laparoscopic surgery for polyps that cannot be removed via colonoscopy (9).
In laparoscopic surgery, the doctor makes several small incisions in the abdominal wall to perform the procedure. Additionally, lymph node sampling is often conducted in the area where the cancer is located, which is a routine part of this procedure (9).
4.3.2. Chemotherapy
For advanced CRC or cases where the cancer has spread to the lymph nodes, chemotherapy is typically administered after surgery (10). Chemotherapy aims to eliminate any remaining cancer cells in the body and reduce the risk of the cancer becoming more malignant (10).
In some cases, chemotherapy is given before surgery to shrink the tumor, making it easier to remove the affected portion of the intestine (10). However, chemotherapy can have side effects, such as hair loss and other systemic complications (10).
4.3.3. Radiation Therapy
Radiation therapy is another treatment option for CRC (11). This method uses powerful energy sources, such as X-rays or gamma rays, to destroy cancer cells (11).
Radiation therapy may be used prior to surgery to shrink the tumor, facilitating easier removal. In some instances, it is combined with chemotherapy to enhance the effectiveness of the treatment (11).
4.3.4. Monoclonal Antibodies
Monoclonal antibodies are a specialized class of glycoproteins produced in response to exposure to foreign bodies or antigens (12). Their primary function is to bind to antigens, thereby preventing them from interacting with receptors on target cells. Additionally, they cover invading microorganisms, enabling other components of the immune system to recognize and destroy them (12).
In human blood serum, approximately 10⁷ to 10⁹ distinct antibody molecules exist. However, determining their exact structure is challenging because serum contains a mixture of antibodies produced by various B lymphocyte clones, referred to as polyclonal antibodies (12).
Many monoclonal antibodies are utilized in cancer treatment, particularly in targeted therapies (12). These drugs are designed to specifically target cancer cells. Cancer cells often express proteins that facilitate unchecked growth and division, and monoclonal antibodies precisely target these proteins (12).
For example, Rituximab is a monoclonal antibody that binds to CD20 proteins found on type B-cells in certain cancers. By binding to these proteins, Rituximab triggers the immune system to destroy the targeted cancer cells (12).
4.4. Targeted Treatment with Nanoparticles
Researchers have identified targeted drug delivery as one of the most effective treatment methods, often guided by the attending physician's recommendation (13). Nanoparticles have emerged as promising tools for targeted drug delivery, offering significant advantages in therapeutic applications (13). They exhibit unique magnetic, thermal, optical, and electrical properties that make them well-suited for advanced medical applications (13).
Nanoparticles serve as carriers for targeted therapies, improving the efficacy of traditional treatments such as chemotherapy and radiotherapy while minimizing their associated side effects. Unlike conventional methods that may cause damage to DNA and surrounding healthy tissues, nanoparticle carriers can deliver drugs precisely to the target site without causing harm (13).
To be effective, nanoparticle drug carriers must meet specific criteria: They should be degradable, stable, non-immunogenic, easy to manufacture, cost-effective, and capable of releasing their payload exclusively at the target site (13). Nanotechnology enables the delivery of anti-neoplastic drugs with high specificity and concentration, offering significant advancements in cancer treatment, particularly CRC (13).
Two unique features make nanoparticles exceptional for drug delivery: (1) Differential membrane electrical properties: Tumor cells and normal tissue cells exhibit different electrical properties in their membranes, allowing nanoparticles to target cancer cells more precisely; (2) electromagnetic nanoparticles: These nanoparticles can convert long-distance magnetic energy into electrical energy. Their inherent shape and magnetic properties enable researchers to remotely manipulate the electric field of membranes, achieving high-specificity drug release (13).
Nanoparticles have paved the way for innovative and effective drug delivery systems, offering a transformative approach to cancer treatment.
Cancer cells are otherwise normal cells that acquire unique mutations in growth regulatory genes, enabling them to divide uncontrollably and gain the ability to metastasize (14). These cells outcompete normal cells for vital resources like oxygen, glucose, and amino acids to sustain their growth and division. However, a tumor cannot grow beyond approximately 2 cubic millimeters without developing its own blood supply (14).
This requirement is fulfilled through the release of angiogenic factors by neoplastic tissues, which promote the formation of new blood vessels. These newly formed vessels enable the tumor to continue its growth (14). Unlike normal blood vessels, which have intact cellular junctions, tumor-associated blood vessels are characterized by numerous pores and voids between their endothelial cells (14).
Conventional cancer treatments include surgery, radiotherapy, chemotherapy, hormone therapy, and immunotherapy (14). However, the inability of many drugs to specifically target cancer cells limits their effectiveness and introduces challenges in further treatment development (14). Improving the specificity of drug delivery is often more efficient and cost-effective than developing entirely new drugs (14).
Drug delivery targeting can be classified into three types: Passive, active, or physical targeting. These strategies enable the targeting of specific organs, cells, or even organelles within cells. Many cancers exhibit organelle-specific pathways that provide opportunities for precision drug delivery (13-15).
For instance, nanoparticles can be functionalized with materials like polyethylene glycol (PEG) to form complexes that bind to cell surfaces (13-15). Once attached, these particles are internalized by the cell through endocytosis, enabling the transfer of the drug into the target cell for precise therapeutic action (13-15).
4.5. Genetic Engineering and Nanoparticles
Nanovectors, such as modified viruses, are being explored for their potential to positively influence the body's immune system. Research focuses on developing nanoparticles capable of carrying genes and being compatible with the biological systems of the body (16). Recent advancements in gene therapy involve transferring drugs, gene products, or the target gene itself using nanoparticles in conjunction with viral lipids or protein complexes (15). Although viral complexes were initially employed, their ability to stimulate immune responses rendered them less favorable for therapeutic applications (16). Currently, non-viral complexes are preferred for gene delivery, with nanoparticles emerging as the most advanced method (16). One notable example involves the use of gold nanoparticles, which are particularly effective due to their ability to attach easily to animal or plant cells. Their antimicrobial properties minimize immune system activation and inflammatory responses, making them an ideal choice for gene-related therapies (16, 17). Gold nanoparticles can bind to target gene products and act as biomarkers, aiding in the identification and study of genetic materials (17). Recent innovations have produced nanoparticles with higher efficiency than previous methods, allowing for the labeling of various biomolecules (17). Advances in the use of gold nanoparticles have enabled better sensitivity, deeper tissue penetration, and enhanced detection capabilities in target tissues (17). Applications of gold nanoparticles include detecting point mutations, isolating mutations in hot spots, and identifying target proteins in genetic disorders. They are also instrumental in RNA interference technologies (RNAi), where specially designed RNA biomolecules target pathogenic gene products with precision. This ability to specifically target genetic elements underscores the transformative potential of nanoparticles in genetic engineering and gene therapy (17).
During their research, scientists concluded that nanoparticles could serve as carriers in conjunction with the CRISPR/Cas9 system. This intracellular process involves cellular uptake, enabling the transfer of Cas9 and single-guide RNA (sgRNA) to cellular targets, emerging as one of the most promising methods for treating various genetic diseases, including human cancers (18). However, translating CRISPR/Cas9 for cancer gene therapy requires the development of safe and efficient probes for precise delivery of Cas9 and sgRNA to tumors (18, 19). In recent studies, researchers developed novel core-shell nanostructures such as liposome-encapsulated hydrogel nanoparticles (LHNPs) optimized for the efficient delivery of Cas9 constructs and nucleic acids (19). These nanocarriers, including plasmids, allow entry into target cells via non-passive endocytosis, bypassing lysosomes to prevent degradation and damage (19). Researchers found that by combining sgRNA, Cas9, and mRNA, they could produce a complex known as ribonucleoprotein (RNP). This complex, upon cytoplasmic translation, induces genomic changes in target gene products or cells (19). Ribonucleoprotein complexes exhibit strong negativity when placed in positively charged environments, enhancing their stability and effectiveness (19).
Additionally, inorganic compounds such as chitosan sulfate, protamine, and dendrimers facilitate genome editing with increased accuracy and reduced error. These compounds enhance the efficiency of CRISPR/Cas9 systems, making genomic modifications more precise and reliable. This advancement underscores the significant potential of combining nanoparticles with CRISPR/Cas9 for effective gene therapy in cancer and other genetic disorders (19).
In addition, nanotechnology-based delivery systems are well-suited for encapsulating CRISPR/Cas9 components, protecting them from degradation by blood nucleases and proteases. This is critical because CRISPR/Cas9 components, as biomolecules, carry cargo with a negative charge, which affects their entry into cells (17, 18). Nanocarriers can be chemically adapted or modified to enhance their reactivity, ensuring more effective targeting and intracellular absorption, particularly at tumor sites (18). Researchers have described these nanocarriers as responsive to both internal biochemical stimuli, such as pH, ATP, and cellular microenvironments, and external probes, such as light, magnetic fields, ultrasound, and heat (19). These stimuli-responsive carriers allow CRISPR/Cas9 to selectively perform gene editing functions at precise locations and times, minimizing side effects and off-target activity (19). By leveraging this precision, CRISPR/Cas9 can control the level of gene expression by targeting transcription processes, enabling more accurate and safer gene modifications (18, 19). This approach demonstrates significant potential for reducing unintended disruptions in gene expression (19), thereby enhancing the therapeutic efficiency of CRISPR/Cas9 systems (18, 19).
4.6. Nanoparticles in Medicine
Nanoscience has become a significant force in medicine and treatment, particularly in cancer therapy (20). With the increasing global prevalence of cancer, referred to as a "cancer tsunami," advanced and innovative therapies are urgently needed (20). One such promising treatment is the use of nanorobots, which can transfer drugs directly to genes, gene products, or target cells with high precision and efficiency (20). Nanoparticles can be broadly categorized as organic or inorganic. Inorganic nanoparticles, such as metals or diamonds, and organic nanoparticles, including proteins and polynucleotides, each offer unique advantages (20). For example, diamond nanoparticles, due to their exceptional physical and chemical properties, demonstrate enhanced performance in targeted delivery, making them an ideal choice for constructing nanorobots (15, 20). In orthopedics, natural bone pores are about 100 nm wide. When artificial bone implant pores remain smooth, the body may trigger an immune response. By introducing nano-sized features on the surface of prostheses for hips or knees, osteoblast stimulation and bone matrix growth can be encouraged, improving implant integration (15, 20). Cancer treatment using nanoparticles has also advanced through photodynamic therapy (PDT). This method relies on the destruction of cancer cells using atomic oxygen generated upon exposure to cytotoxic laser beams (15, 20). Cancer cells absorb a specialized dye used in this process in much higher concentrations than healthy cells. When exposed to laser light, the cancer cells are selectively destroyed (14, 15, 20). However, a side effect of this therapy is that residual dye molecules migrate to the skin and eyes, making patients highly sensitive to sunlight for an average of six weeks (14, 15, 20). To mitigate this effect, the hydrophobic version of the dye molecule is encapsulated within porous nanoparticles, preventing its spread to non-target areas. Importantly, the nanoparticle design does not compromise the oxygen production capability. With a pore size of approximately 1 nanometer, oxygen can freely diffuse while avoiding systemic side effects (Figure 1) (14, 15, 20). This innovative use of nanoparticles represents a major breakthrough in precision medicine, improving treatment outcomes while minimizing side effects.
Types of nanoparticle complexes
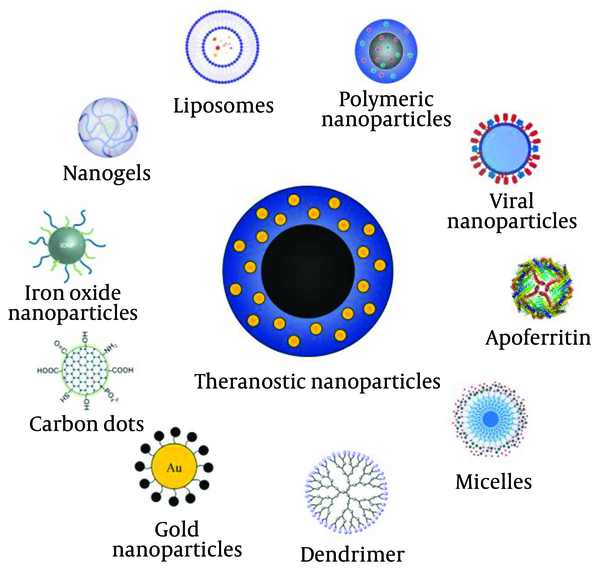
4.7. Nanoparticles in Cancer Treatment
While there are standard methods for diagnosing and treating cancer, the use of nanoparticles has emerged as a promising alternative due to their compatibility with the environment and reduced side effects compared to traditional treatment methods (15, 21, 22). This approach is based on a safe and consistent drug delivery system, often utilizing solid lipid nanoparticles and liposomes. These nanoparticles are designed to deliver drugs with high specificity to the target cell, gene, or gene product, ensuring that adequate drug concentrations reach the tumor site for a defined period while minimizing harmful effects on other organs (15, 21-23).
Solid lipid nanoparticles offer a complex system with unique advantages over other drug delivery systems (15, 21-23). They are capable of maintaining high drug specificity and reducing systemic toxicity. However, even short-term or single-dose use of these systems may pose risks to human health if not carefully managed. The use of biodegradable nanoparticles provides a potential solution, but prolonged or repeated use can still lead to unwanted side effects (15, 21-23).
Despite these benefits, challenges and limitations remain in the use of nanoparticles for medical applications. Issues such as high synthesis costs and suboptimal pharmacokinetic properties hinder the widespread adoption of nanoparticle-based therapies (15, 21). However, ongoing research and advancements in nanotechnology aim to address these limitations. With improved cost-effectiveness and enhanced pharmacokinetics, nanoparticles hold the potential to revolutionize cancer treatment by increasing efficiency and overcoming existing barriers (15, 21).
4.8. Application of Nanoparticle Complexes for Inhibition of Oncogenic Gene Expression in Cancer Cells
The use of Nanoparticle Complexes is very effective in increasing the lifespan of the complex, so, for example, Gold, Iron Oxide, Spion, Copper, Nickel, Manganese, Platinum, Diamond, Calcium Carbonate, etc. (24-26). Nanoparticles can be used. We will mention a few types of them. For example, it is used for the genetic aspect of CRC with a potential investigation (24-26). During the research, the studies show that most scientists focus on inhibiting the genes KRAS, Muc16, APC, TTN, TP53, ANKZF1, SNAI1, PPFIA4, SIX4, NOTUM, Oct-4, Sax2, P53, Abcg2, Nanog (24-27).
MicroRNAs such as miR-21 and miR-145 play critical roles in Cancer Biology, with miR-21 acting as an oncogene by targeting tumor suppressors like PTEN, and miR-145 functioning as a tumor suppressor by regulating oncogenes like KRAS and c-Myc. To enhance the therapeutic potential of miRNA inhibitors (antagomiRs) or mimics, Nanoparticles and Antioxidant Complexes are employed. For instance, siRNA-loaded Nanoparticles targeting miR-21 can efficiently silence its expression, while miR-145 mimics encapsulated in lipid-based or polymeric Nanoparticles restore its function in cancer cells. Additionally, Antioxidant Complexes such as Chrysin-Curcumin stabilize these miRNAs, protect them from degradation, and synergistically reduce oxidative stress, thereby enhancing their therapeutic efficacy. Combining Nanoparticles and Antioxidant Systems ensures improved delivery, stability, and bioavailability of miRNA-based therapies, making them more effective in cancer treatment (28-30).
For example, telomerase, telomeres is an essential therapeutic target in cancer cells, whose central role in tumor genesis is known (31). Nowadays, researchers are performing new therapeutic methods based on molecular therapeutic targets of bioactive substances such as Curcumin and Chrysin with fewer side effects than other chemical drugs (31). However, due to their low solubility in water and high clearance in the bloodstream, Nanoparticles can increase their half-life and biocompatibility (30, 31). Therefore, the purpose of using curcumin and chrysin nanoparticles is to suppress the expression of the telomerase gene in CRC cells (31).
Also, by using calcium carbonate nanoparticles and siRNA, they can suppress the interfering and angiogenic gene that causes Angiogenesis in CRC tissue and disrupt its gene expression to achieve this goal (26). We found that we can extract DNA from the Liposome Complex with the help of non-coding RNA, which researchers usually use siRNA targeting VEGF-C through CaCO3 Nanoparticles to significantly reduce the expression of VEGF-C, which leads to the fact that the factor Cervical Endothelial Growth is disturbed and suppressed (28, 29).
4.9. Nanoparticles in the Targeted Treatment of Colorectal Cancer
Or paying attention to the many advances of humankind in the cancer debate, but we still witness the resistance of this type of cancer, especially CRC, to current treatments such as chemotherapy and radiation therapy, for this purpose, using nanoparticles in the transfer of drugs to the target gene to increase the amount (24).
5. Discussion
The integration of chemotherapy and radiation therapy in CRC treatment has been shown to effectively target and reduce the expression of critical genes involved in Tumorigenesis, such as Oct4, Sax2, P53, Ras, Abcg2, and Nanog. These genes play vital roles in maintaining tumor cell growth, drug resistance, and the self-renewal capacity of cancer stem cells. By downregulating these genes, chemotherapy and radiation therapies reduce cancer cell proliferation, leading to improved clinical outcomes (23-27).
The addition of gold nanoparticles, combined with Hyperthermia and PDT, significantly enhances the therapeutic efficacy of these conventional treatments. Gold nanoparticles can be engineered to deliver targeted therapy directly to tumor cells, increasing the localized concentration of therapeutic agents while minimizing systemic toxicity. These Nanoparticles also have the potential to overcome biological barriers such as poor drug penetration and the presence of multidrug-resistant cancer cells. The Nanoparticles are particularly effective in targeting and disrupting cancer stem cells, which are often responsible for tumor recurrence and metastasis. by influencing cell cycle progression, particularly in the G1 and G0 phases, gold nanoparticles interfere with the proliferation of cancer stem cells and induce cell death (24, 25, 28).
Also, the synergistic effect of combining gold nanoparticles with Hyperthermia and PDT has been shown to disrupt the tumor microenvironment and enhance the penetration of light-based therapies. This multi-modal approach not only improves the delivery of therapeutic agents to tumor cells but also promotes the thermal ablation of cancer cells, leading to enhanced therapeutic outcomes. The use of gold nanoparticles significantly augments the effectiveness of primary treatments, including radiation and chemotherapy, and offers potential in treating both primary and invasive colorectal tumors. As these nanoparticles can be tailored to interact with specific molecular pathways and genes, their application could play a pivotal role in personalized medicine, providing a more targeted and efficient approach to CRC therapy. These findings suggest that the incorporation of Gold Nanoparticles with Hyperthermia and PDT represents a promising direction for future CRC treatments. Their ability to enhance Drug Delivery, target specific Cancer Cells, and improve the efficacy of traditional therapies may address key challenges such as drug resistance, poor tumor targeting, and systemic toxicity, making this approach a significant advancement in cancer treatment (27-31).
5.1. Conclusions
Cancer remains a hazardous and deadly disease worldwide. Most conventional cancer therapies, such as chemotherapy and radiation, are associated with significant side effects. Researchers have identified nanoparticles as a promising alternative for cancer therapy due to their effectiveness and reduced adverse effects.
With the advancements made in nanotechnology, it is predicted that nanoparticles will soon play a critical role in the treatment of various genetic and hereditary diseases, including cancers. These materials are particularly promising because they are compatible with the body’s biological conditions, exhibiting minimal or no side effects on both the body and the environment.
Therefore, it is suggested that future research, particularly in the biomedical field, prioritizes the development of nanoparticle-based treatments. These innovative therapies have the potential to replace conventional methods like chemotherapy and radiation therapy, which often cause irreversible side effects.
References
-
1.
Sadeghi Z, Maleki P, Mohammadi Bondarkhilli SA, Mohammadi M, Raheb J. Dataset on cytotoxicity effect of polyethylenimine-functionalized graphene oxide nanoparticles on the human embryonic carcinoma stem cell, NTERA2 cell line. Data Brief. 2019;26:104487. [PubMed ID: 31667251]. [PubMed Central ID: PMC6811929]. https://doi.org/10.1016/j.dib.2019.104487.
-
2.
DeFrates K, Markiewicz T, Gallo P, Rack A, Weyhmiller A, Jarmusik B, et al. Protein Polymer-Based Nanoparticles: Fabrication and Medical Applications. Int J Mol Sci. 2018;19(6). [PubMed ID: 29890756]. [PubMed Central ID: PMC6032199]. https://doi.org/10.3390/ijms19061717.
-
3.
Murray RW. Nanoelectrochemistry: metal nanoparticles, nanoelectrodes, and nanopores. Chem Rev. 2008;108(7):2688-720. [PubMed ID: 18558753]. https://doi.org/10.1021/cr068077e.
-
4.
Kordkatouli M, Sateei A, Dulskas A. Potential Roles and Mechanisms of Avena Sativa in Cancer Prevention. Multidisciplinary Cancer Investigation. 2024;8(2). https://doi.org/10.61186/mci.8.1.3.
-
5.
Belpomme D, Irigaray P, Hardell L, Clapp R, Montagnier L, Epstein S, et al. The multitude and diversity of environmental carcinogens. Environ Res. 2007;105(3):414-29. [PubMed ID: 17692309]. https://doi.org/10.1016/j.envres.2007.07.002.
-
6.
Amereh F, Jahangiri-Rad M, Mazloomi S, Rafiee M. The role of environmental and lifestyle factors in the incidence and prevalence of cancer. J Environ Health Engineering. 2017;4(1):30-42. https://doi.org/10.18869/acadpub.jehe.4.1.30.
-
7.
Rasool S, Rasool V, Naqvi T, Ganai BA, Shah BA. Genetic unraveling of colorectal cancer. Tumour Biol. 2014;35(6):5067-82. [PubMed ID: 24573608]. https://doi.org/10.1007/s13277-014-1713-7.
-
8.
Qudayr A, Kabel AM, AbdElmaaboud MA, Alghamdi WY, Alghorabi A. Colorectal Cancer: New Perspectives. J Cancer Res Treat. 2018;6(3):80-3.
-
9.
Kaltenbach T, Anderson JC, Burke CA, Dominitz JA, Gupta S, Lieberman D, et al. Endoscopic Removal of Colorectal Lesions-Recommendations by the US Multi-Society Task Force on Colorectal Cancer. Gastroenterol. 2020;158(4):1095-129. [PubMed ID: 32122632]. https://doi.org/10.1053/j.gastro.2019.12.018.
-
10.
Dezfuli AAZ, Abu-Elghait M, Salem SS. Recent Insights into Nanotechnology in Colorectal Cancer. Appl Biochem Biotechnol. 2024;196(7):4457-71. [PubMed ID: 37751009]. https://doi.org/10.1007/s12010-023-04696-3.
-
11.
Cummings BJ. Adjuvant radiation therapy for colorectal cancer. Cancer. 1992;70(5 Suppl):1372-83. [PubMed ID: 1511387]. https://doi.org/10.1002/1097-0142(19920901)70:3+<1372::aid-cncr2820701527>3.0.co;2-a.
-
12.
Singh S, Kumar NK, Dwiwedi P, Charan J, Kaur R, Sidhu P, et al. Monoclonal Antibodies: A Review. Curr Clin Pharmacol. 2018;13(2):85-99. [PubMed ID: 28799485]. https://doi.org/10.2174/1574884712666170809124728.
-
13.
Yu X, Trase I, Ren M, Duval K, Guo X, Chen Z. Design of Nanoparticle-Based Carriers for Targeted Drug Delivery. J Nanomater. 2016;2016. [PubMed ID: 27398083]. [PubMed Central ID: PMC4936496]. https://doi.org/10.1155/2016/1087250.
-
14.
Guduru R, Liang P, Runowicz C, Nair M, Atluri V, Khizroev S. Magneto-electric nanoparticles to enable field-controlled high-specificity drug delivery to eradicate ovarian cancer cells. Sci Rep. 2013;3:2953. [PubMed ID: 24129652]. [PubMed Central ID: PMC3797424]. https://doi.org/10.1038/srep02953.
-
15.
Khang D, Carpenter J, Chun YW, Pareta R, Webster TJ. Nanotechnology for regenerative medicine. Biomed Microdevices. 2010;12(4):575-87. [PubMed ID: 19096767]. https://doi.org/10.1007/s10544-008-9264-6.
-
16.
Kasala D, Yoon AR, Hong J, Kim SW, Yun CO. Evolving lessons on nanomaterial-coated viral vectors for local and systemic gene therapy. Nanomedicine (Lond). 2016;11(13):1689-713. [PubMed ID: 27348247]. [PubMed Central ID: PMC5561791]. https://doi.org/10.2217/nnm-2016-0060.
-
17.
Rosi NL, Giljohann DA, Thaxton CS, Lytton-Jean AK, Han MS, Mirkin CA. Oligonucleotide-modified gold nanoparticles for intracellular gene regulation. Science. 2006;312(5776):1027-30. [PubMed ID: 16709779]. https://doi.org/10.1126/science.1125559.
-
18.
Chen Z, Liu F, Chen Y, Liu J, Wang X, Chen AT, et al. Targeted Delivery of CRISPR/Cas9-Mediated Cancer Gene Therapy via Liposome-Templated Hydrogel Nanoparticles. Adv Funct Mater. 2017;27(46). [PubMed ID: 29755309]. [PubMed Central ID: PMC5939593]. https://doi.org/10.1002/adfm.201703036.
-
19.
Zhou S, Li Y, Wu Q, Gong C. Nanotechnology‐based CRISPR/Cas9 delivery system for genome editing in cancer treatment. MedComm–Biomaterials Applications. 2024;3(1). https://doi.org/10.1002/mba2.70.
-
20.
Bayda S, Adeel M, Tuccinardi T, Cordani M, Rizzolio F. The History of Nanoscience and Nanotechnology: From Chemical-Physical Applications to Nanomedicine. Molecules. 2019;25(1). [PubMed ID: 31892180]. [PubMed Central ID: PMC6982820]. https://doi.org/10.3390/molecules25010112.
-
21.
Doyle D. Nanotechnology in Anesthesia and Medicine. Adv Anesth. 2013;31(1):181-200. https://doi.org/10.1016/j.aan.2013.08.010.
-
22.
Gao Y, Xie J, Chen H, Gu S, Zhao R, Shao J, et al. Nanotechnology-based intelligent drug design for cancer metastasis treatment. Biotechnol Adv. 2014;32(4):761-77. [PubMed ID: 24211475]. https://doi.org/10.1016/j.biotechadv.2013.10.013.
-
23.
Kumari Singh S, Solanki R, Patel S. Nano pharmaceutical delivery in combating colorectal cancer. Med Drug Discovery. 2024;21. https://doi.org/10.1016/j.medidd.2023.100173.
-
24.
Pedrosa P, Corvo ML, Ferreira-Silva M, Martins P, Carvalheiro MC, Costa PM, et al. Targeting Cancer Resistance via Multifunctional Gold Nanoparticles. Int J Mol Sci. 2019;20(21). [PubMed ID: 31694227]. [PubMed Central ID: PMC6861975]. https://doi.org/10.3390/ijms20215510.
-
25.
Cisterna BA, Kamaly N, Choi WI, Tavakkoli A, Farokhzad OC, Vilos C. Targeted nanoparticles for colorectal cancer. Nanomedicine (Lond). 2016;11(18):2443-56. [PubMed ID: 27529192]. [PubMed Central ID: PMC5619175]. https://doi.org/10.2217/nnm-2016-0194.
-
26.
Mehdinejad S, Peymani M, Salehzadeh A, Zaefizadeh M. Genetic insights and therapeutic potential for colorectal cancer: mutation analysis of KRAS gene and efficacy of Oleuropein-conjugated iron oxide nanoparticles. Naunyn-Schmiedeberg's Arch Pharmacol. 2024;397(11):8771-83. https://doi.org/10.1007/s00210-024-03182-9.
-
27.
Kordkatouli M, CHO WC, Mohammad Bondarkhilli SA, Dulskas A, Qureshi SAM. Oct-4 and Its Role in the Oncogenesis of Colorectal Cancer. Middle East J Cancer. 2024;15(2_Supplement).
-
28.
He XW, Liu T, Xiao Y, Feng YL, Cheng DJ, Tingting G, et al. Vascular endothelial growth factor-C siRNA delivered via calcium carbonate nanoparticle effectively inhibits lymphangiogenesis and growth of colorectal cancer in vivo. Cancer Biother Radiopharm. 2009;24(2):249-59. [PubMed ID: 19409048]. https://doi.org/10.1089/cbr.2008.0515.
-
29.
Mohammadi Bondarkhilli SA, Kordkatouli M, Maroufi M, Dulskas A. Oncogenic and anticancer roles of miRNAs in colorectal cancer: A review. Micro Nano Bio Aspects. 2024;3(1):14-22. https://doi.org/10.22034/mnba.2024.429195.1053.
-
30.
Kordkatouli M, Mohammadi Bondarkhilli SA, Sateei A, Mahmood Janlou MA. Roles of miR-21 in the Onset and Advancement of Colorectal Cancer (CRC). Multidisciplinary Cancer Investigation. 2024;8(1):0. https://doi.org/10.61186/mci.8.1.3.
-
31.
Bagheri R, Sanaat Z, Zarghami N. Synergistic Effect of Free and Nano-encapsulated Chrysin-Curcumin on Inhibition of hTERT Gene Expression in SW480 Colorectal Cancer Cell Line. Drug Res (Stuttg). 2018;68(6):335-43. [PubMed ID: 29294495]. https://doi.org/10.1055/s-0043-121338.