Abstract
Background:
Electromagnetic fields (EMF) have teratogenic effects during the embryonic development. In current study, histopathological and physiological effects of sinusoidal EMF on the brain were investigated. We sought to determine the apoptosis level and changes in blood brain barrier permeability in brain tissue of pre-incubated white leghorn hen eggs in the field of EMF.Materials and Methods:
In this experimental study, 300 healthy, fresh, and fertilized eggs (55-65 g) were divided into experimental (3 groups, N=50), control (N=75) and sham (N=75) groups. Experimental eggs (inside the coil) were exposed to 3 different intensities of 1.33, 2.66 and 7.32 mT and sham groups were also located inside the same coil but with no exposure, for 24 hrs before incubation. Control, sham and experimental groups were incubated in an incubator (38±0.5ºC, 60% humidity). Brains of 14 day old chicken embryos of all groups were removed, fixed in formalin (10%), stained with H & E and TUNEL, apoptotic cells were studied under light microscope. Brains of other embryos were prepared for scanning electron microscope. By injections of Evans blue, any possible changes in brain vessels were also investigated.Results:
Our results showed electromagnetic fields have toxic effects on cell organelles and cell membranes. EMF would increase the level of cellular apoptosis in the brain. They also would tear up the blood vessels. Thereafter, they would affect the permeability of blood brain barrier of exposed chicken embryos.Conclusion:
These findings suggest that electromagnetic fields induce different degrees of brain damages in chicken embryos brain tissue.Keywords
Brain Apoptosis Blood brain barrier Electromagnetic field Chicken embryo
Introduction
There are many reports about the teratogenic effects of chemical and physical agents on embryo’s brain apoptosis. (Electric, Magnetic and Electromagnetic fields) EF, MF, and EMF as physical agents have negative effects during the embryonic development. Much of the present studies show the effects of EMF particularly sinusoidal fields are around 50/60 Hz [1] and also, increased risk of brain turnouts, a disrupted learned behavior etc, and an increase in chick embryo mortality as result of cell phone radiations is reported [2]. The question arises as to whether there is a particular sensitivity of the neural tissues of the brain to electromagnetic fields such as that which is produced by cell phones [2-4].
Mouse embryonic stem (ES-derived nesting-positive neural progenitor) cells were used as an experimental model to study the effects of (EMF). The results show that EMF exposure of ES-derived neural progenitor cells transiently affects the transcript level of genes related to apoptosis and cell cycle control [4]. Rats acutely exposed to a 60 Hz sinusoidal magnetic field showed an increase in DNA single and double-strand breaks and also apoptosis and necrosis in their brain cells. DNA strand breaks, DNA-protein cross-links, and activation of protein kinas C; increase the production of HSP (heat shock proteins); and alter calcium homeostasis in cells [5]. In vivo, rats exposed to microwaves from GSM (Global System for Mobile Communication) mobile phones revealed nerve cell damage due to an increased albumin intake across the blood-brain barrier. Several authors have reported that electromagnetic exposure alters blood brain barrier (BBB). Other authors have reported difficulties in confirming these findings [6, 7]. Disagreement among investigators might be due to lack of an assay of BBB permeability that combines sensitivity and specificity.
Present study based on many reports about the effects of EMFs on brain [2-4] and post incubated chicken embryos [8] covers the effects of EMFs on preincubated chick embryos [9, 10] for the first time.
Pay attention to chick embryo development in pre and post hatching, preincubation period coincides with a short interval between these two terms. So, study the effective intensities of EMF on chick embryo at 24 h preincubation would be an appropriate experiment for examination of the effect of these fields on apparently inactive and undifferentiated cells (pre-primitive streak stage). The aim of this study is to find out the most negative effect intensities of EMF [9, 10] on brain and to report brain anomalies during the development such as nerve cell damage, changes in level of apoptosis and increase of blood brain barrier permeability and also probably relation between these two processes using light and electron microscope (SEM) in preincubated white leghorn chicken embryos.
Materials and Methods
In this experimental study, we used histopathological, physiological and cellular methods in this study. All experimental animal procedures were conducted with the approval of the Ethics Committee of the Shahid Beheshti University of Medical Sciences of Iran.
Subjects: Three hundred healthy, fresh fertilized white leghorn hen eggs (55-65 g, from Bonyad Mostazaffan farm, Karaj, Iran) were transported to the laboratory immediately after collection. They were selected and stored horizontally at room temperature (10-15ºC) for maximum of 60 h.
EMF exposure: EMF source was a cylindrical coil, 42 cm in length (L), with diameter of 9.6 cm inside and 11.5 cm outside, made of 980 turn of 2.5 mm diameter enameled copper wire (N). Values in the center of the coil were calculated according to: B =µºNI/L (µº=1.256×10 -6), where “B” represents flux densities and “I” represents density of electrical current applied to the coil. To determine values of magnetic fields at distances of 5 and 10 cm from the center of coil, “K” was measured as: K5=0.9427 and K10=0.9079 (B=KI).
To create one-way wave and regulate different flux densities, equipment with one key (for both activating and deactivating processes), one round screw (for influx of electrical currents) and two exits for AC and DC currents, was used. Connections between AC exit (used in present investigation) and coil was established through a dividing box. Two digital ampermeters showed flux densities.
Coil was located in an east-west direction, magnetic flow was parallel to long axis of embryo’s body (longitude 351,400 and latitude 511,250) and perpendicular to long axis of eggs [8-10]. A system of air conditions (one, 5 cm from the entrance of coil and others beneath the coil), ventilated coil chamber. Frequency was always 50 Hz, with 3 most effective flux intensities of 1.33, 2.66 and 7.32 mT (milli Tesla) [9, 10].
A total of 300 healthy, fresh fertilized eggs were divided into experimental 1, 2, 3 (N=50), control (N=75) and sham (N=75) groups. Experimental groups (inside the coil) were exposed to 3 different intensities (1.33, 2.66 and 7.32 mT) for a period of 24 h before incubation. Unexposed-control, sham-exposed (inside the coil, with no exposure, for 24 h) and exposed-groups were incubated in an incubator (38±0.5ºC, 60% humidity) for 14 consecutive days (stage 40; Hamburger-Hamilton, 1951), embryos were removed from their shells, washed in physiological serum, embryonic membranes were separated from umbilical cord blood vessels using fine needle.
Hematoxylin and eosin and TUNEL staining: Morphological structures of the embryos were carefully studied, normal and abnormal brains were randomly separated from normal and abnormal embryos, fixed in formaldehyde, sectioned (4-5 µ) for Hematoxylin and Eosin (H&E) and TUNEL staining. H&E staining method was down in three stages, deparafination, hydration and staining (staining procedure: Immerse sections in the filtered Harris Hematoxylin for 1 min.
Rinse with tap water/ exchange tap water until the water is clear/ immerse sections in Eosin stain for 1-2 min / rinse with tap water/exchange tap water until the water is clear/dehydrate in ascending alcohol solutions (50%, 70%, 80%, 95% ×2, 100% ×2) clear with xylem (×2) and also, TUNEL staining was down by in its of cell death detection kit was purchased from Roche Applied Science (Germany) in three stages, Paraffin-embedded sections, frozen sections and labeling and detection (staining Procedure: Remove paraffin by incubating slides for 3 minute each in: 4× xylem-100% eton - 96% eton-80% eton / transfer slides to a tray and wash 4 × 3 min in H2O / wash slides 3 minute in TBS and carefully remove liquid / add 200 µL proteins K (10 µg/mL) to completely cover the section and incubate 15 min / wash slides 2 x 3 minute in TBS / fix frozen sections in 4% PFA in TBS for 20 minute / wash slides 30 minute in TBS / Add 200 µL 0.1% triton X-100 to completely cover the section and incubate 2 minute. / Wash slides 2×3 minute in TBS and carefully remove liquid / Add 10-20 µL TUNEL-Mix and cover section with a cover slip. Incubate 60 minute at 37ºC in the dark / wash slides 3×3 minute in TBS and carefully remove liquid / add 50-100 µL PI (1 µg/mL) to completely cover the section and incubate 5 min / Remove PI (toxic waste) and wash slides 5 minute in TBS).
Evans blue injection: Vascular permeability was quantitatively evaluated by Evans blue dye, was obtained from sigma (Germany). Two percent Evans blue dye in saline was injected into chorioallantoic vessels as a BBB permeability tracer on day 13 of embryonic development; any possible changes in brain blood vessels were also investigated on day 17. Samples were weighted and homogenized in 50% trichloroacetic acid solution. The supernatant was obtained by centrifugation, was measured for absorption of Evans blue by spectrophotometer (610 nm) [11].
Preparation for scanning electron microscope (SEM): Some of brains of 14 day old chicken embryos of all groups (control, sham and experimental groups) were fixed in 2.5% glutharaldehyde (41ºC) for scanning electron microscope (SEM) studies (Electron Microscope Center, Department of Anatomy, Medical School, University of Shahid Beheshti, Tehran, Iran) after removing.
Statistical Analysis: Statistical analysis was conducted with the use of one-way ANOVA for intergroup comparisons. Differences with p<0.05 (Mean±SEM) were considered statistically significant. Results were charted in Excel, using software of SPSS-16.
Results
Histopathological studies: H & E staining indicated differences between groups. These differences were more considerable in exposure groups compared to control and sham group. In the control and sham groups brain cells and also vessels were normal (Fig. 1A, 1B), while abnormal distribution of neural cells and damaged vessels were observed in experimental groups (Fig. 1C-1E).
H & E staining of brain of 14-day old white leghorn chick embryos with normal structure and blood vessel (A & B) experimental groups with abnormal structure, destroyed vessel and released red blood cells in tissue (C-E), black arrows and circles are red blood cells and red arrows are blood vessels (400×)
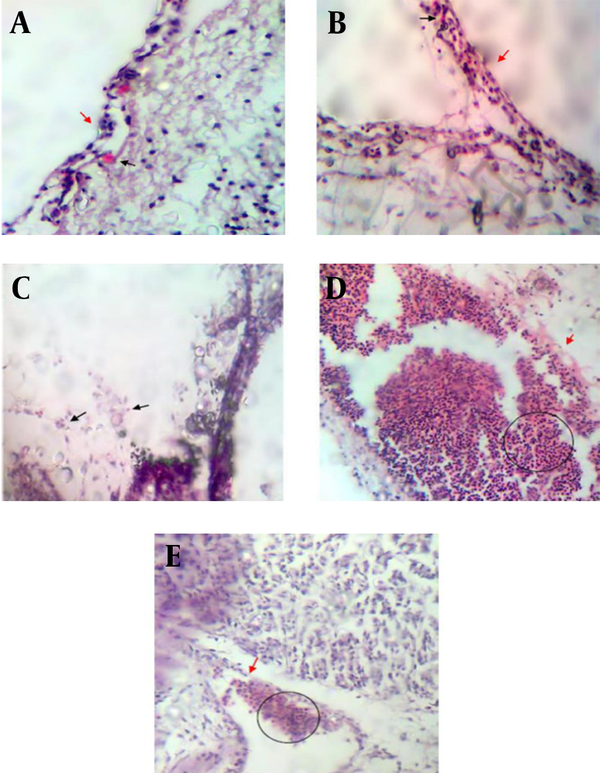
Histopathological investigations of 14 day old white leghorn chick embryo’s brain after exposure to the 50 Hz electromagnetic field (3 different intensities 1.33, 2.66 and 7.32 mT) showed that number of apoptotic cells in experimental groups were more than control and sham group. Actually, apoptosis was observed in unexposure groups (control and sham) too (Fig. 2A, 2B), but in experimental groups this process significantly increased by electromagnetic field exposure. (Fig. 2C-2E, Fig. 3).
BBB permeability: At the first time, 7 day old embryos were selected for Evans blue injection and opened at day 14. This experiment repeated again and again, but all samples were died after injection. Injection was tested for 8, 10, 11, 12 and 13 day old embryos too, and all of them were died after injection except 13-day chick embryo. Also removing day for Evans blue measurement in embryo’s brain was selected by try and error. The results of BBB-permeability of albumin in 17 day old white leghorn chick embryos exposed to 3 different intensities of electromagnetic fields showed a significant effect compared to control and sham especially in 7.32 and 2.66 mT intensities.
Apoptosis of brain of 14 day old white leghorn chick embryos of control and sham groups contain a few apoptotic cells (A and B), experimental groups contain more apoptotic cells (C-E), apoptotic cells are dark brown and around shape (A, B, C, D, and E ,black arrow) (1000×), (TUNEL staining)
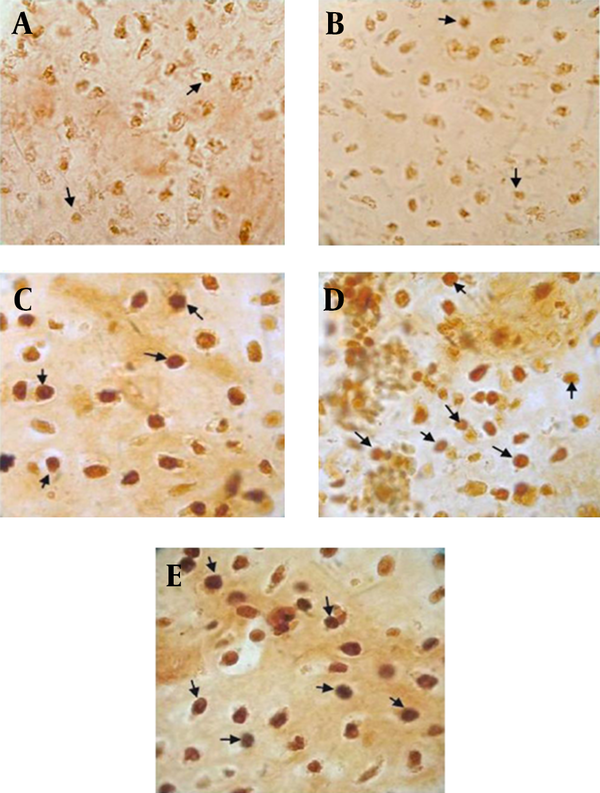
The number of died cell per field in various groups (* p<0.05)
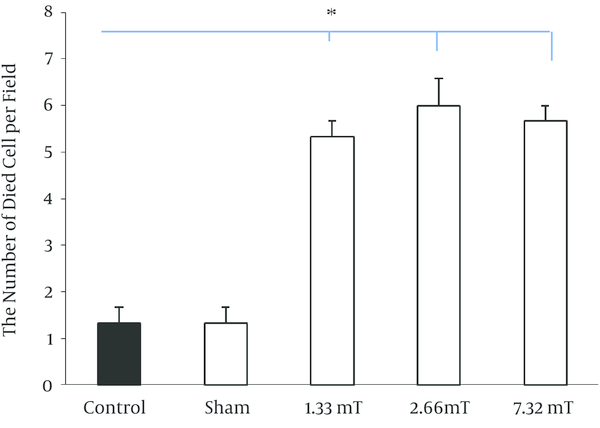
In experimental groups, BBB permeability of albumin (by Evans blue) was more than control and sham groups. Brain edema formation was associated with increased BBB permeability during 24 h. In the control and sham groups, EB concentration in cerebral tissue was 3.98±1.8 and 4.2±1.9 µg/g brain tissue, respectively. Administration of electromagnetic field at doses 2.66 mT was associated with an increase in EB extravasations in the brain tissue. This effect was not observed at the 1.33 and 7.32 mT electromagnetic pre-incubation dose significantly (Fig. 4).
Evans blue dye content of brain tissue following injection to corioalantoic vessels. Columns represent Evans blue dye content (µg/g, mean±SEM) of tissue samples obtained from 17 days old chicken embryo brain tissue. *Statistical analysis showed that the differences between control and sham groups and treatment groups as well as among the treatment groups are significant (p=0.05, LSD test).
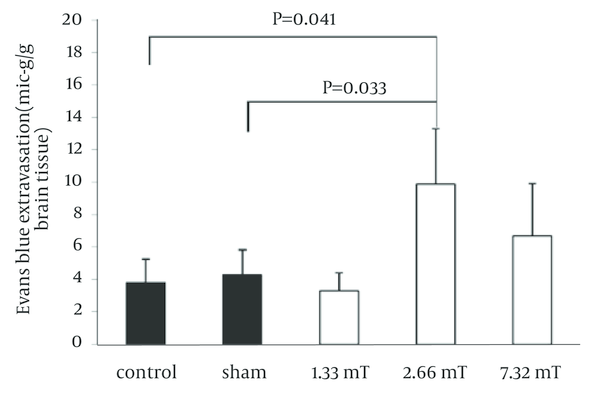
SEM of vessel of brain of 14 day old white leghorn chick embryos of control and sham groups with healthy vessel (A, B), experimental groups with damaged vessel (C-E), numerous erythrocytes (C, black circle), fibrinogen (C,E, black arrow), platelet (D, E, white arrow) (A-E, scale bar,50 mm)
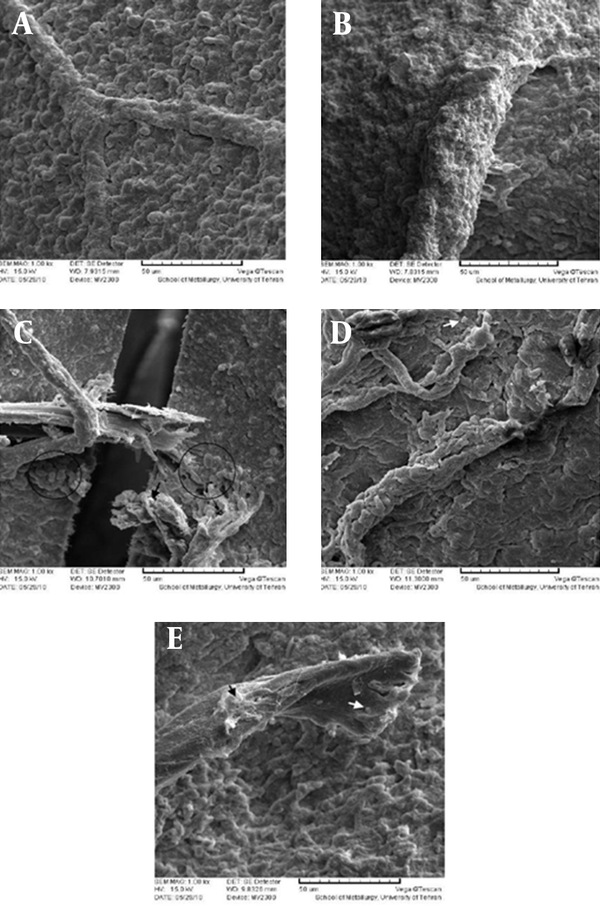
SEM studies: At SEM studies the normal vessel were observed in control and sham groups of brains of 14 day old white leghorn chicken embryos (Fig. 5A, 5B), while in experimental groups, it was completely disrupted and caused to release the erythrocytes, platelet and fibrinogen inside brains tissue (Fig. 5C-5E).
Discussion
H&E staining indicated differences between groups. Also the considerable apoptosis in exposure groups were observed. The results of BBB permeability studies showed a significant effect in 7.32 and 2.66 mT intensities. At SEM studies the disrupted vessels and hemorrhagia were observed in experimental groups.
During embryos development, CNS is particularly sensitive. Observations confirm glycosaminoglycans disintegrations, abnormal spaces, necrotic zones and an increase in number of mesenchymal cells of head region of 48 h embryos exposed to EMF which would cause malformations in head regions [12]. It is clear from our study that some intensities of electromagnetic field have drastic effects on brain tissue as prove represented thoroughly by histopathological changes in this research (normal neurons and neuroglia in control and sham groups, neural cell abnormalities in exposure groups) [8]. Our findings (about abnormalities in head region) are inconsistent with studies covering post-incubated chick embryos [9, 10]
We could observe the apoptosis in all groups. While, it was more considerable in exposure groups and our statistical analysis verifies this observation. Pay attention to critical role of apoptotic processes in brain development especially in correct differentiation and/or synaptogenesis [13] the effect of EMF on apoptosis during development is important. Lai and Singh showed exposure of a 60 Hz magnetic field at 0.01 mT for 24 h on rat caused a significant increase in DNA single- and double-strand breaks. Prolonging the exposure to 48 h caused a larger increase. And also they expressed, acute magnetic field exposure increases apoptosis and necrosis of brain cells in the rat [5] (in our previous study we reported that 50 Hz EMF for 24 h on chicken embryo in preincubation had destructive effects on nucleus and endoplasmic reticulum of neurons) [10]. The apoptosis can be initiated by stress on cellular organelles, including mitochondria and ER (endoplasmic reticulum). ER stress, including the disruption of ER calcium homeostasis and accumulation of excess proteins, induce apoptosis by the activation of ER-localized caspase-12. The ER-stress-related activation of caspase-12 has been detected in experimental models of neurodegenerative disease. Data from human studies suggest that Bcl-2 family proteins might also participate in the regulation of the stress response by interacting with HSPs. Apoptosis can also be regulated by intracellular signal transduction pathways [14]. Considerable evidences suggest that brief electromagnetic fields exposures activate several components of stress response pathway in cells and tissues. Activation of transcription factors is the first steps in induction of stress proteins. Increase in mRNA transcript factors, such as HSP72, also follows the EMFs stimulations [10]. GSM (1800 MHz) radiation was shown to activate the HSP27/p38MAPK stress response pathway in human endothelial cells [4].
The blood brain barrier is responsible for the maintenance of the neuronal microenvironment. This is accomplished by isolation of the brain from the blood by the tight junctions that join endothelial cells in cerebral micro vessels and by selective transport and metabolism of substances from blood or brain by the endothelial cells [15]. Endothelial blood-brain barrier tight junctions differ from epithelial tight junctions, not only by distinct morphological and molecular properties, but also by the fact that endothelial tight junctions are more sensitive to micro environmental than epithelial factors. Signaling pathway involved in tight junction regulation include G-protein, extra and intracellular calcium levels, camp levels, proteases and cytokines. Common to most of these pathways is the modulation of cytoskeleton elements and the connection of tight junctions trans-membrane molecules to the cytoskeleton [16]. Chaplains, one of important enzyme families that contribute to apoptotic cell death after brain injury, are calcium-dependent proteases with many cellular targets including cytoskeleton elements [14].
There are reports about the effect of EMF on membrane of neuron current. The depressing effect of static magnetic fields (SMF) and low frequency electromagnetic fields (LF-EMF) on specific electrical conductivity (SEC) of CaCl2 solutions has been documented. The effect of LF-EMF on SEC is frequency-dependent, with the most pronounced effect appearing at 4 Hz. At the same time, it was shown that SMF-treated physiological solution (PS) caused the elevation of intracellular camp-dependent Nance exchange by pushing out Camions and accumulating Na ions. This leads to the argument of Ach-induced current through the membrane of intracellular perused neurons. However, the effect of LF EMF-treated PSs on chemosencitivity of the whole neurons is not clear [17].
In response to the changes in physiological and behavioral state, it is possible that regulated opening of the BBB is a critical component for proper brain function. Therefore, after observation the negative effect of EMF on chicken embryos brain development, we focused our study on the BBB development. Exposure to EMF [GSM 1800 standard] increased BBB permeability for 14C-sucrose significantly compared to unexposed samples in vitro [18]. Levin reported rat BBB permeation of sucrose to be 1.2×10 -7 cm/s in vivo [19].
In present study, the permeability of BBB was investigated by scanning electron microscope (SEM) and Evans blue injection. In experimental groups stained by H & E method we could observe vessels injury that was established by SEM study. The BBB permeability to Evans blue was observed in all groups because this barrier does not develop completely at 17-day old chicken embryo [20, 21]. But increased absorption of Evans blue by exposure groups (2.66 and 7.32 mT intensities) was considerable that statistical analysis verifies this observation.
Based on our previous conclusion about the negative effect of three different intensities (used in this project) on nucleus, endoplasmic reticulum and Golgi system (have main role for protein processing) in brain cells, our observation about the increasing of apoptotic cells due to the EMF exposure, some data about the ER stress, including the disruption of ER calcium homeostasis and accumulation of excess proteins, that induces apoptosis, and also the importance of extra and intracellular calcium levels as a signaling pathway involved in tight junction regulation [9], we can conclude Tight junctions as a principal protein part of BBB [22] could damage by EMFs exposure. Ultimately, we hope that present report could be a guideline for other investigations about EMF, brain and BBB, in future.
Acknowledgements
References
-
1.
Coulton LA, Barker AT. The effect of low-frequency pulsed magnetic fields on chick embryonic growth. Physics in Medicine and Biology. 1991;36(3):369-81. https://doi.org/10.1088/0031-9155/36/3/005.
-
2.
Ingole IV, Ghosh SK. Cell phone radiation and developing tissues in chick embryo: A light microscopic study of kidneys. J Anat Soc India. 2006;55(2):7-12.
-
3.
Beason RC, Semm P. Responses of neurons to an amplitude modulated microwave stimulus. Neuroscience Letters. 2002;333(3):175-8. https://doi.org/10.1016/s0304-3940(02)00903-5.
-
4.
Nikolova T, Czyz J, Rolletschek A, Blyszczuk P, Fuchs J, Jovtchev G, et al. Electromagnetic fields affect transcript levels of apoptosis-related genes in embryonic stem cell-derived neural progenitor cells. FASEB J. 2005;19(12):1686-8. [PubMed ID: 16116041]. https://doi.org/10.1096/fj.04-3549fje.
-
5.
Lai H, Singh NP. Magnetic-Field-Induced DNA Strand Breaks in Brain Cells of the Rat. Environmental Health Perspectives. 2004;112(6):687-94. https://doi.org/10.1289/ehp.6355.
-
6.
Persson BR, Salford LG, Brun A. Blood-brain barrier permeability in rats exposed to electromagnetic fields used in wireless communication. Wirel Netw. 1997;3(6):455-61. https://doi.org/10.1023/a:1019150510840.
-
7.
Williams WM, Platner J, Michaelson SM. Effect of 2450 MHz microwave energy on the blood-brain barrier to hydrophilic molecules. C. Effect on the permeability to [14C]sucrose. Brain Res. 1984;7(2):183-90. https://doi.org/10.1016/0165-0173(84)90023-7.
-
8.
Ubeda A, Trillo MA, Chacon L, Blanco MJ, Leal J. Chick embryo development can be irreversibly altered by early exposure to weak extremely-low-frequency magnetic fields. Bioelectromagnetics. 1994;15(5):385-98. [PubMed ID: 7802707].
-
9.
Lahijani MS, Nojooshi SE, Siadat SF. Light and electron microscope studies of effects of 50 Hz electromagnetic fields on preincubated chick embryo. Electromagn Biol Med. 2007;26(2):83-98. [PubMed ID: 17613036]. https://doi.org/10.1080/15368370601185888.
-
10.
Lahijani MS, Tehrani DM, Sabouri E. Histopathological and ultrastructural studies on the effects of electromagnetic fields on the liver of preincubated white Leghorn chicken embryo. Electromagn Biol Med. 2009;28(4):391-413. [PubMed ID: 20017630]. https://doi.org/10.3109/15368370903287689.
-
11.
Aoki T, Sumii T, Mori T, Wang X, Lo EH. Blood-brain barrier disruption and matrix metalloproteinase-9 expression during reperfusion injury: mechanical versus embolic focal ischemia in spontaneously hypertensive rats. Stroke. 2002;33(11):2711-7. [PubMed ID: 12411666].
-
12.
Delgado JM, Leal J, Monteagudo JL, Gracia MG. Embryological changes induced by weak, extremely low frequency electromagnetic fields. J Anat. 1982;134(Pt 3):533-51. [PubMed ID: 7107514].
-
13.
Saunders RD, Jefferys JG. Weak electric field interactions in the central nervous system. Health Phys. 2002;83(3):366-75. [PubMed ID: 12199550].
-
14.
Zhang X, Chen Y, Jenkins LW, Kochanek PM, Clark RS. Bench-to-bedside review: Apoptosis/programmed cell death triggered by traumatic brain injury. Crit Care. 2005;9(1):66-75. [PubMed ID: 15693986]. https://doi.org/10.1186/cc2950.
-
15.
Farrell CL, Risau W. Normal and abnormal development of the blood-brain barrier. Microsc Res Tech. 1994;27(6):495-506. [PubMed ID: 7516744]. https://doi.org/10.1002/jemt.1070270604.
-
16.
Wolburg H, Lippoldt A. Tight junctions of the blood-brain barrier: development, composition and regulation. Vascul Pharmacol. 2002;38(6):323-37. [PubMed ID: 12529927].
-
17.
Ayrapetyan SN, Hunanyan A, Hakobyan SN. 4 Hz EMF treated physiological solution depresses Ach-induced neuromembrane current. Bioelectromagnetics. 2004;25(5):397-9. [PubMed ID: 15197765]. https://doi.org/10.1002/bem.20025.
-
18.
Schirmacher A, Winters S, Fischer S, Goeke J, Galla HJ, Kullnick U, et al. Electromagnetic fields (1.8 GHz) increase the permeability to sucrose of the blood-brain barrier in vitro. Bioelectromagnetics. 2000;21(5):338-45. [PubMed ID: 10899769].
-
19.
Levin VA. Relationship of octanol/water partition coefficient and molecular weight to rat brain capillary permeability. J Med Chem. 1980;23(6):682-4. [PubMed ID: 7392035].
-
20.
Franke H, Ringelstein EB, Stogbauer F. Electromagnetic fields (GSM 1800) do not alter blood-brain barrier permeability to sucrose in models in vitro with high barrier tightness. Bioelectromagnetics. 2005;26(7):529-35. [PubMed ID: 16142784]. https://doi.org/10.1002/bem.20123.
-
21.
Bertossi M, Roncali L, Nico B, Ribatti D, Mancini L, Virgintino D, et al. Perivascular astrocytes and endothelium in the development of the blood-brain barrier in the optic tectum of the chick embryo. Anat Embryol (Berl). 1993;188(1):21-9. [PubMed ID: 8214622].
-
22.
Engelhardt B. Development of the blood-brain barrier. Cell Tissue Res. 2003;314(1):119-29. [PubMed ID: 12955493]. https://doi.org/10.1007/s00441-003-0751-z.