Abstract
Background:
Exercise training and crocin, as a herbal antioxidant, help improve metabolism and insulin sensitivity in type 2 diabetes mellitus (T2D). AMP-activated protein kinase (AMPK) and nuclear respiratory factor 1 (NRF1) involve in the mitochondrial biogenesis pathway and diabetes control.Objective:
This study aimed to investigate the effects of high-intensity interval training (HIIT), and low intensity continued training (LICT) in combination with crocin consumption on AMPK and NRF1 gene expression in the muscle tissue of diabetic rats.Methods:
In this experimental study, 56 adult rats were divided into eight groups (n = 7), including diabetic (crocin utilization, HIIT, LICT, HIIT with crocin, LICT with crocin, control, sham groups) and healthy control group. The HIIT and LICT groups performed five sessions of training per week for eight weeks, and the crocin consumption groups received 25 mg/kg of crocin daily for eight weeks. Moreover, AMPK and NRF1 genes expression levels were evaluated in different groups using real-time polymerase chain reaction (RT-PCR).Results:
According to our results, while induction of diabetes significantly decreased the levels of NRF1 and AMPK gene expression (P ≤ 0.05), training and crocin consumption significantly increased the levels of AMPK and NRF1 gene expression (P ≤ 0.05).Conclusions:
It appears that crocin consumption along with HIIT improves diabetes symptoms through AMPK and NRF1 mechanism in diabetic rats.Keywords
1. Background
Insulin resistance, elevated hepatic glucose output, and β-cell dysfunction are the factors that describe type 2 diabetes (T2D) (1). Diabetic patients can be characterized by weakened protein, lipid, and carbohydrate metabolism and high levels of fasting blood glucose (2). Muscles, eyes, blood vessels, heart, and kidneys are the important organs affected in diabetes-induced chronic hyperglycemia. Hence, muscular atrophy, retinopathy, cardiac dysfunction, atherosclerosis, and nephropathy are T2D-induced complications. To improve T2D, insulin sensitivity and glucose homeostasis are considered as effective treatments. AMP-activated protein kinase (AMPK) is a sensor of energy that diminishes hepatic glucose production, stimulates fatty acid oxidation in the adipose, and induces glucose uptake in the skeletal muscles. The activation of AMPK causes insulin sensitivity, and therefore AMPK is an attractive therapeutic target for T2D (1). Another potential AMPK target is nuclear respiratory factor 1 (NRF1), which is needed for the coordinated expression of a multitude of nuclear- and mitochondrial-encoded enzymes found within the mitochondrial respiratory chain. AMPK increases the expression of NRF1. Today, recent studies focus on anti-diabetic natural antioxidants, which improve oxidative stress and inflammation in obesity and T2D. Crocin is a natural carotenoid found in saffron, which is responsible for the color of saffron and can induce its metabolic effects through the increase of antioxidants (3). Research shows that crocin has neuro-protective, anti-platelet, anti-hyperlipidemic, anti-carcinogenic, and antioxidant properties (4). Previous studies reported the anti-oxidative and hypoglycemic properties of crocin in diabetic rats (5). Accordingly, it was shown that in diabetic rats, blood glucose level significantly reduced by the administration of 60 mg/kg of crocin in the kidneys and liver. Also, administration of 50 and 100 mg/kg of crocin significantly decreased serum glucose, triglyceride, total cholesterol, advanced glycation end products (AGEs), and low-density lipoprotein (LDL) levels in diabetic rats while increasing high-density lipoprotein (HDL) (6). It is reported that both high-intensity interval training (HIIT) and low-intensity continued training (LICT) can improve lipids and blood glucose metabolism. Accordingly, physical activity along with exercise modifications can be used in T2D treatment (7). Furthermore, exercise can increase insulin sensitivity and FOXO1 expression and reduce blood lipid profiles and glycated hemoglobin (HbA1c) (7). The results of various studies have shown that exercise in interaction with the use of antioxidants can have positive effects on the improvement of diabetes and its impairments. In this vein, it has been shown that four weeks of consuming coriander extract with endurance training had interactive hypoglycemic effects in diabetic rats (8). Also, simultanous consumption of saffron with resistance training significantly improved the glycemic indices of diabetic rats (9).
2. Objectives
Although studies provide a good amount of information on the biological properties of crocin, there is limited in vivo research about its potential anti-diabetic properties alone or in combination with training. Therefore, the main purpose of this research was to survey the effect of crocin consumption combined with endurance training on the levels of AMPK and NRF1 gene expression in diabetic rats.
3. Methods
3.1. Animals and Animal Treatment
In this empirical study, 56 adult male pedigree Sprague-Dawley rats with a weight of 155 ± 30 g were purchased from the animal proliferation and reproduction center of Islamic Azad University (IAU) in Marvdasht, Iran. Following transfer to the animal physiological lab, the rats were maintained under standard conditions (humidity of 50%, 12-hour light and dark cycle, the temperature of 22 to 27°C, and free access to water and food) and passed the seven-day adaptation period. Streptozotocin (STZ) (Aldrich Sigma) in combination with a high-fat diet was applied to induce T2D. To this end, all rats were subjected to eight weeks of a high-fat diet of 45% whole animal fat, with every 100 grams containing 41 grams of carbohydrate, 24 grams of protein, and 24 grams of fat (10). After eight weeks, 50 mg/kg of STZ was administered intraperitoneally (10), and 96 hours after the administration with a small injury in the animals' tails, a drop of blood was placed on a glucometer tape and read by a glucometer. After measuring blood glucose levels in rats, those with blood glucose levels higher than 300 mg/dL were chosen as the study samples. Afterward, the rats were divided into eight groups (n = 7), including diabetic (crocin utilization, HIIT, LICT, HIIT with crocin, LICT with crocin, control, sham groups) and healthy control group. To inquire the diabetes induction of effects on the research variables, healthy control group was selected. The rats in HIIT and HIIT with crocin groups were trained in a period of eight weeks, five training sessions per week at the severity of 80 to 85% of the maximum speed for two minutes, and active rest alternations of one minute. HIIT reached from five sessions in the first week to 12 sessions in the last week. The rats in LICT and LICT with crocin groups were also trained for eight weeks, five sessions per week at an intensity of 50 to 55% of maximum running speed. LICT started in the first week at 25 minutes and reached 50 minutes in the last week. In addition, the whole size of training activities (severity, time, and repetition) was coordinated between the two groups of LICT and HIIT (11). Also, crocin utilization groups received 25 mg/kg of crocin (Sigma Company, USA) daily for eight weeks. Crocin was administered intraperitoneally and dissolved in normal saline (11). To control the effects of crocin injection on the variables of the study, the sham group received crocin solvent intraperitoneally each day. Then, 48 hours after the final training session, rats were sacrificed to measure the variables. After about five minutes, rats were anesthetized with xylazine 2% at a dose of 10 mg/kg and ketamine 10% at a dose of 50 mg/kg, and the soleus muscle was extracted by specialists, and the tissue was kept in the freezer at −80°C for RNA purification.
3.2. RNA Extraction and RT-PCR Analysis
After extraction of DNA, the levels of AMPK and NRF1 gene expression were measured by the real-time polymerase chain reaction (RT-PCR) method. The sequence of primers used is shown in Table 1.
The Sequence Primers of the Genes Required for Real-Time PCR
Gene | Forward (5' - 3') | Reverse (5' - 3') | Product Size (bp) |
---|---|---|---|
B2m | CGTGCTTGCCATTCAGAAA | ATATACATCGGTCTCGGTGG | 244 |
AMPK | GCACCTTCGGCAAAGTGAAG | CTCTTCAACCCTCCCGTGTT | 266 |
NRF1 | TGGCTGAAGCCACCTTACAA | ATGAACTCCATCTGGGCCATT | 113 |
3.3. Statistical Analysis
The Shapiro-Wilk test was used to determine the findings of normal distribution. Also, for analysis of the findings, one-way ANOVA and two-way ANOVA with Tukey's post-hoc tests were used. Furthermore, P < 0.05 was considered as a significant level.
4. Results
The relative levels of AMPK and NRF1 gene expression (2 – ΔΔCT) in eight research groups are reported in Figures 1 and 2, respectively. The results of the independent sample t-test showed that the levels of AMPK (P < 0.001) and NRF1 (P < 0.001) gene expression in the diabetic control group significantly decreased compared to the healthy control group. Therefore, the induction of diabetes with STZ and a high-fat diet significantly decreased the levels of AMPK and NRF1 gene expression in rats. The one-way ANOVA results revealed significant differences in the levels of AMPK and NRF1 gene expression in the research groups. The results of Tukey's post hoc test showed no significant differences in the levels of AMPK (P = 0.96) and Nrf1 (P = 0.78) gene expression in the diabetic control group and sham group. Therefore, it is estimated that crocin solvent has no significant effect on the gene expression levels of AMPK and NRF1 in diabetic rats. However, in the crocin consumption group, the levels of NRF1 (P < 0.001) and AMPK (P < 0.001) gene expression were higher than those in the diabetic control group. In the HIIT group, the levels of NRF1 (P = 0.002) and AMPK (P = 0.012) gene expression were significantly increased compared to the diabetic control group. In the HIIT with crocin consumption group, the level of NRF1 was significantly higher than the crocin consumption group (P = 0.001). Moreover, in the LICT with crocin consumption group, the APMK was significantly lower than the crocin consumption group (P = 0.01), and in the LICT group, the level of NRF1 was significantly higher than the diabetic control group (P = 0.02) (Figures 1 and 2).
The amount of AMPK expression in the soleus muscles of rats in all research groups. Data are reported as mean ± SEM. Comparisons between different groups are shown in the figure. * P-value < 0.05; **, P-value < 0.01.
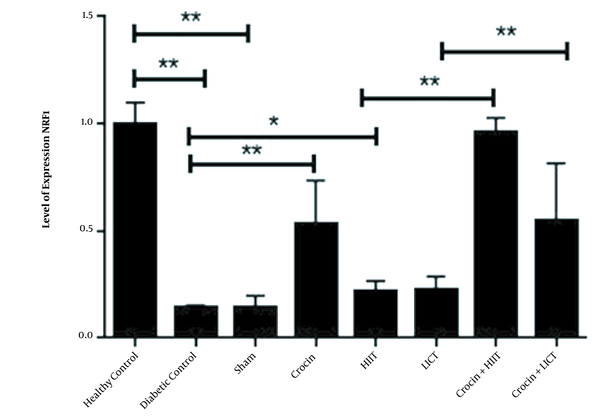
The amount of NRF1 expression in the soleus muscles of rats in all research groups. Data are reported as mean ± SEM. Comparisons between different groups are shown in the figure. * P-value < 0.05; **, P-value < 0.01.
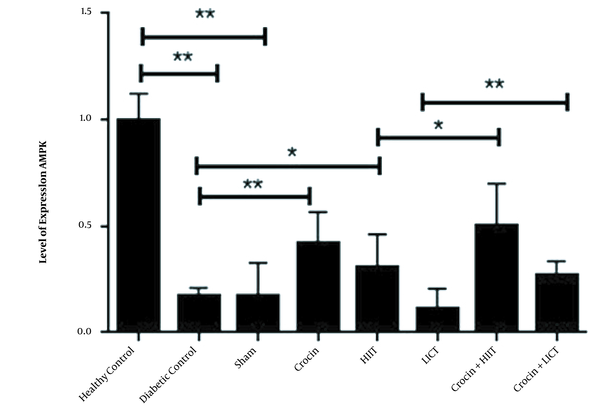
5. Discussion
The present study showed that the induction of diabetes with STZ (50 mg/kg) and a high-fat diet significantly reduced the levels of AMPK and NRF1 gene expression. STZ-induced diabetes is a well-known model that mimics diabetic conditions and is useful for evaluating diabetes complications (12). It is suggested that the diabetic consequence of STZ leads to toxicity in pancreatic cells by excessive production of reactive oxygen species (ROS), which consequently reduces the release and synthesis of insulin (13). For example, Dehghani et al. reported that the injection of 55 mg/kg of STZ reduced the release and production of insulin and induced oxidative stress and apoptosis (2).
In the present investigation, consuming 25 mg/kg of crocin increased the levels of AMPK and NRF1 gene expression. The antioxidant effects of AMPK seem to depend on the abundance of nutrients and the antioxidant capacity of cells. Studies show that foods high in antioxidants, especially those high in polyphenols and fiber, activate AMPK. Vegetables and fruits are rich in polyphenols and fiber. Polyphenols are chemicals that color fruits and vegetables and can help activate AMPK (14). Also, in one study, it was shown that the optimal level of arginine in the diet (1.62 - 1.96%) can improve the antioxidant capacity by the AMPK signaling pathway (15).
The ability of crocin to increase the NRF1 and AMPK could be attributed to the improvement of mitochondrial biogenesis in the skeletal muscle, as well as the antioxidant and insulin sensitivity properties of crocin (16). Studies of the hypoglycemic effects of crocin have shown that this combination reduces blood sugar, lowers advanced glycation end products, decreases insulin resistance, and is also useful in the treatment of diabetic neuropathy (11). It is possible that crocin, with its strong antioxidant capacity, can be useful in the treatment of T2D by improving mitochondrial function and reducing ROS and oxidative stress (4). Antioxidant effects of crocin, which have been previously examined in other models, might confirm this hypothesis (5). For instance, Naghizadeh et al. reported that crocin by a reduction in malondialdehyde (MDA) and increase of glutathione peroxidase decreased cisplatin-induced renal oxidative stress (17). Moreover, Chen et al. reported that consumption of 18.7 and 37.5 mg/kg of crocin for six weeks raised glutathione peroxidase in the liver, superoxide dismutase levels in the kidneys and liver, and total antioxidant capacity in the kidneys in rats (18). Also, Altınöz et al. demonstrated that consumption of crocin at the dose of 20 mg/kg for 21 days could modulate mRNA expression of antioxidant enzymes (CAT, GPX, and SOD). Further consumption of crocin can treat the STZ-induced oxidative stress in the brain of diabetic rats (19). The radical scavenging activity of crocin has also been shown in several in vitro models (20). Generally, it appears that crocin, by increasing the rate of antioxidant enzymes and radical scavenging activity applies its antioxidant activities in the tissues (5).
The results of the present research revealed that HIIT significantly increased the levels of AMPK and NRF1 gene expression in diabetic rats. Previous studies have reported that sports activities reduce the risk of T2D and cardiovascular disease (1). Antioxidant supplements and exercise can increase the AMP/ATP ratio and consequently activate AMPK (21). Also, studies have indicated that muscle contractions in humans (22) as well as rodents (23) increase the AMPK; thus, exercise conveys many of its positive effects. Exercise increases the ATP turnover by approximately 100-fold in the skeletal muscle, leading to a rapid increase in the ADP and AMP levels in a severity-dependent manner (24). In the present study, HIIT had a substantial effect on the increased values of AMPK and NRF1 gene expression in diabetic rats. One hour cycling on a bicycle with 75% of VO2max induced AMPK activation (23); also, cycling up to exhaustion with 45% of VO2max induced Thr172-AMPKα2 phosphorylation (25).
The positive effects of exercise are linked to weight loss, increased muscle Glut4, increased blood flow, decreased hepatic glucose production, and normalized blood lipids. Long-term exercise improves glucose tolerance and insulin sensitivity and increases glycogen storage by increasing skeletal muscle mass (26). Research has clearly shown that endurance athletes and active individuals have a higher mitochondrial content, which results in better oxidation capacity, proper body composition, and resistance to various diseases, including obesity and diabetes (27).
It was shown that LICT had no significant effect on AMPK level in diabetic rats. Efficient compatibility to the training needs optimum exposure to pulses of reactive oxygen and nitrogen species (RONS). An inadequate exercising stimulus may induce oxidative deactivation of AMPK, decreased adaptation, and extreme RONS formation (28). In this regard, the findings showed that AMPK activation decreased in response to 120 minutes of cycling (29). A decreased free radical release and adrenergic response to prolonged exercise could explicate the decreased AMPK activation (28).
Regarding the interactive effects in the present study, HIIT, along with crocin consumption compared to crocin consumption, significantly increased the levels of NRF1 gene expression. In the present investigation, changes in the levels of AMPK and NRF1 gene expression in the muscle tissue confirms the antioxidant properties and potential role of crocin in defense against free radicals. Therefore, HIIT, along with concurrent consumption of crocin, could be considered as a beneficial therapy for diabetic patients. In conclusion, based on the results of this study, it appears that crocin probably holds protective effects on diabetes, and it can be used in the treatment of diabetic complications. Furthermore, it seems that interval training together with the consumption of crocin probably have the potential to improve diabetes symptoms through AMPK and NRF1 mechanism in diabetic rats.
References
-
1.
Coughlan KA, Valentine RJ, Ruderman NB, Saha AK. AMPK activation: a therapeutic target for type 2 diabetes? Diabetes Metab Syndr Obes. 2014;7:241-53. [PubMed ID: 25018645]. [PubMed Central ID: PMC4075959]. https://doi.org/10.2147/DMSO.S43731.
-
2.
Dehghan F, Hajiaghaalipour F, Yusof A, Muniandy S, Hosseini SA, Heydari S, et al. Saffron with resistance exercise improves diabetic parameters through the GLUT4/AMPK pathway in-vitro and in-vivo. Sci Rep. 2016;6:25139. [PubMed ID: 27122001]. [PubMed Central ID: PMC4848502]. https://doi.org/10.1038/srep25139.
-
3.
Sanchez AM, Carmona M, Jaren-Galan M, Mosquera MI, Alonso GL. Picrocrocin kinetics in aqueous saffron spice extracts (Crocus sativus L.) upon thermal treatment. J Agric Food Chem. 2011;59(1):249-55. [PubMed ID: 21141822]. https://doi.org/10.1021/jf102828v.
-
4.
Hazman O, Aksoy L, Buyukben A. Effects of crocin on experimental obesity and type-2 diabetes. Turk J Med Sci. 2016;46(5):1593-602. [PubMed ID: 27966333]. https://doi.org/10.3906/sag-1506-108.
-
5.
Rajaei Z, Hadjzadeh MA, Nemati H, Hosseini M, Ahmadi M, Shafiee S. Antihyperglycemic and antioxidant activity of crocin in streptozotocin-induced diabetic rats. J Med Food. 2013;16(3):206-10. [PubMed ID: 23437790]. https://doi.org/10.1089/jmf.2012.2407.
-
6.
Shirali S, Zahra Bathaie S, Nakhjavani M. Effect of crocin on the insulin resistance and lipid profile of streptozotocin-induced diabetic rats. Phytother Res. 2013;27(7):1042-7. [PubMed ID: 22948795]. https://doi.org/10.1002/ptr.4836.
-
7.
Zanuso S, Jimenez A, Pugliese G, Corigliano G, Balducci S. Exercise for the management of type 2 diabetes: a review of the evidence. Acta Diabetol. 2010;47(1):15-22. [PubMed ID: 19495557]. https://doi.org/10.1007/s00592-009-0126-3.
-
8.
Hosseini SA, Zar AS, Ghasemi A, Khoradmehr O, Farkhaie F. [hypoglycemic interactional effects of coriandrum sativum extract and endurance training in diabetic rats]. Iranian J Nutr Sci Food Technol. 2018;13(2):21-30. Persian.
-
9.
Hosseini SA, Nik bakht H, Azarbayjani MA. [The effect of aqua extract of saffron with resistance training on glycemic indexes of streptozotocin induced diabetic rats]. Armaghane Danesh. 2013;18(4):284-94. Persian.
-
10.
Khalafi M, Shabkhiz F, Azali Alamdari K, Bakhtiyari A. [Irisin response to two types of exercise training in type 2 diabetic male rats]. J Arak Uni Med Sci. 2016;19(6):37-45. Persian.
-
11.
Peyravi A, Yazdanpanahi N, Nayeri H, Hosseini SA. The effect of endurance training with crocin consumption on the levels of MFN2 and DRP1 gene expression and glucose and insulin indices in the muscle tissue of diabetic rats. J Food Biochem. 2020;44(2). e13125. [PubMed ID: 31849103]. https://doi.org/10.1111/jfbc.13125.
-
12.
Arshadi S, Azarbayjani MA, Hajaghaalipor F, Yusof A, Peeri M, Bakhtiyari S, et al. Evaluation of Trigonella foenum-graecum extract in combination with swimming exercise compared to glibenclamide consumption on type 2 Diabetic rodents. Food Nutr Res. 2015;59:29717. [PubMed ID: 26699937]. [PubMed Central ID: PMC4689800]. https://doi.org/10.3402/fnr.v59.29717.
-
13.
Szkudelski T. The mechanism of alloxan and streptozotocin action in B cells of the rat pancreas. Physiol Res. 2001;50(6):537-46. [PubMed ID: 11829314].
-
14.
Jansen T, Kvandova M, Daiber A, Stamm P, Frenis K, Schulz E, et al. The AMP-activated protein kinase plays a role in antioxidant defense and regulation of vascular inflammation. Antioxidants (Basel). 2020;9(6):525. [PubMed ID: 32560060]. [PubMed Central ID: PMC7346208]. https://doi.org/10.3390/antiox9060525.
-
15.
Liang H, Ji K, Ge X, Ren M, Liu B, Xi B, et al. Effects of dietary arginine on antioxidant status and immunity involved in AMPK-NO signaling pathway in juvenile blunt snout bream. Fish Shellfish Immunol. 2018;78:69-78. [PubMed ID: 29678792]. https://doi.org/10.1016/j.fsi.2018.04.028.
-
16.
Kapucu A. Crocin ameliorates oxidative stress and suppresses renal damage in streptozotocin induced diabetic male rats. Biotech Histochem. 2021;96(2):153-60. [PubMed ID: 32835521]. https://doi.org/10.1080/10520295.2020.1808702.
-
17.
Boroushaki MT, Naghizadeh B, Mofidpoor H. Protective effects of crocin against cisplatin-induced acute renal failure and oxidative stress in rats. Toxicology Letters. 2008;(180). S230. [PubMed ID: 18506215].
-
18.
Chen Y, Yang T, Huang J, Tian X, Zhao C, Cai L, et al. Comparative evaluation of the antioxidant capacity of crocetin and crocin in vivo. Chinese Pharmacol Bull. 2010;26(2):248-51.
-
19.
Altınöz E, Ekici C, Özyazgan B, Çiğremiş Y. The effects of crocin (active contstituent of saffron) treatment on brain antioxidant enzyme mRNA levels in diabetic rats/Diyabetik ratlarda safranın aktif içeriği olan krosin tedavisinin beyindeki antioksidan enzimlerin mRNA seviyeleri üzerine etkisi. Turkish J Biochem. 2016;41(2). https://doi.org/10.1515/tjb-2016-0018.
-
20.
Mousavi SH, Tayarani NZ, Parsaee H. Protective effect of saffron extract and crocin on reactive oxygen species-mediated high glucose-induced toxicity in PC12 cells. Cell Mol Neurobiol. 2010;30(2):185-91. [PubMed ID: 19711182]. https://doi.org/10.1007/s10571-009-9441-z.
-
21.
Richter EA, Ruderman NB. AMPK and the biochemistry of exercise: implications for human health and disease. Biochem J. 2009;418(2):261-75. [PubMed ID: 19196246]. [PubMed Central ID: PMC2779044]. https://doi.org/10.1042/BJ20082055.
-
22.
Park H, Kaushik VK, Constant S, Prentki M, Przybytkowski E, Ruderman NB, et al. Coordinate regulation of malonyl-CoA decarboxylase, sn-glycerol-3-phosphate acyltransferase, and acetyl-CoA carboxylase by AMP-activated protein kinase in rat tissues in response to exercise. J Biol Chem. 2002;277(36):32571-7. [PubMed ID: 12065578]. https://doi.org/10.1074/jbc.M201692200.
-
23.
Wojtaszewski JF, Nielsen P, Hansen BF, Richter EA, Kiens B. Isoform-specific and exercise intensity-dependent activation of 5'-AMP-activated protein kinase in human skeletal muscle. J Physiol. 2000;528 Pt 1:221-6. [PubMed ID: 11018120]. [PubMed Central ID: PMC2270117]. https://doi.org/10.1111/j.1469-7793.2000.t01-1-00221.x.
-
24.
Sahlin K, Tonkonogi M, Soderlund K. Energy supply and muscle fatigue in humans. Acta Physiol Scand. 1998;162(3):261-6. [PubMed ID: 9578371]. https://doi.org/10.1046/j.1365-201X.1998.0298f.x.
-
25.
Wojtaszewski JF, Mourtzakis M, Hillig T, Saltin B, Pilegaard H. Dissociation of AMPK activity and ACCbeta phosphorylation in human muscle during prolonged exercise. Biochem Biophys Res Commun. 2002;298(3):309-16. [PubMed ID: 12413941]. https://doi.org/10.1016/s0006-291x(02)02465-8.
-
26.
Yang D, Yang Y, Li Y, Han R. Physical exercise as therapy for type 2 diabetes mellitus: From mechanism to orientation. Ann Nutr Metab. 2019;74(4):313-21. [PubMed ID: 31013502]. https://doi.org/10.1159/000500110.
-
27.
Steib K, Schaffner I, Jagasia R, Ebert B, Lie DC. Mitochondria modify exercise-induced development of stem cell-derived neurons in the adult brain. J Neurosci. 2014;34(19):6624-33. [PubMed ID: 24806687]. [PubMed Central ID: PMC6608139]. https://doi.org/10.1523/JNEUROSCI.4972-13.2014.
-
28.
Morales-Alamo D, Calbet JAL. AMPK signaling in skeletal muscle during exercise: Role of reactive oxygen and nitrogen species. Free Radic Biol Med. 2016;98:68-77. [PubMed ID: 26804254]. https://doi.org/10.1016/j.freeradbiomed.2016.01.012.
-
29.
McConell GK, Lee-Young RS, Chen ZP, Stepto NK, Huynh NN, Stephens TJ, et al. Short-term exercise training in humans reduces AMPK signalling during prolonged exercise independent of muscle glycogen. J Physiol. 2005;568(Pt 2):665-76. [PubMed ID: 16051629]. [PubMed Central ID: PMC1474728]. https://doi.org/10.1113/jphysiol.2005.089839.