Abstract
Background:
The currently approved treatment for lead toxicity is chelation therapy to reduce the burden of the toxic effects of lead, but the safety and efficacy of the various chelating agents may be questioned.Objectives:
This study was aimed to evaluate the effects of caffeic acid, a dietary non-flavonoid phenolic acid, on lead accumulation and lead-induced oxidative stress in mice.Materials and Methods:
In this experimental study, 24 mice were divided into four groups. Group 1 served as control. Mice in group 2 received water containing 1000 ppm lead acetate. Group 3 animals received caffeic acid (60 mg/kg body weight) i.p. during lead treatment. Mice in group 4 only received caffeic acid. At the end of the experiment (18 days), blood samples were drawn and the levels of lead and some oxidative-stress related parameters were measured.Results:
Blood Pb concentration increased significantly in group 2 as compared to control group. Lead exposure caused significant increase of malondialdehyde and decrease of glutathione concentrations in erythrocyte haemolysate as compared to control group. Although caffeic acid was effective in normalization of the attenuated levels of erythrocytic glutathione, its administration had no significant effect in decreasing the augmented levels of erythrocytic malondialdehyde in group 3. Values of other measured parameters including erythrocytic activities of glutathione peroxidase and superoxide dismutase did not change significantly among experimental groups.Conclusions:
Present results show some beneficial effects of caffeic acid against lead poisoning and it can be thus proposed as a potential prophylactic treatment for amelioration of lead toxicity.Keywords
1. Background
Lead (Pb), a common environmental pollutant of worldwide concern, is well-known as a highly toxic agent. It can induce serious adverse health effects including neurological, gastrointestinal, reproductive, renal, hepatic, circulatory and hematological dysfunctions [1]. The currently approved treatment for Pb toxicity is chelation therapy to reduce the burden of the toxic effects of Pb, but the safety and efficacy of the various chelating agents may be questioned [2, 3]. It has been reported that chelating agents may produce a toxic potential themselves and also are incapable of alleviating some toxic effects of lead [3, 4]. Hence, potentially safe alternatives for the treatment of lead poisoning would have very important health-related consequences.
Lead-induced oxidative damage has been proposed as one of the important mechanisms of lead-related pathologies [1]. Several mechanisms have been proposed to mediate the oxidative stress caused by lead, mostly associated with disrupted prooxidant/antioxidant balance. Accumulated evidence of lead’s capacity to induce oxidative stress suggests that lead toxicity may be mitigated by improving the cellular availability of antioxidant agents [1]. It has been proposed that usage of an antioxidant compound in the presence of a chelator will improve the efficacy of the lead poisoning treatment [1, 5]. Hence, it can be presumed that natural compounds with both chelating and antioxidant activities could be good candidates for mitigating adverse effects of lead [2, 5, 6].
Caffeic acid (CA) is a well-known dietary phenolic compound abundantly present in many plants. CA acts as a free-radical scavenger by virtue of its hydrogen-donating ability, forming aryloxyl radicals and stabilization of such radicals by other functional groups in the structure, thus enhancing antioxidant activity [7, 8]. It has been reported that administration of CA protects the liver from nickel induced oxidative damage by decreasing the liver marker enzymes, lipid peroxidative markers and by increasing antioxidant cascade [8]. Moreover, beneficial effect of CA on the improvement of chromium induced oxidative stress in rat intestine has been documented [9].
2. Objectives
In this light, the aim of this study was to evaluate the effects of CA on the lead levels as well as some oxidative status related parameters in blood of mice following experimental lead poisoning.
3. Materials and Methods
3.1. Chemicals
In this experimental study, caffeic acid, lead acetate, 5, 5’-dithiobis-2-nitrobenzoic acid (DTNB), and 2-thiobarbituric acid (TBA) were purchased from Sigma Chemical Co. (St. Louis, MO, USA). Commercial enzyme kits for superoxide dismutase (Ransod, RANDOX/SD-125) and glutathione peroxidase (Ransel, RANDOX/RS-505) were achieved from Randox Laboratories (UK). The rest of the utilized chemicals were of analytical grade and were supplied by Sigma (St. Lewis, MO, USA) or Merck (Darmstadt, Germany).
Experimental design and sampling: Twenty four male albino mice weighing approximately 30 g were divided randomly into four groups of 6 each. Mice were housed in clean cages at room temperature (22 - 25°C) and a photoperiod of 12 hours light/12 hours dark per day. Animals received standard laboratory balanced commercial diet ad libitum. Group 1 mice received basal diet and tap water throughout the experiment and served as the control. Mice in group 2 received tap water containing 1000 ppm lead acetate. Mice in group 3 received CA (60 mg/kg body weight, daily) i.p. in isotonic saline during lead acetate treatment (1000 ppm in drinking water). Mice in group 4 only received CA (60 mg/kg body weight, daily) i.p. in isotonic saline throughout the experiment. The experiment was approved by Research ethics committee of the faculty of veterinary medicine of Ferdowsi University of Mashhad.
At the end of the experimental period (18 days), blood samples were taken from the heart under ether anesthesia in heparinized vials. The blood samples were centrifuged at 750 g for 20 minutes, and after plasma removal erythrocyte pellet was washed three times with normal saline solution. The washed centrifuged erythrocytes were lysed by adding the same volume of ice-cold redistilled water. Erythrocyte haemolysate were stored at -70°C until analysis.
Biochemical assays: For measurement of Pb levels, sample preparation was done as described previously [10, 11]. Pb concentrations in prepared whole blood samples were determined (in toxicology laboratory of Imam Reza hospital, Mashhad, Iran) by atomic absorption spectrophotometer (Perkin-Elmer 3030) at 283.3 nm wavelength by use of a graphite furnace. Limit of detection for this analysis was 5 ng/g and recovery for spiked samples was > 90%.
Determination of malondialdehyde (MDA) concentration in erythrocyte haemolysate was based on spectrophotometry of the pink colored product of thiobarbituric acid reactive substances, as described by Latha and Pari [12]. The concentration of MDA was calculated using a molar extinction coefficient value of 156,000 M-1 cm-1. Activities of glutathione peroxidase (GPx) and superoxide dismutase (SOD) in erythrocyte haemolysate were measured using Ransel and Ransod kits (Randox Company, UK), respectively, and the results were expressed as units per gram haemoglobin. Hemoglobin concentration was determined by cyanmethaemoglobin method.
Glutathione (GSH) concentration in erythrocyte haemolysate was assayed by the method previously described by Ellaman [13]. In this method, DTNB is reduced to 2-nitro-5’-mercaptobenzoic acid by GSH. The amount of the yellow colour produced was measured at 412 nm and expressed as micromoles per gram hemoglobin.
All experimental values have been represented as mean ± SEM. All results were analyzed using one way analysis of variance (ANOVA), followed by Bonferroni multiple comparisons test. The level of significance was set at P < 0.05. All calculations were performed using SPSS-13 software.
4. Results
Mortality was not observed in any experimental group throughout the experiment period. Blood Pb concentrations from mice in four treatment groups are compared in Figure 1. Pb concentration increased significantly in group 2 as compared to control unexposed group (P < 0.05). Blood Pb concentration was significantly lower in mice receiving Pb and CA (group 3) as compared to group 2, which received only Pb.
Values of measured oxidative stress-related biomarkers in erythrocyte haemolysate are presented in table 1. Lead acetate exposure caused a significant increase of MDA concentration in erythrocyte haemolysate as compared with control group. Mean erythrocytic SOD and GSH values were decreased in group 2 as compared to control group, although the decrease was only significant for GSH concentration. Administration of CA during Pb exposure increased the attenuated levels of erythrocytic GSH to the levels that were not significantly different from control group. However, CA had no significant effect in decreasing the augmented levels of erythrocytic MDA in group 3.
The Effect of Lead (Pb) and Caffeic Acid (CA) on Blood Lead Concentration (Mean ± SEM) in Mice (n = 6 in Each Group)
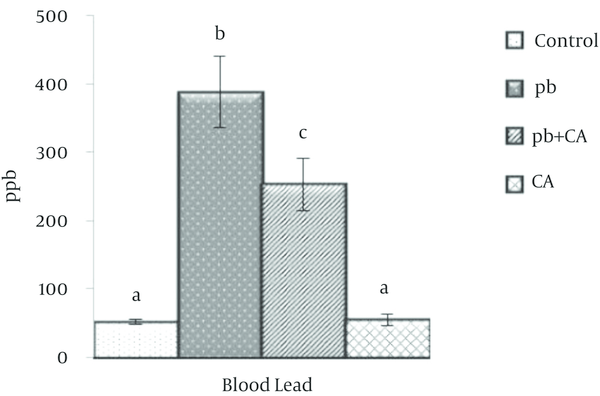
Effects of Lead (Pb) and Caffeic Acid (CA) on Some Oxidative Stress Markers in Blood of Mice
5. Discussion
In the present study, augmented values of lead concentration in Pb-exposed mice were reduced notably by CA administration. CA ability in forming complexes with some metal ions has been reported previously, the effect which is favored by the presence of two hydroxyl groups attached to its main ring that may produce a site for chelation [8, 9]. Up to authors’ knowledge, CA has not been used as a possible therapeutic approach for lead poisoning. However, it has been proposed that protective mechanism exerted by CA towards nickel toxicity may be due to its ability to chelate the nickel from liver by forming complexes with metal favoring its elimination [8].
Various natural nutrients including methionine, taurine, vitamins B1, B6, C, and E, selenium, copper, calcium, zinc, and alpha-lipoic acid have been shown, in animal studies, to stop or minimize the damaging effects of lead and improve the effects of pharmaceutical chelating agents [1, 14]. In line with the present study finding, some diverse biologically active compounds like allicin [11], methionine [15], thiamine [16], ascorbic acid [17], lipoic acid [18], and pyridoxine [19] has been shown to chelate lead and enhance its excretion from the body, resulting in decreased Pb accumulation in blood and tissues. Moreover, significant efficiency of Smilax glabra extract (contained 26.2% phenolics and 20.3% flavonoids) has been reported in reducing blood and tissue lead burden [20].
An investigation of the literature indicates that oxidative stress is an important contributor to the pathogenesis of lead poisoning, so a synergism might exist between antioxidants and chelating compounds [1]. It is widely accepted that pollutant-induced reactive oxygen species (ROS) generation can initiate or promote lipid peroxidation. Lead-induced enhancement of thiobarbituric acid reactive substances (TBARS) have been reported in some tissues of animals [21-25] that can be due to the generation of ROS without commensurate increases in the level of antioxidant defenses [26]. Results of the present work showed a significant increase of MDA concentrations in the erythrocyte haemolysate of Pb-exposed mice.
Determination of various endogenous antioxidants is applied as a marker of oxidative status. Depletion of tissue GSH is one of the primary factors that permit lipid peroxidation [27]. Results of the present work show significant decrease of GSH concentrations in the erythrocyte haemolysate of lead-exposed mice which is in line with previously published works [23, 28, 29]. Pb+2 binding to sulfhydryl groups can reduce GSH levels and can interfere with its antioxidant activity [18]. However, alterations of SOD and GPx activities were not significant in lead-exposed group in comparison to control group that is different to some extent from previously published results [29, 30].
Beneficial effects of some antioxidant compounds in reducing toxic effects of lead have been reported [30]. Pande et al. [22] demonstrated that lead-induced MDA production was decreased by 30% - 40% after supplementation with antioxidants. It has been also reported that both vitamins C and E are effective in decreasing lead-induced elevated values of TBARS by 60 and 30 percent, respectively [24]. Allicin was markedly effective in decreasing lead-induced lipid peroxidation and increasing the cellular antioxidant enzyme activities and GSH levels [31]. Despite known property of CA as an antioxidant [7, 8], its administration in the present work had no significant effect on increased values of MDA in erythrocyte haemolysate of mice in group 3 as compared to lead-exposed animals in group 2. Despite the present findings, Psotova et al. [32] reported that CA was able to decrease the augmented levels of lipid peroxidation and to eliminate intracellular GSH decline of hepatocytes intoxicated by Fe (III), Cu (II) and tert-butyl hydroperoxide. In addition, CA significantly reduced lipid peroxidation and restored the levels of antioxidant defense in the liver of rats intoxicated with nickel [8].
Like some other antioxidant compounds, phenolic acids, has been also reported to display a bimodal effect depending on their concentration range applied. The results of an in vitro study showed that some natural phenolics including caffeic, chlorogenic, ferulic, protocatechuic and rosmarinic acids behaved as antioxidants with chemoprotective effects in some low concentration ranges [32]. On the other hand, protocatechuic acid at concentrations above 2.5 mM showed in vitro pro-oxidation activity linked to the decrease in reduced glutathione and increase in cellular TBARS [33]. Nakamura et al. [34] described hepatotoxic and nephrotoxic effect of protocatechuic in mice at doses of 500 mg/kg, linked to decrease in GSH. The pro-oxidation effect of CA on Cu2+-induced LDL oxidation has been also described at its high concentrations [35].
The present study is the first investigation in which protective effects of caffeic acid against lead toxicity was evaluated. Some limitations of the present study including lack of sham group receiving intraperitoneal injection and the use of only one dose of caffeic acid should be considered when interpreting the results.
In summary, in the present work some beneficial effects of caffeic acid against Pb toxicity in mice were proved and this compound can be thus proposed as potential prophylactic treatment for amelioration of Pb toxicity. However, the results also indicate that applied dose of caffeic acid was unable to protect against lipid peroxidation induced by lead, despite its antioxidant properties. Although these results seem encouraging, more studies are required to elucidate the molecular basis of the curative properties of caffeic acid, its possible side effects, and its optimal dosage for therapeutic intervention of Pb poisoning.
Acknowledgements
References
-
1.
Patrick L. Lead toxicity part II: the role of free radical damage and the use of antioxidants in the pathology and treatment of lead toxicity. Altern Med Rev. 2006;11(2):114-27. [PubMed ID: 16813461].
-
2.
Gurer H, Ercal N. Can antioxidants be beneficial in the treatment of lead poisoning? Free Radic Biol Med. 2000;29(10):927-45. [PubMed ID: 11084283].
-
3.
Ahamed M, Siddiqui MK. Environmental lead toxicity and nutritional factors. Clin Nutr. 2007;26(4):400-8. [PubMed ID: 17499891]. https://doi.org/10.1016/j.clnu.2007.03.010.
-
4.
Ajayi GO, Adeniyi TT, Babayemi DO. Hepatoprotective and some haematological effects of Allium sativum and vitamin C in lead-exposed wistar rats. Int J Med Med Sci. 2009;1(3):64-7.
-
5.
Gurer H, Ozgunes H, Saygin E, Ercal N. Antioxidant effect of taurine against lead-induced oxidative stress. Arch Environ Contam Toxicol. 2001;41(4):397-402. [PubMed ID: 11598776]. https://doi.org/10.1007/s002440010265.
-
6.
Bokara KK, Brown E, McCormick R, Yallapragada PR, Rajanna S, Bettaiya R. Lead-induced increase in antioxidant enzymes and lipid peroxidation products in developing rat brain. Biometals. 2008;21(1):9-16. [PubMed ID: 18214713]. https://doi.org/10.1007/s10534-007-9088-5.
-
7.
Pari L, Karthikesan K. Protective role of caffeic acid against alcohol-induced biochemical changes in rats. Fundam Clin Pharmacol. 2007;21(4):355-61. [PubMed ID: 17635173]. https://doi.org/10.1111/j.1472-8206.2007.00505.x.
-
8.
Pari L, Prasath A. Efficacy of caffeic acid in preventing nickel induced oxidative damage in liver of rats. Chem Biol Interact. 2008;173(2):77-83. [PubMed ID: 18405891]. https://doi.org/10.1016/j.cbi.2008.02.010.
-
9.
Arivarasu NA, Priyamvada S, Mahmood R. Caffeic acid inhibits chromium(VI)-induced oxidative stress and changes in brush border membrane enzymes in rat intestine. Biol Trace Elem Res. 2012;148(2):209-15. [PubMed ID: 22328309]. https://doi.org/10.1007/s12011-012-9349-1.
-
10.
Shahsavani D, Baghshani H, Alishahi E. Efficacy of allicin in decreasing lead (Pb) accumulation in selected tissues of lead-exposed common carp (Cyprinus carpio). Biol Trace Elem Res. 2011;142(3):572-80. [PubMed ID: 20711682]. https://doi.org/10.1007/s12011-010-8801-3.
-
11.
Najar-Nezhad V, Aslani MR, Balali-Mood M. Evaluation of allicin for the treatment of experimentally induced subacute lead poisoning in sheep. Biol Trace Elem Res. 2008;126(1-3):141-7. [PubMed ID: 18719860]. https://doi.org/10.1007/s12011-008-8185-9.
-
12.
Latha M, Pari L. Preventive effects of Cassia auriculata L. flowers on brain lipid peroxidation in rats treated with streptozotocin. Mol Cell Biochem. 2003;243(1-2):23-8. [PubMed ID: 12619885].
-
13.
Ellaman GL. Tissue sulfhydryl group. Arch Biochem Biophys. 1959;82:70-2.
-
14.
Kalia K, Flora SJ. Strategies for safe and effective therapeutic measures for chronic arsenic and lead poisoning. J Occup Health. 2005;47(1):1-21. [PubMed ID: 15703449].
-
15.
Xie L, Gao Q, Xu H. Ameliorative effect of L-methionine on Pb-exposed mice. Biol Trace Elem Res. 2003;93(1-3):227-36. [PubMed ID: 12835504]. https://doi.org/10.1385/BTER:93:1-3:227.
-
16.
Coppock RW, Wagner WC, Reynolds JD, Vogel RS, Gelberg HB, Florence LZ, et al. Evaluation of edetate and thiamine for treatment of experimentally induced environmental lead poisoning in cattle. Am J Vet Res. 1991;52(11):1860-5. [PubMed ID: 1785729].
-
17.
Simon JA, Hudes ES. Relationship of ascorbic acid to blood lead levels. JAMA. 1999;281(24):2289-93. [PubMed ID: 10386552].
-
18.
Gurer H, Ozgunes H, Oztezcan S, Ercal N. Antioxidant role of alpha-lipoic acid in lead toxicity. Free Radic Biol Med. 1999;27(1-2):75-81. [PubMed ID: 10443922].
-
19.
Tandon SK, Flora SJ, Singh S. Influence of pyridoxine (vitamin B6) on lead intoxication in rats. Ind Health. 1987;25(2):93-6. [PubMed ID: 3624007].
-
20.
Xia D, Yu X, Liao S, Shao Q, Mou H, Ma W. Protective effect of Smilax glabra extract against lead-induced oxidative stress in rats. J Ethnopharmacol. 2010;130(2):414-20. [PubMed ID: 20580805]. https://doi.org/10.1016/j.jep.2010.05.025.
-
21.
Shafiq ur R, Rehman S, Chandra O, Abdulla M. Evaluation of malondialdehyde as an index of lead damage in rat brain homogenates. Biometals. 1995;8(4):275-9. [PubMed ID: 7580048].
-
22.
Pande M, Mehta A, Pant BP, Flora SJ. Combined administration of a chelating agent and an antioxidant in the prevention and treatment of acute lead intoxication in rats. Environ Toxicol Pharmacol. 2001;9(4):173-84. [PubMed ID: 11292581].
-
23.
Aykin-Burns N, Laegeler A, Kellogg G, Ercal N. Oxidative effects of lead in young and adult Fisher 344 rats. Arch Environ Contam Toxicol. 2003;44(3):417-20. [PubMed ID: 12712304]. https://doi.org/10.1007/s00244-002-2023-4.
-
24.
Flora SJ, Pande M, Mehta A. Beneficial effect of combined administration of some naturally occurring antioxidants (vitamins) and thiol chelators in the treatment of chronic lead intoxication. Chem Biol Interact. 2003;145(3):267-80. [PubMed ID: 12732454].
-
25.
Masso-Gonzalez EL, Antonio-Garcia MT. Natural antioxidants protect against lead-induced damage during pregnancy and lactation in rat's pups. Ecotoxicol Environ Saf. 2009;72(8):2137-42. [PubMed ID: 19439355]. https://doi.org/10.1016/j.ecoenv.2009.03.013.
-
26.
Adhami VM, Husain R, Agarwal AK, Seth PK. Intrahippocampal cholinergic-rich transplants restore lead-induced deficits: a preliminary study in rats. Neurotoxicol Teratol. 2000;22(1):41-53. [PubMed ID: 10642113].
-
27.
Konukoglu D, Serin O, Demiriz Kemerli G, Serin E, Hayirhoglu A, Oner B. A study on the carotid artery intima-media thickness and its association with lipid peroxidation. Clin Chim Acta. 1998;277(1):91-8. [PubMed ID: 9776048].
-
28.
Gong Z, Evans HL. Effect of chelation with meso-dimercaptosuccinic acid (DMSA) before and after the appearance of lead-induced neurotoxicity in the rat. Toxicol Appl Pharmacol. 1997;144(2):205-14. [PubMed ID: 9194404]. https://doi.org/10.1006/taap.1997.8118.
-
29.
Sharma V, Sharma A, Kansal L. The effect of oral administration of Allium sativum extracts on lead nitrate induced toxicity in male mice. Food Chem Toxicol. 2010;48(3):928-36. [PubMed ID: 20060875]. https://doi.org/10.1016/j.fct.2010.01.002.
-
30.
Hsu PC, Liu MY, Hsu CC, Chen LY, Guo YL. Lead exposure causes generation of reactive oxygen species and functional impairment in rat sperm. Toxicology. 1997;122(1-2):133-43. [PubMed ID: 9274809].
-
31.
Shahsavani D, Baghshani H, Aslani MR, Fatemi FS. The impact of allicin on lead-induced oxidative damage in selected organs of the common carp (Cyprinus carpio). Comparat Clin Pathol. 2012;21(5):769-75.
-
32.
Psotova J, Lasovsky J, Vicar J. Metal-chelating properties, electrochemical behavior, scavenging and cytoprotective activities of six natural phenolics. Biomed Pap Med Fac Univ Palacky Olomouc Czech Repub. 2003;147(2):147-53. [PubMed ID: 15037895].
-
33.
Babich H, Sedletcaia A, Kenigsberg B. In vitro cytotoxicity of protocatechuic acid to cultured human cells from oral tissue: involvement in oxidative stress. Pharmacol Toxicol. 2002;91(5):245-53. [PubMed ID: 12570031].
-
34.
Nakamura Y, Torikai K, Ohigashi H. Toxic dose of a simple phenolic antioxidant, protocatechuic acid, attenuates the glutathione level in ICR mouse liver and kidney. J Agric Food Chem. 2001;49(11):5674-8. [PubMed ID: 11714376].
-
35.
Yamanaka N, Oda O, Nagao S. Prooxidant activity of caffeic acid, dietary non-flavonoid phenolic acid, on Cu2+-induced low density lipoprotein oxidation. FEBS Lett. 1997;405(2):186-90. [PubMed ID: 9089288].