Abstract
Background:
Dysbiosis and metabolic disorders of the microbiota, often caused by an imbalance in the intestinal microbial composition, are significant issues linked to immobility, obesity, and diabetes. Physical exercise is recognized for its role in managing these symptoms by regulating the composition and metabolites of the intestinal microbiota, thereby improving gut health and overall metabolic function.Objectives:
This study aimed to investigate and compare the effects of continuous endurance training (CET) and high-intensity interval training (HIIT) on two key cecal microbiota metabolites, butyrate and propionate, in diabetic rats.Methods:
Forty-five male Wistar rats were made diabetic by a high-fat diet and were trained under CET and HIIT exercise protocols. Cecal tissue samples were taken from the rats to evaluate the effect of exercise, and the levels of two microbial metabolites, butyrate and propionate, were measured using the high-performance liquid chromatography (HPLC) method.Results:
Among the exercise patterns studied, HIIT significantly improved the concentrations of butyrate and propionate, while CET showed no effect on these metabolites.Conclusions:
Our findings suggest that, unlike CET, HIIT may effectively mitigate metabolic disturbances resulting from gut dysbiosis in diabetic patients. However, any definitive conclusion about the effects of CET and HIIT exercises on intestinal microbial metabolites necessitates further comprehensive tests on other metabolites and an examination of additional supporting evidence, such as changes in the composition of the intestinal microbiome.Keywords
Microbiota Metabolites Short Chain Fatty Acids Diabetes Exercise
1. Background
Alterations in intestinal microbiota composition, termed dysbiosis, lead to fluctuations in metabolite production through fermentation processes (1, 2). Short-chain fatty acids (SCFAs), such as acetate, butyrate, and propionate, are vital products of this fermentation, profoundly impacting glucose and insulin regulation (3-5). For instance, butyric acid exhibits local anti-inflammatory and immune-regulating properties, alongside its role as an energy substrate and regulator of gut hormones (6). Short-chain fatty acids have been implicated in obesity prevention, insulin resistance mitigation, and induction of thermogenesis in brown fat (5, 7-9). Dysbiosis can lead to significant changes in gut microbial diversity, increased monosaccharide absorption, elevated levels of lipogenic enzymes, and the development of insulin resistance and obesity. In obese individuals, enzymes in the glucose signaling pathways with insulin are diminished, contributing to chronic low-grade inflammation. This inflammation is marked by higher levels of pro-inflammatory cytokines and free fatty acids (FFA), which can interfere with the normal function and secretion of insulin, inducing insulin resistance and beta-cell dysfunction (10, 11).
Studies have shown that changes in the gut microbiota lead to alterations in the production of SCFAs and enzymes that directly influence glucose and insulin regulation, ultimately contributing to obesity (12). Short-chain fatty acids, such as propionate and acetate, are transported through the bloodstream to various organs, where they serve as substrates for energy metabolism, particularly by liver cells, which use propionate for gluconeogenesis (13). Short-chain fatty acids also stimulate the secretion of endocrine hormones that regulate energy balance and glucose homeostasis (4). Therefore, dysbiosis, through its impact on SCFA production and glucose and insulin-regulating enzymes, is expected to contribute to the development of obesity and type 2 diabetes.
The gut microbiota and mucosal integrity are subject to various influences, including diet, environment, and notably, physical activity (14). Conversely, obesity and inactivity exhibit a strong correlation, directly contributing to insulin resistance and augmenting the risk of metabolic syndrome (15, 16). Given the potential link between obesity and gut microbiome alterations, understanding the factors influencing intestinal microbial changes and their correction becomes imperative in combating obesity and preventing metabolic syndrome.
Regular physical activity has been shown to modulate the intestinal microbiota (17), prompting the need to investigate how different exercise interventions, varying in intensity and duration, influence microbial content and metabolites, alongside their role in diabetes control and inflammation mitigation.
Several mechanisms can explain how exercise impacts the gut microbiome. Research has shown that exercise reduces the abundance of bacteria producing branched-chain amino acids (BCAA) (18), which are mediators in the development of insulin resistance. Evidence suggests that exercise-induced changes in the gut microbiota are associated with improved glucose homeostasis and insulin sensitivity (19). The gut-associated lymphoid tissue (GALT) houses most of our immune cells, and exercise can modulate their gene expression, favoring anti-inflammatory and antioxidant responses, which may mediate host-microbial interactions (20). Exercise can also modify bile acid circulation, a known regulator of microbial community structure. Additionally, the metabolic demands of exercise release compounds like myokines and lactate (21), which might directly or indirectly interact with the gut environment, potentially influencing the microbiome. However, due to the lack of control over diet, age, and type of exercise in these studies, the mechanisms and extent of exercise's impact on the gut microbiota remain inconclusive, leading to conflicting results. Therefore, understanding the effects of exercise on gut microbiota composition and structure is still in its early stages, and the role of microbiota in exercise adaptation remains unknown.
2. Objectives
This study aims to investigate the impact of two major exercise patterns, continuous endurance training (CET) and high-intensity interval training (HIIT), on cecal butyrate and propionate metabolites. Utilizing the high-performance liquid chromatography (HPLC) method, we seek to elucidate the differential effects of these exercise modalities on intestinal microbial metabolites, shedding light on their potential in diabetes management and inflammation control.
3. Methods
3.1. Study Subjects and Sample Collection
Forty-five male Wistar rats, aged 8 weeks and weighing 200 ± 10 g, were obtained from the Razi Institute, Iran. After a week of acclimatization, the rats were divided into control and high-calorie diet groups. Diabetes was confirmed after 16 weeks based on fasting blood sugar levels (> 250 mg/dL) and HOMA-IR (> 3) (Table 1). The rats were then randomly assigned to four groups: Non-diabetic control (NDC), diabetic control (DC), CET, and HIIT. Thirteen rats were excluded due to leg injuries or not meeting the diabetes threshold. After 24 hours of the final exercise session, the rats were euthanized with ketamine (90 mg/kg) and xylazine (10 mg/kg), and blood and cecal tissue samples were collected for analysis and stored at -80°C.
Serum Glucose Levels in Rats: Blood Sugar Analysis
3.2. Diet and Exercise Protocol
The high-calorie diet used in this study was procured from the Razi Institute and consisted of 30% animal fat, 25% fructose, and 45% basic animal food composition. Rats underwent familiarization with the exercise regimen one week post-induction of diabetes, with five weekly training sessions. Prior to commencing the formal training, rats were acclimated to the exercise protocol at a reduced speed compared to the designated intensity. The main training phase then spanned nine weeks.
The exercise regimen was structured as follows: The DC and NDC groups did not engage in any formal exercise program. However, to maintain consistent conditions among all groups, they were placed on a stationary treadmill for 10 to 15 minutes, five times a week. The CET and HIIT groups followed the protocol outlined in Table 2 for a duration of ten weeks. The general steps of the experiment are illustrated in Figure 1.
Continuous Endurance Training and High-intensity Interval Training Exercise Protocol (22, 23)
Practice Procedures | Warm up | Context of Exercise Plan | Cooling Down |
---|---|---|---|
Practice time (min) | 5 min | 30 min (CET) → 1 min (4 HIIT repeats) and 3 min (HIIT recovery) | 5 min |
Intensity of practice (% of VO2 max) | 30 - 40% | 50 - 60% (CET) → 30 - 35% (4 HIIT repeats) and 85 - 90% (HIIT recovery) | 30 - 40% |
Decline | 0 | 0 | 0 |
Schematic representation of the experiment phases: A, induction of diabetes through a high-fat diet; B, confirmation of diabetes via a blood sugar test; C, implementation of exercise interventions using HIIT and CET protocols; D, collection of cecal samples; and E, analysis of the intervention's effect on cecal microbiota metabolites using HPLC. Abbreviations: NDC, non-diabetic control; DC, diabetic control; CET, continuous endurance training; HIIT, high-intensity interval training.
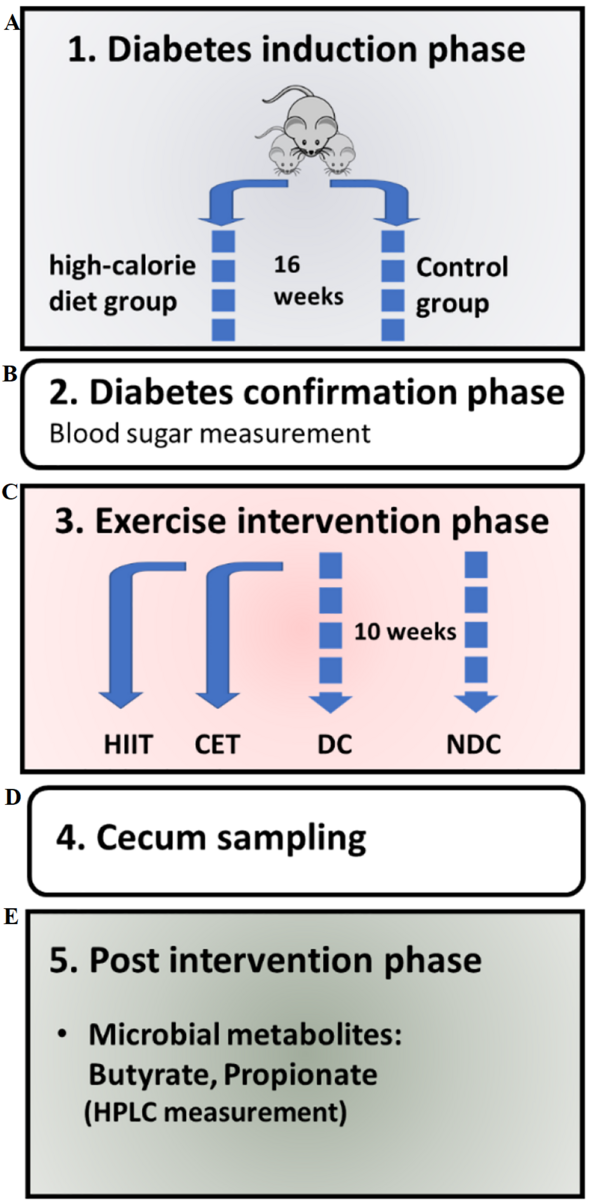
3.3. Analysis of Butyrate and Propionate Short-Chain Fatty Acids in Rat Cecum
Butyrate and propionate, key markers of exercise-induced gut microbiome changes (24), were quantified using an Agilent 1200 HPLC-UV system (25). Chromatographic separation was performed using an Eclipse XDB-C18 column. Data acquisition and processing were carried out using ChemStation software. The mobile phase consisted of a sodium monophosphate-water mixture and acetonitrile. Calibrators were prepared from 0.5 M and 0.05 M working solutions (Appendix 1 in Supplementary File). Sample preparation involved liquid-liquid extraction. After injecting 10 μL samples, elution through the column followed a specified schedule (Appendix 2 in Supplementary File).
3.4. Statistical Analysis
Experimental data from the four groups (NDC, DC, CET, HIIT), each with 8 members, were analyzed using SPSS (v22) and MATLAB (vR2020b). Normality was checked using the chi-square test. ANOVA was used to assess group differences, followed by the Tukey-Kramer test for multiple comparisons. Significance levels were set at 0.001, 0.01, and 0.05. Mean, median, standard deviation (STD), standard error (SE), and variation span were calculated, and outliers were removed. Linear regression (correlation coefficient: 0.99) was used to establish the chromatography test standard curve.
4. Results
4.1. Butyrate
The results of cecal butyric acid concentration measurements are depicted in Figure 2 and summarized in Appendix 3 in Supplementary File. In Figure 2A and B, a notable decrease in butyrate concentration from 11.73 mM to 7.05 mM was observed in the diabetic group, aligning with previous findings indicating the adverse impact of diabetes on SCFAs (26-28). Remarkably, the HIIT intervention led to a significant improvement in butyrate concentration. Conversely, CET exercise showed no significant effect on butyrate levels. Multiple comparisons indicated significant differences between the diabetic groups with HIIT (P = 0.036) and the non-diabetic groups with CET (P = 0.033). Furthermore, the one-way ANOVA test confirmed the effectiveness of exercise interventions on butyrate concentration (P = 0.0073, Appendix 4 in Supplementary File). In Figure 2C, the probability density function of the CET group leaned towards the DC group, while that of the HIIT group inclined towards the NDC group. The STD of the NDC and HIIT groups hovered around 1.8 mM, while that of the DC and CET groups was approximately 0.4 mM (Appendix 3 in Supplementary File).
Effect of exercise intervention on cecal butyrate concentration. A and B, bar and box plots illustrate the relative values and variation spans of butyrate concentration across the NDC, DC, CET, and HIIT groups. In B, red lines denote the medians. Statistical significance was determined using Tukey's multiple comparison test: *** P ≤ 0.001, ** P ≤ 0.01, and * P ≤ 0.05, with a sample size of n = 8. C, the probability density function depicts the normal distribution of butyrate concentration within the studied groups. Abbreviations: NDC, non-diabetic control; DC, diabetic control; CET, continuous endurance training; HIIT, high-intensity interval training.
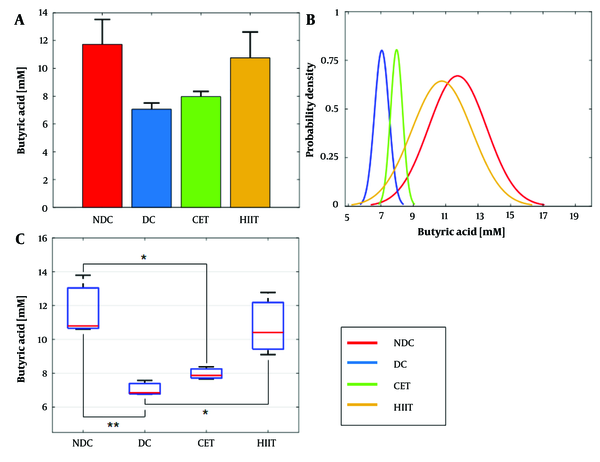
4.2. Propionate
Changes in propionic acid concentration were analyzed in relation to diabetes and exercise interventions, as illustrated in Figure 3 and summarized in Appendix 5 in Supplementary File. Notably, propionate concentration exhibited a significant reduction under the influence of diabetes, showing a 94% decrease compared to the non-diabetic group. In contrast, exercise interventions led to an increase in propionate concentration, with values of 0.6 mM for CET and 0.21 mM for HIIT. High-intensity interval training demonstrated superior efficacy in elevating propionate concentration compared to CET, with a statistically significant performance difference observed at a level of 0.032. The one-way ANOVA hypothesis test confirmed the impact of both diabetes and exercise interventions on cecal propionic acid concentration (P = 0.0013, Appendix 6 in Supplementary File for details) (Figure 3A and B). Probability density function analysis revealed an overlap between the HIIT group and non-diabetic subjects, while the CET group overlapped with diabetic subjects (Figure 3C). Statistical measures, including mean, STD, and SE for each group, are provided in Appendix 5 in Supplementary File for reference.
Effect of exercise intervention on cecal propionate concentration. A and B, bar and box plots illustrate the relative values and variation spans of propionate concentration across the NDC, DC, CET, and HIIT groups; B, red lines denote the medians. Statistical significance was determined using Tukey's multiple comparison test: *** P ≤ 0.001, ** P ≤ 0.01, and * P ≤ 0.05, with a sample size of n = 8; C, the probability density function depicts the normal distribution of propionate concentration within the studied groups. Abbreviations: NDC, non-diabetic control; DC, diabetic control; CET, continuous endurance training; HIIT, high-intensity interval training.
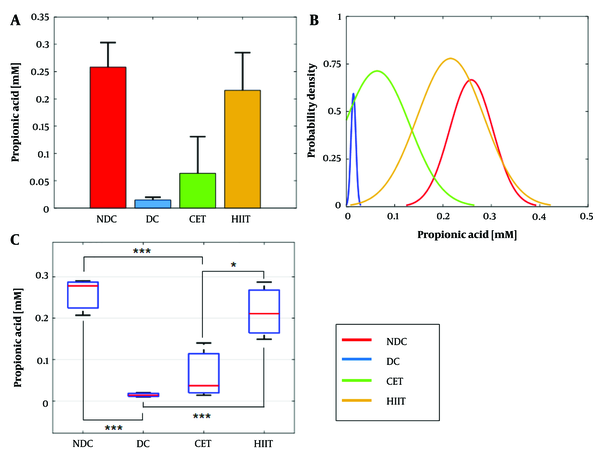
5. Discussion
In this study, the effects of two types of exercise, CET and HIIT, on the levels of butyrate and propionate metabolites and fasting blood glucose levels in diet-induced diabetic rats were investigated. Diabetes led to a significant decrease in both metabolites in the diabetic group compared to NDCs. Subsequently, the HIIT intervention, contrary to CET, substantially compensated for the reduction in both metabolites. Given that these two metabolites are the main energy sources for colonocytes (29), it can be expected that their concentration improvement by HIIT could enhance glycemic control and insulin sensitivity. This prediction aligns with the results of reduced levels of fasting blood sugar (FBS) and insulin resistance measured in the HIIT group compared to the DC group. On the other hand, despite CET not affecting the enhancement of butyrate and propionate production in the gut, it significantly reduced FBS and insulin resistance. This suggests that, besides gut microbial metabolites, other factors also influence blood sugar regulation and insulin sensitivity. Consistent with previous clinical studies (30-32), the present results demonstrate the positive impact of both HIIT and CET patterns in balancing blood sugar and modulating insulin resistance.
Considering the effect of HIIT on the concentration of the examined metabolites, it is also expected that HIIT plays a role in regulating the population of microbiota producing these SCFAs and alleviating dysbiosis. Among the anaerobic gut bacteria, butyrate is produced by Firmicutes and propionate by Firmicutes and Bacteroidetes (33). The positive effect of HIIT on the Firmicutes and Bacteroidetes phyla has been reported in previous studies (34-36), which is consistent with the findings of the current study and indirectly confirms it.
Here, we discuss possible mechanisms explaining the regulatory role of exercise on gut microbial metabolites, diabetes, and insulin resistance:
Exercise and SCFA production: Following exercise, gut microbiota synthesize various metabolites, including SCFAs (butyrate, propionate, acetate), which may explain HIIT’s positive effects on type 2 diabetes. Short-chain fatty acids improve insulin resistance through interactions with G-protein coupled receptors (GPRs), specifically GPR43 and GPR41 on intestinal L cells, which regulate digestion and satiety (3). However, the exact mechanism by which SCFAs enhance glucagon-like peptide 1 (GLP-1) secretion remains unclear.
Improved intestinal barrier function: Diabetes often impairs intestinal barrier function (11). However, regular exercise can improve intestinal permeability and endotoxemia in type 2 diabetes patients. This improvement may be due to an increase in SCFA-producing bacteria, especially butyrate producers. Butyrate promotes colonic regulatory T cell differentiation and anti-inflammatory cytokine production, strengthening the intestinal barrier (24, 37). Further research is needed to understand the exact mechanisms by which SCFAs affect this function.
Impact of exercise on intestinal transit time: Patients with type 2 diabetes have longer intestinal transit times due to elevated glucose levels, which reduce gastric and small intestine motility (38). This leads to increased nutrient absorption and energy intake, potentially disrupting energy homeostasis and contributing to obesity and type 2 diabetes. Shortening transit time appears to increase the prevalence of fast-growing bacterial species, most of which are SCFA producers, while microbes with longer replication times risk being washed out (39). Additionally, SCFAs, especially propionate, can increase GLP-1 secretion, which is released postprandially and helps boost insulin secretion from beta cells (7).
Exercise and improved microbial population: Anaerobic exercise routines like HIIT may boost the levels of butyrate and propionate in the gut by favoring beneficial SCFA-producing bacteria and reducing harmful BCAA-producing bacteria. Further studies on gut microbial composition are needed to confirm this.
5.1. Exercise and Gut Microbial Metabolites: Current Insights
5.1.1. Human Studies
The first indications that exercise has a role in modulating the human gut microbiome and the metabolic capacity of the microbiota were observed through cross-sectional studies (40). For instance, professional rugby players exhibited greater alpha diversity (41) and higher levels of amino acid and carbohydrate metabolic pathways, along with increased fecal SCFA (42) compared to sedentary individuals. Additionally, in non-athletic populations, women who exercised for at least three hours per week had higher levels of butyrate producers like Roseburia hominis, Faecalibacterium prausnitzii, and the mucin degrader Akkermansia muciniphila.
High-intensity combined aerobic and resistance training for 12 weeks in males with overweight and obesity led to decreased fecal and serum BCAA and aromatic amino acids (AA), and increased fecal propionate, GABA, and serum SCFA in exercise responders (19, 43). The lowering of plasma BCAA when performing high-intensity exercise was preceded by a significant increase in plasma lactate levels, showing that a significant attenuation of BCAA during incremental exercise coincides with exercise-induced acceleration of glycogen utilization. Additionally, it has been found (44) that moderate-intensity combined aerobic and resistance training for 8 weeks resulted in a greater decrease in urinary PAG and TMAO compared to a protein-only group. However, a similar study found no effects of exercise training on fecal SCFAs in individuals with type 2 diabetes (45). Also, in response to exercise, humans consistently show increases in Bacteroides and Roseburia. Bacteroides participates in metabolic pathways such as sphingolipid and SCFA production, bile acid metabolism, and mucus utilization (46-48). Roseburia primarily produces SCFAs, particularly butyrate, and is involved in conjugated fatty acid metabolism (46). Short-chain fatty acids and conjugated fatty acids regulate intestinal permeability, while SCFAs also contribute to energy homeostasis, glucose tolerance, and insulin sensitivity.
5.1.2. Animal Studies
Four weeks of aerobic training of varying durations (20, 40, or 60 minutes) in female C57BL/6 WT mice resulted in lower serum D-Lac, LPS, and DAO compared to an unrestricted running control (49). However, no differences in fecal metabolomics profiles were observed with aerobic training in overweight or obese mixed-breed horses undergoing weight loss (50). The differing responses to aerobic and anaerobic exercise align with our study's findings for CET and HIIT exercise patterns. In another animal model, Coprococcus, a SCFA producer, consistently increased with exercise (51). Generally, SCFA producers were found to increase with exercise in animal models.
5.2. Conclusions
The study findings highlight the effectiveness of HIIT in mitigating the negative impact of diabetes on the production of beneficial metabolites such as butyrate and propionate, leading to a significant increase in the concentration of these SCFAs. Additionally, HIIT has demonstrated superior efficacy over CET in reducing fasting blood glucose levels and insulin resistance. Based on these results, it is reasonable to expect that HIIT could contribute to the prevention and management of type 2 diabetes while potentially yielding superior enhancements in gut microbiota metabolic disorders compared to CET. However, a more comprehensive comparison necessitates further investigation into other relevant parameters.
References
-
1.
Hrncir T. Gut Microbiota Dysbiosis: Triggers, Consequences, Diagnostic and Therapeutic Options. Microorganisms. 2022;10(3). [PubMed ID: 35336153]. [PubMed Central ID: PMC8954387]. https://doi.org/10.3390/microorganisms10030578.
-
2.
Solouki S, Fazeli MR, Solouki S. [Efficiency of multispecies probiotic supplements in bioremoval of bisphenol A: An in vitro study]. Appl Food Biotechnol. 2018;5(1):37-45. FA.
-
3.
Rekha K, Venkidasamy B, Samynathan R, Nagella P, Rebezov M, Khayrullin M, et al. Short-chain fatty acid: An updated review on signaling, metabolism, and therapeutic effects. Crit Rev Food Sci Nutr. 2024;64(9):2461-89. [PubMed ID: 36154353]. https://doi.org/10.1080/10408398.2022.2124231.
-
4.
Portincasa P, Bonfrate L, Vacca M, De Angelis M, Farella I, Lanza E, et al. Gut Microbiota and Short Chain Fatty Acids: Implications in Glucose Homeostasis. Int J Mol Sci. 2022;23(3). [PubMed ID: 35163038]. [PubMed Central ID: PMC8835596]. https://doi.org/10.3390/ijms23031105.
-
5.
Etemadi A, Dabaghi P, Hosseini Y, Gholampourdehaki M, Solouki S, Gholamhosseini L, et al. Identifying depressive symptoms in patients with type 2 diabetes mellitus: the role of glucose variability and concomitant hypothyroidism. Int J Diabete Dev Countries. 2023;43. https://doi.org/10.1007/s13410-023-01177-5.
-
6.
Mohamed Elfadil O, Mundi MS, Abdelmagid MG, Patel A, Patel N, Martindale R. Butyrate: More Than a Short Chain Fatty Acid. Curr Nutr Rep. 2023;12(2):255-62. [PubMed ID: 36763294]. https://doi.org/10.1007/s13668-023-00461-4.
-
7.
McNabney SM, Henagan TM. Short Chain Fatty Acids in the Colon and Peripheral Tissues: A Focus on Butyrate, Colon Cancer, Obesity and Insulin Resistance. Nutrients. 2017;9(12). [PubMed ID: 29231905]. [PubMed Central ID: PMC5748798]. https://doi.org/10.3390/nu9121348.
-
8.
You H, Tan Y, Yu D, Qiu S, Bai Y, He J, et al. The Therapeutic Effect of SCFA-Mediated Regulation of the Intestinal Environment on Obesity. Front Nutr. 2022;9:886902. [PubMed ID: 35662937]. [PubMed Central ID: PMC9157426]. https://doi.org/10.3389/fnut.2022.886902.
-
9.
Solouki S, Mehrabi F, Mirzaii-Dizgah I. Localization of long-term synaptic plasticity defects in cerebellar circuits using optokinetic reflex learning profile. J Neural Eng. 2022;19(3). [PubMed ID: 35675762]. https://doi.org/10.1088/1741-2552/ac76df.
-
10.
Cochrane Central Register of Controlled Trials. Carbohydrate-induced Resilience of the Gut Microbiome After Antibiotics Use. Cochrane Central Register of Controlled Trials; 2020. Available from: https://www.cochranelibrary.com/central/doi/10.1002/central/CN-02181371/full.
-
11.
Sharma S, Tripathi P. Gut microbiome and type 2 diabetes: where we are and where to go? J Nutr Biochem. 2019;63:101-8. [PubMed ID: 30366260]. https://doi.org/10.1016/j.jnutbio.2018.10.003.
-
12.
Blaak EE, Canfora EE, Theis S, Frost G, Groen AK, Mithieux G, et al. Short chain fatty acids in human gut and metabolic health. Benef Microbes. 2020;11(5):411-55. [PubMed ID: 32865024]. https://doi.org/10.3920/BM2020.0057.
-
13.
Lin HV, Frassetto A, Kowalik EJ, Nawrocki AR, Lu MM, Kosinski JR, et al. Butyrate and propionate protect against diet-induced obesity and regulate gut hormones via free fatty acid receptor 3-independent mechanisms. PLoS One. 2012;7(4). e35240. [PubMed ID: 22506074]. [PubMed Central ID: PMC3323649]. https://doi.org/10.1371/journal.pone.0035240.
-
14.
Campbell SC, Wisniewski PJ, Noji M, McGuinness LR, Haggblom MM, Lightfoot SA, et al. The Effect of Diet and Exercise on Intestinal Integrity and Microbial Diversity in Mice. PLoS One. 2016;11(3). e0150502. [PubMed ID: 26954359]. [PubMed Central ID: PMC4783017]. https://doi.org/10.1371/journal.pone.0150502.
-
15.
Chomiuk T, Niezgoda N, Mamcarz A, Sliz D. Physical activity in metabolic syndrome. Front Physiol. 2024;15:1365761. [PubMed ID: 38440349]. [PubMed Central ID: PMC10910017]. https://doi.org/10.3389/fphys.2024.1365761.
-
16.
Strasser B. Physical activity in obesity and metabolic syndrome. Ann N Y Acad Sci. 2013;1281(1):141-59. [PubMed ID: 23167451]. [PubMed Central ID: PMC3715111]. https://doi.org/10.1111/j.1749-6632.2012.06785.x.
-
17.
Estaki M, Morck DW, Ghosh S, Quin C, Pither J, Barnett JA, et al. Physical Activity Shapes the Intestinal Microbiome and Immunity of Healthy Mice but Has No Protective Effects against Colitis in MUC2(-/-) Mice. mSystems. 2020;5(5). [PubMed ID: 33024049]. [PubMed Central ID: PMC7542559]. https://doi.org/10.1128/mSystems.00515-20.
-
18.
Pedersen HK, Gudmundsdottir V, Nielsen HB, Hyotylainen T, Nielsen T, Jensen BA, et al. Human gut microbes impact host serum metabolome and insulin sensitivity. Nature. 2016;535(7612):376-81. [PubMed ID: 27409811]. https://doi.org/10.1038/nature18646.
-
19.
Liu Y, Wang Y, Ni Y, Cheung CKY, Lam KSL, Wang Y, et al. Gut Microbiome Fermentation Determines the Efficacy of Exercise for Diabetes Prevention. Cell Metab. 2020;31(1):77-91 e5. [PubMed ID: 31786155]. https://doi.org/10.1016/j.cmet.2019.11.001.
-
20.
Packer N, Hoffman-Goetz L. Exercise training reduces inflammatory mediators in the intestinal tract of healthy older adult mice. Can J Aging. 2012;31(2):161-71. [PubMed ID: 22647663]. https://doi.org/10.1017/S0714980812000104.
-
21.
Egan B, Zierath JR. Exercise metabolism and the molecular regulation of skeletal muscle adaptation. Cell Metab. 2013;17(2):162-84. [PubMed ID: 23395166]. https://doi.org/10.1016/j.cmet.2012.12.012.
-
22.
Delfan M, Delphan M, Kordi MR, Ravasi AA, Safa M, Gorgani-Firuzjaee S, et al. High intensity interval training improves diabetic cardiomyopathy via miR-1 dependent suppression of cardiomyocyte apoptosis in diabetic rats. J Diabetes Metab Disord. 2020;19(1):145-52. [PubMed ID: 32550164]. [PubMed Central ID: PMC7270284]. https://doi.org/10.1007/s40200-019-00485-0.
-
23.
Omidifar A, Shirvani H, Taheri RA, Gorgani-Firouzjae S, Delfan M, Kalaki-Jouybari F, et al. Protective effects of HIIT vs. CET exercise training on high-fat-high-fructose diet-induced hyperglycemia, hyperlipidemia, and histopathology of liver in rats: regulation of SIRT1/PGC-1α. Sport Sci Health. 2021;17(3):707-15. https://doi.org/10.1007/s11332-021-00736-9.
-
24.
Bongiovanni T, Yin MOL, Heaney LM. The Athlete and Gut Microbiome: Short-chain Fatty Acids as Potential Ergogenic Aids for Exercise and Training. Int J Sports Med. 2021;42(13):1143-58. [PubMed ID: 34256388]. https://doi.org/10.1055/a-1524-2095.
-
25.
De Baere S, Eeckhaut V, Steppe M, De Maesschalck C, De Backer P, Van Immerseel F, et al. Development of a HPLC-UV method for the quantitative determination of four short-chain fatty acids and lactic acid produced by intestinal bacteria during in vitro fermentation. J Pharm Biomed Anal. 2013;80:107-15. [PubMed ID: 23542733]. https://doi.org/10.1016/j.jpba.2013.02.032.
-
26.
Tang R, Li L. Modulation of Short-Chain Fatty Acids as Potential Therapy Method for Type 2 Diabetes Mellitus. Can J Infect Dis Med Microbiol. 2021;2021:6632266. [PubMed ID: 33488888]. [PubMed Central ID: PMC7801078]. https://doi.org/10.1155/2021/6632266.
-
27.
Kim CH. Microbiota or short-chain fatty acids: which regulates diabetes? Cell Mol Immunol. 2018;15(2):88-91. [PubMed ID: 28713163]. [PubMed Central ID: PMC5811682]. https://doi.org/10.1038/cmi.2017.57.
-
28.
Mandaliya DK, Seshadri S. Short Chain Fatty Acids, pancreatic dysfunction and type 2 diabetes. Pancreatol. 2019;19(2):280-4. [PubMed ID: 30713129]. https://doi.org/10.1016/j.pan.2019.01.021.
-
29.
Litvak Y, Byndloss MX, Baumler AJ. Colonocyte metabolism shapes the gut microbiota. Sci. 2018;362(6418). [PubMed ID: 30498100]. [PubMed Central ID: PMC6296223]. https://doi.org/10.1126/science.aat9076.
-
30.
Safarimosavi S, Mohebbi H, Rohani H. High-Intensity Interval vs. Continuous Endurance Training: Preventive Effects on Hormonal Changes and Physiological Adaptations in Prediabetes Patients. J Strength Cond Res. 2021;35(3):731-8. [PubMed ID: 29939900]. https://doi.org/10.1519/JSC.0000000000002709.
-
31.
Batrakoulis A, Jamurtas AZ, Metsios GS, Perivoliotis K, Liguori G, Feito Y, et al. Comparative Efficacy of 5 Exercise Types on Cardiometabolic Health in Overweight and Obese Adults: A Systematic Review and Network Meta-Analysis of 81 Randomized Controlled Trials. Circ Cardiovasc Qual Outcomes. 2022;15(6). e008243. [PubMed ID: 35477256]. https://doi.org/10.1161/CIRCOUTCOMES.121.008243.
-
32.
Wang GH, Ruan L, Wu RP, Jin Z. Effects of aerobic exercise and resveratrol on adipocytokines in rats with nonalcoholic fatty liver disease. Sci Sports. 2023;38(1):57-67. https://doi.org/10.1016/j.scispo.2021.12.008.
-
33.
Louis P, Flint HJ. Formation of propionate and butyrate by the human colonic microbiota. Environ Microbiol. 2017;19(1):29-41. [PubMed ID: 27928878]. https://doi.org/10.1111/1462-2920.13589.
-
34.
Solouki S, Fazeli MR. [The effect of commercial probiotic formulations on reduction of bisphenol-A in-vitro]. J Appl Microbiol Food Ind. 2017;3(2):9-21. FA.
-
35.
Denou E, Marcinko K, Surette MG, Steinberg GR, Schertzer JD. High-intensity exercise training increases the diversity and metabolic capacity of the mouse distal gut microbiota during diet-induced obesity. Am J Physiol Endocrinol Metab. 2016;310(11):E982-93. [PubMed ID: 27117007]. [PubMed Central ID: PMC4935139]. https://doi.org/10.1152/ajpendo.00537.2015.
-
36.
Motiani KK, Collado MC, Eskelinen JJ, Virtanen KA, Loyttyniemi E, Salminen S, et al. Exercise Training Modulates Gut Microbiota Profile and Improves Endotoxemia. Med Sci Sports Exerc. 2020;52(1):94-104. [PubMed ID: 31425383]. [PubMed Central ID: PMC7028471]. https://doi.org/10.1249/MSS.0000000000002112.
-
37.
Mayorga-Ramos A, Barba-Ostria C, Simancas-Racines D, Guaman LP. Protective role of butyrate in obesity and diabetes: New insights. Front Nutr. 2022;9:1067647. [PubMed ID: 36505262]. [PubMed Central ID: PMC9730524]. https://doi.org/10.3389/fnut.2022.1067647.
-
38.
Suryani D, Subhan Alfaqih M, Gunadi JW, Sylviana N, Goenawan H, Megantara I, et al. Type, Intensity, and Duration of Exercise as Regulator of Gut Microbiome Profile. Curr Sports Med Rep. 2022;21(3):84-91. [PubMed ID: 35245243]. https://doi.org/10.1249/JSR.0000000000000940.
-
39.
Dabke K, Hendrick G, Devkota S. The gut microbiome and metabolic syndrome. J Clin Invest. 2019;129(10):4050-7. [PubMed ID: 31573550]. [PubMed Central ID: PMC6763239]. https://doi.org/10.1172/JCI129194.
-
40.
Mailing LJ, Allen JM, Buford TW, Fields CJ, Woods JA. Exercise and the Gut Microbiome: A Review of the Evidence, Potential Mechanisms, and Implications for Human Health. Exerc Sport Sci Rev. 2019;47(2):75-85. [PubMed ID: 30883471]. https://doi.org/10.1249/JES.0000000000000183.
-
41.
Clarke SF, Murphy EF, O'Sullivan O, Lucey AJ, Humphreys M, Hogan A, et al. Exercise and associated dietary extremes impact on gut microbial diversity. Gut. 2014;63(12):1913-20. [PubMed ID: 25021423]. https://doi.org/10.1136/gutjnl-2013-306541.
-
42.
Barton W, Penney NC, Cronin O, Garcia-Perez I, Molloy MG, Holmes E, et al. The microbiome of professional athletes differs from that of more sedentary subjects in composition and particularly at the functional metabolic level. Gut. 2018;67(4):625-33. [PubMed ID: 28360096]. https://doi.org/10.1136/gutjnl-2016-313627.
-
43.
Gawedzka A, Grandys M, Duda K, Zapart-Bukowska J, Zoladz JA, Majerczak J. Plasma BCAA concentrations during exercise of varied intensities in young healthy men-the impact of endurance training. PeerJ. 2020;8. e10491. [PubMed ID: 33391874]. [PubMed Central ID: PMC7759138]. https://doi.org/10.7717/peerj.10491.
-
44.
Cronin O, Barton W, Skuse P, Penney NC, Garcia-Perez I, Murphy EF, et al. A Prospective Metagenomic and Metabolomic Analysis of the Impact of Exercise and/or Whey Protein Supplementation on the Gut Microbiome of Sedentary Adults. mSystems. 2018;3(3). [PubMed ID: 29719871]. [PubMed Central ID: PMC5915698]. https://doi.org/10.1128/mSystems.00044-18.
-
45.
Torquati L, Gajanand T, Cox ER, Willis CRG, Zaugg J, Keating SE, et al. Effects of exercise intensity on gut microbiome composition and function in people with type 2 diabetes. Eur J Sport Sci. 2023;23(4):530-41. [PubMed ID: 35107058]. https://doi.org/10.1080/17461391.2022.2035436.
-
46.
Ghosh S, Whitley CS, Haribabu B, Jala VR. Regulation of Intestinal Barrier Function by Microbial Metabolites. Cell Mol Gastroenterol Hepatol. 2021;11(5):1463-82. [PubMed ID: 33610769]. [PubMed Central ID: PMC8025057]. https://doi.org/10.1016/j.jcmgh.2021.02.007.
-
47.
Wang X, Hu Y, Zhu X, Cai L, Farooq MZ, Yan X. Bacteroides-derived isovaleric acid enhances mucosal immunity by facilitating intestinal IgA response in broilers. J Anim Sci Biotechnol. 2023;14(1):4. [PubMed ID: 36604758]. [PubMed Central ID: PMC9817248]. https://doi.org/10.1186/s40104-022-00807-y.
-
48.
Johnson EL, Heaver SL, Waters JL, Kim BI, Bretin A, Goodman AL, et al. Sphingolipids produced by gut bacteria enter host metabolic pathways impacting ceramide levels. Nat Commun. 2020;11(1):2471. [PubMed ID: 32424203]. [PubMed Central ID: PMC7235224]. https://doi.org/10.1038/s41467-020-16274-w.
-
49.
Chen H, Shen L, Liu Y, Ma X, Long L, Ma X, et al. Strength Exercise Confers Protection in Central Nervous System Autoimmunity by Altering the Gut Microbiota. Front Immunol. 2021;12:628629. [PubMed ID: 33796102]. [PubMed Central ID: PMC8007788]. https://doi.org/10.3389/fimmu.2021.628629.
-
50.
Walshe N, Cabrera-Rubio R, Collins R, Puggioni A, Gath V, Crispie F, et al. A Multiomic Approach to Investigate the Effects of a Weight Loss Program on the Intestinal Health of Overweight Horses. Front Vet Sci. 2021;8:668120. [PubMed ID: 34222398]. [PubMed Central ID: PMC8249564]. https://doi.org/10.3389/fvets.2021.668120.
-
51.
Castro AP, Silva KKS, Medeiros CSA, Alves F, Araujo RC, Almeida JA. Effects of 12 weeks of resistance training on rat gut microbiota composition. J Exp Biol. 2021;224(12). [PubMed ID: 34137868]. https://doi.org/10.1242/jeb.242543.