Abstract
Background:
Peripheral nerve injuries remain a great challenge for microsurgery despite the significant progress in recent decades. The current gold standard is autogenous nerve grafting with a success rate as low as 50% in long gaps. Current studies have focused on finding alternative methods for bridging nerve defects. Previous data have demonstrated the role of human amniotic membrane in stimulating neural regeneration. On the other hand, adipose-derived mesenchymal stem cells can differentiate into all three germ layers and could support nerve repair. The purpose of this study was to compare the role of the human amniotic membrane with and without adipose tissue stem cells in sciatic nerve injury with gap in rats.Objectives:
We aimed to evaluate the effectiveness of the human amniotic membrane with and without adipose-derived mesenchymal stem cells in sciatic nerve injury with gap in rats.Methods:
Twenty-four male Wistar rats in four random groups were used in our study. In the first group, the nerve gap was repaired using the inverse resected nerve segment (Control group), the second group was repaired with a human amniotic membrane (AM group), the third group was repaired with an amnion sheet with seeded adipose-derived mesenchymal stem cells (AM/ADMSCs group), and the last group was not repaired, and both stumps were sutured to muscles.Results:
All the animals underwent the procedures and survived without complication. The sciatic function index and hot plate test results were significantly improved in the AM and AM/ADMSCs groups compared to the Control group (as a gold standard of care) (P>0.05). Based on histopathology findings, regenerative nerve fibers were seen in the implanted area of both AM and AM/ADMSCs groups; however, nerve fibers were surrounded by significant fibrosis (scar formation) in the AM/ADMSCs group. The axon count in the Control group was significantly higher than both experimental groups (P < 0.01).Conclusions:
Our study showed the role of amniotic membrane in the promotion of nerve regeneration in sciatic nerve injury with a gap, but adding adipose-derived mesenchymal stem cells not only has no extra benefits, but also causes more tissue scar.Keywords
Adipose-Derived Stem Cells Human Amniotic Membrane Peripheral Nerve Injuries
1. Background
Peripheral nerve injuries are common due to trauma, some diseases, and surgical complications, and their surgical management remains a significant challenge for clinicians (1-4). Annually, millions of people suffer from painful neuropathies and sensory-motor deficits due to peripheral nerve injuries (5). Despite numerous advances in clinical studies and laboratory experiments, peripheral nerve repair, especially in cases with nerve gaps, has a poor outcome (6-9). Wallerian degeneration (WD) occurs following peripheral nerve damage. During this process, myelin is removed from axons, and then axons are disintegrated and destroyed distally toward the site of injury (10-15). In the regeneration phase, axons begin to regrow from the proximal stump, and the target organ is reinnervated under ideal circumstances (16, 17). Direct neurorrhaphy is a standard procedure for nerve injury repair, but it works when the neural gap is smaller than 5 mm, and nerve stumps can be sutured without tension. The current gold standard for longer gap is autogenous nerve grafting (18-21), with a success rate as low as 50%. In addition to the low success rate, it has some other disadvantages, including inevitable sensory deficit, painful neuroma at the donor site, donor site scar, prolonged surgical time, and limitation of graft material in cases of extensive lesions (e.g., brachial plexus) (18-20, 22).
Previous studies have shown that neurotrophic factors influence the nerve regeneration process. Both distal and proximal stumps of the injured nerve release trophic factors supporting the growth of dorsal root ganglion. Nerve ends produce both neurotrophic factors and extracellular matrix components with contact guidance and hormonal support to develop and advance axons regeneration. However, surroundings invade the environment provided by nerve ends. In fact, inflammatory factors and cells from the surrounding tissue can cause adverse effects on nerve regeneration and tissue scar. Therefore, a major concern is the isolation of the neural gap from the surrounding tissue to provide optimum intrinsic conditions.
Current studies have focused on finding alternative methods for bridging nerve defects. Previous experiments have examined venous grafts as well as artificial and natural conduits (21, 23-32). Today's methods can support nerve regeneration; however, they have not consistently replicated the results obtained by autologous nerve transplantation (33). The reason for using conduits is creating a protective channel through which regeneration can occur in a more straightforward manner. Ideal conduit materials for a successful neural regeneration should be biocompatible to cause minimal inflammation and able to stimulate axonal regeneration. A neural conduit must also have specific mechanical properties, such as flexibility and comfortable handling (34-38).
The human amniotic membrane (hAM) is abundantly available and easily extracted, being rich in collagen, laminin, fibronectin, and other basement membrane components (39-41). Previous data have demonstrated numerous clinical applications of amniotic membranes. Davis et al. showed that the hAM could stimulate neural regeneration in vivo and in vitro (39). Other studies demonstrated the hAM's capability to limit scar formation and adhesions when used as a wrapped conduit around the repaired nerve (42). The hAM consists of specific proteins, including collagen, laminin, and proteoglycans; therefore, it is used as a nerve conduit in rat (43, 44) and rabbit models for nerve repair (42, 45).
Recent studies have concentrated on the utility and capability of stem cells in peripheral nerve repair. Studies demonstrated the differentiation ability of bone marrow stem cells (BMSCs) into numerous cell lineages, including neurons, astrocytes, oligodendrocytes, and Schwann cells (46, 47). Experimental studies have revealed the role of these cells in improving peripheral nerve function after repair. Adipose-derived mesenchymal stem cells (ADMSCs) are capable of differentiating into functional neurons (48-50) and can support nerve repair (19). Adipose-derived mesenchymal stem cells produce large numbers of growth factors that can promote the development and function of the peripheral nervous system (50).
2. Objectives
The purpose of this study was to evaluate the effectiveness of the hAM with and without adipose-derived mesenchymal stem cells in sciatic nerve injury with a gap in rats.
3. Methods
3.1. Animal Experiments
Twenty-four male Wistar rats (200 - 250 gr) were used for the experiments. They were randomized into four groups. The study design was approved by Iran National Committee for Ethics in Biomedical Research (Approval ID: IR.TUMS.NI.REC.1398.039). All the experimental procedures were carried out under the regulations and ethical considerations in animal experiments at Tehran University of Medical Sciences. The animals were kept in a room with controlled temperature and humidity (20 - 25°C, 70 - 80%, respectively) and in individual cages. They were exposed to 12 hours of daylight and fed according to the standard protocols. Twelve hours before surgery, they were only given access to water. After surgery, they received pain medications.
3.2. Procedure
The animals were anesthetized using the intraperitoneal injection of the mixture of 50 mg/kg ketamine and 5 mg/kg xylazine. The right sciatic nerve was aseptically explored in mid-thigh, and then an 8-mm nerve segment was resected above the bifurcation area.
In the first group, the nerve gap was repaired using the inverse resected nerve segment (Control Group). In the amnion membrane group (AM group), the distal and proximal stumps were sutured to the ends of the viable amnion sheet. Then, the amnion was rolled around both stumps to form a tube which filled the nerve gap. Finally, the amnion sheet overlapped with both nerve stumps (Figure 1). The same procedure was applied in the stem cell group, except for using an amnion sheet with seeded ADMSCs (AM/ADMSCs group). In the last group, the nerve gap was not repaired (Negative Control).
Amnion sheet was formed a tube which filled the nerve gap
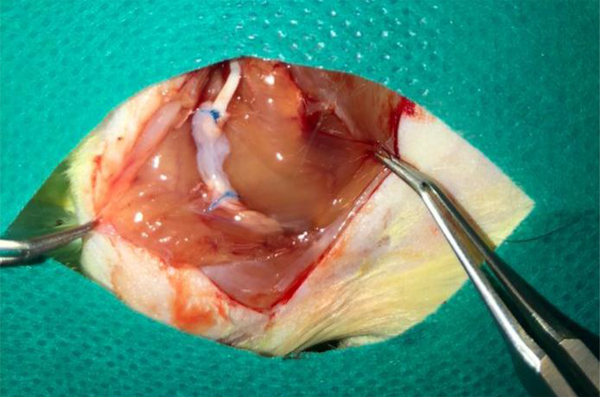
3.3. Preparation of Denuded Amniotic Membrane (dAM)
Fetal membranes were collected during elective cesarean section after obtaining written informed consent from healthy donors. All donors were screened with our donor's inclusion criteria. The donor's blood sample was taken for laboratory tests. They were examined for HIV, HBV, HCV, HTLV, and venereal diseases. The harvested tissues were washed thoroughly with sterile injectable normal saline (Samen Pharmaceuticals, Iran), packed in sterile organ bags, and transferred to the processing facility. Amniotic membrane processing was done according to a previously published protocol (51). The AM was separated from the harvested membranes and then washed with cold DPBS including antibiotic and antimycotic (Biowest, France) three times. Then, it was spread over a sterile nitrocellulose sheet (Whatman, USA) and cut into 3 × 3 cm portions. We put each piece into a sterile polypropylene tube (SPL, Korea) and cryopreserved it at -80°C. The tubes contained 50% V/V DMEM (Biowest, France) and glycerol (Sigma-Aldrich, USA). At the time of the surgery, the cryopreserved AMs were defrosted and washed with DPBS. To remove the epithelial cell layer, AM pieces were incubated in 0.05% Trypsin-EDTA (Biowest, France) at 37°C for 30 min. Then, we removed epithelial cells by a cell scraper (SPL, Korea) and washed with DPBS. To confirm the decellularization process, the control samples were fixed with ice-cold methanol, stained with hematoxylin, and examined under microscope (Nikon, Japan).
3.4. ADMSCs seeding on dAM
Cryopreserved ADMSCs (third passage) were prepared by the Cell Therapy and Regenerative Medicine Research Center (Tehran, Iran). These cells were manufactured and fully characterized according to their protocols (52). dAM pieces were transferred to a non-treated six-well culture plate (SPL, Korea) immersed in culture media (DMEM + 5% human platelet lysate [PL BioScience GmbH, Germany]) and incubated in a CO2 incubator (37°C, 5% CO2, 98% humidity) for 30 min. Meanwhile, the cryopreserved ADMSCs were defrosted at 37°C and then washed twice in the culture media and centrifuged at 200 g/5 minutes, counted, and re-suspended in fresh culture media at a concentration of 3 × 104 viable cells/ml. Then, the media were discarded, and the dAMs were washed with DPBS. Adipose-derived mesenchymal stem cells were seeded on dAMs. In each one, we used 3 cc of suspension, and then they were incubated (37°C, 5% CO2, 98% humidity). Fresh culture media was added 24 h later. On day 5, the prepared construct (dAM-ADMSCs) was ready for transplantation. dAM-ADMSCs and dAMs were kept in polypropylene tubes with phosphate-buffered saline (PBS). To confirm ADMSCs adhesion, representative samples were stained with hematoxylin. Furthermore, samples of dAM-ADMSCs were stained with mouse anti-human Vimentin antibody (eBioscience, Cat.No:14-9897-80). The specimens were fixed in 4% paraformaldehyde (20 minutes), then they were washed in PBS-Tween (Atocel, Austria) three times. Permeabilizing was done using 0.1% Triton X-100 (Atocel, Austria) (10 minutes), and finally, they were blocked in 5% goat serum for 1 h. dAM-ADMSCs were incubated in primary antibodies (anti-human Vimentin antibody and mouse anti-human IgG1 isotype control [eBioscience, Cat.No: 14-4714-81]) for 1 h at 37°C. To detect reaction, 3,3' Diaminobenzidine (DAB) staining kit (Dako, Denmark) was used according to the manufacturer's instruction. The stained samples were all examined under microscope (Nikon, Japan).
3.5. Functional Recovery Evaluation
Walking track patterns were evaluated 4, 8, and 12 weeks after surgery. Footprints were recorded through walking across a tunnel. Three indices, including distance between the third toe to the heel (PL), the second finger to the fourth one (IT), and the first to the fifth finger (TS), were measured. These measurements were made in both intact and operated feet. We used the below formula for calculating the sciatic functional index (SFI) (43).
3.6. Withdrawal Reflex Latency Test
Withdrawal reflex latency was measured at the same follow-up periods (4, 8, 12 weeks after surgery) in all the groups. It is an experimental method used for assessing nociception in rats. The rats were placed on a hotplate at 56°C with the affected hind paw. The latency to lick hind paw or jump out of the enclosure were measured with a stopwatch. At this point, the animal was removed from the hot plate.
3.7. Histopathology
In the last follow-up, 12 weeks after surgery, the animals were sacrificed. The sciatic nerve (in graft site) was removed and fixed in 10% neutral buffered formalin (NBF, PH. 7.26) for 48 h. The paraffin-embedded specimen blocks were cut into sections with a 5-µm thickness. Transverse sections of the nerve graft site were stained with both hematoxylin and eosin (H&E) and toluidine blue (TB). Then, the histological slides were examined under light microscopy (Olympus BX51; Olympus, Tokyo, Japan) by a pathologist (Blind Evaluation).
3.8. Statistical Analysis
Results are reported as the mean ± STD. For comparing the axon counts, we used one-way ANOVA and Tukey’s post hoc test in GraphPad Prism, version 7. To compare the SFI index and hot plate test results in different post-surgery follow-ups, we used One-way Repeated Measures Analysis test.
4. Results
4.1. dAM Preparation and ADMSCs Seeding
The results of hematoxylin staining demonstrated that AMs were successfully decellularized using our protocol (Figure 2A and B). Furthermore, hematoxylin and anti-Vimentin staining of dAM-MSCSs indicated that ADMSCs could attach and expand on dAMs (Figure 2B and C).
Hematoxylin staining of intact AM (A), dAM (B), and ADMSCs seeded AM (C). Anti-vimentin immunohistochemistry result of ADMSCs seeded AM (D).
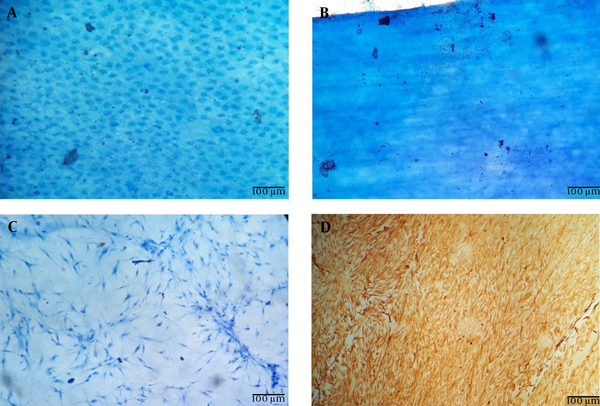
4.2. Animal Study Results
All the animals underwent the above-mentioned procedures and survived without any specific complications.
Sciatic functional index values in all the experimental groups in the three follow-up periods are shown in Figure 3. The results showed that the interventions significantly affected SFI values in all the groups. The estimated effect size was 0.93 (P < 0.05, F = 37.2). Table 1 shows SFI mean in all the groups based on the follow-up time. Four weeks after the surgery, the AM group had a statistically significant improvement in the SFI index compared to the Negative Control (P = 0.02). There was no significant difference between the other groups in the fourth week of the study (P > 0.05). In the next follow-up assessment, eight weeks after surgery, the SFI in both AM and Control groups increased compared to the Negative Control and AM/ADMSCs groups (-31.08, -32.37 vs. -81.86, -83.62, respectively P < 0.05). Finally, 12 weeks after surgery, the SFI value in the AM, AM/ADMSCs, and Control groups reached -20.48, -11.52, -12.8, respectively. There was no significant difference between these groups in SFI improvement at 12 weeks after surgery (P > 0.05). But all these three groups had a significantly higher improvement in SFI value at 12 weeks after surgery.
SFI values in the three follow-up periods
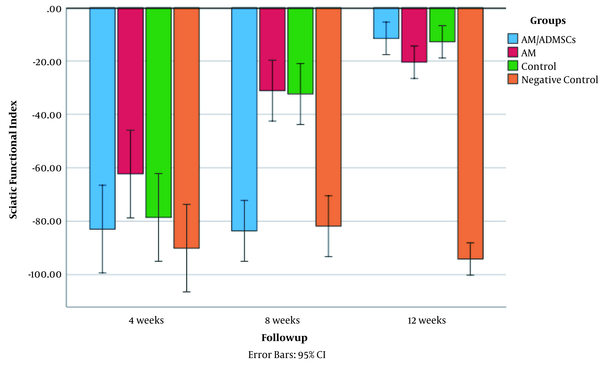
4.3. Hot Plate Test
Hot plate test results in the three follow-up periods are shown in Figure 4. Interventions significantly affected hot plate test values in all the groups. The estimated effect size was 0.99 (P < 0.05, F = 3.7). Hot plate test values in all the groups based on the follow-up time are presented in Figure 4. Four weeks after surgery, there was no significant improvement in hot plate test in the groups (P < 0.05). In the second follow-up (8 weeks after surgery), the AM and Control groups had a significant improvement in hot plate test results compared to the Negative Control group (Means 9.60, 9.82 vs 11.70, respectively; P < 0.05). Twelve weeks after surgery, there were statistically significant improvements in hot plate test results of the experimental groups compared to the Negative Control group (9.70, 9.66, 9.90 vs 11.17, P < 0.05). However, there was no significant difference among the experimental groups (P > 0.05).
Hot plate test results in the three follow-up periods
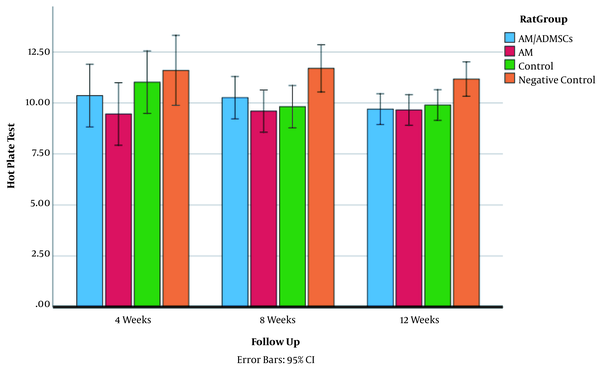
4.4. Histopathological Studies
The macroscopic evaluation of the repaired sciatic nerve exhibited that there was more scar and adhesion in the AM/ADMSCs groups (Figure 5).
Macroscopic view of the repaired sciatic nerve 12 weeks after surgery
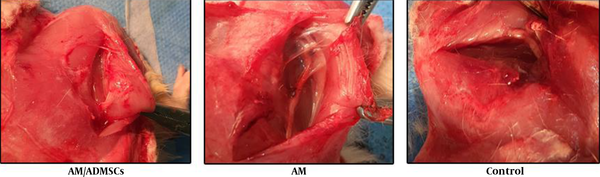
The arrangement of fibers was disturbed in the Control group, and the axons were swollen with vacuolation.
In the AM group, regenerating nerve fibers were seen in the implanted conduit. The implanted conduit was not distinguishable from the peripheral fibrotic tissues. The micrographs of the sciatic nerve in the AM/ADMSCs group showed a close resemblance to AM treatment in terms of regenerated nerve fibers. However, the nerve fibers were surrounded by significant fibrosis (scar formation). Moreover, the implanted conduits induced angiogenesis, as seen in Figure 6 (magnified windows). The number of myelinated nerve fibers in the AM and AM/ADMSCs treatment groups was considerably lower in comparison to those of the normal and control groups (Table 1).
Light micrographs of the sciatic nerve. H&E, TB staining, magnification x40: 300 µm, X400:50 µm. blue arrows: myelinated fibers, thick black arrows: fibrotic tissues, thin black arrows: vacuolation.
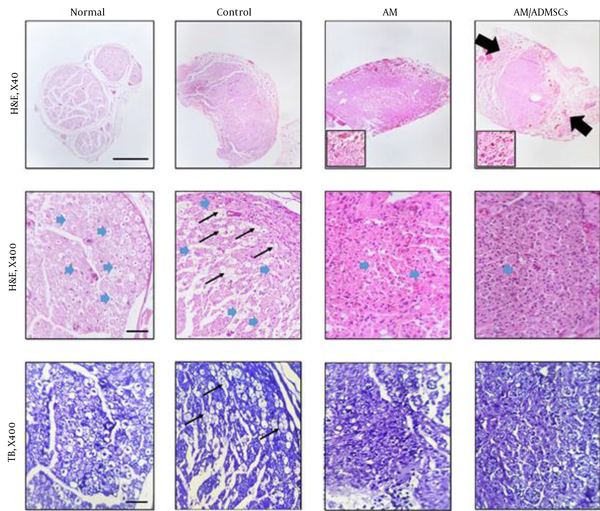
Histomorphometric Analysis of the Sciatic Nerve 12 Weeks Post-sciatic Nerve Injury
Pairwise Comparisons | HSD0.05 = 8.3858, HSD0.01 = 10.7219 | Q0.05 = 4.0200, Q0.05 = 5.1399 |
---|---|---|
T1:T2a | ||
M1 = 186.38, M2 = 129.03 | 57.35 | Q = 27.49 (P = 0.00000) |
T1:T3 | ||
M1 = 186.38, M3 = 50.95 | 135.43 | Q = 64.92 (P = 0.00000) |
T1:T4 | ||
M1 = 186.38, M4 = 28.10 | 158.27 | Q = 75.87 (P = 0.00000) |
T2:T3 | ||
M2 = 129.03, M3 = 50.95 | 78.08 | Q = 37.43 (P = 0.00000) |
T2:T4 | ||
M2 = 129.03, M4 = 28.10 | 100.92 | Q = 48.38 (P = 0.00000) |
T3:T4 | ||
M3 = 50.95, M4 = 28.10 | 22.85 | Q = 10.95 (P = 0.00000) |
Overall, the histomorphometric analysis of these treatments revealed that axon counts in the normal and control groups were significantly higher than those of the experimental groups (P < 0.01). Moreover, this analysis showed that axon count was considerably higher in the AM treatment group than that of the AM/ADMSCs conduit treatment group (P < 0.01).
5. Discussion
The current study revealed that rat sciatic regeneration in both experimental groups (AM & AM/ADMSCs) was comparable with the Control group (as the gold standard of care). As the pathological findings showed, nerve fibers were grown through the nerve gaps. The axons counts in the experimental groups were less than that of the Control group. The micrographs of the sciatic nerve in the AM/ADMSCs group showed a close resemblance to those of the AM group in terms of regenerated nerve fibers. However, nerve fibers were surrounded with significant fibrosis (scar formation).
Using the hAM alone or with seeded-on bone marrow stromal cells as nerve conduit demonstrated to promote functional recovery in spinal cord injury (53). The hAM cells, including human amniotic mesenchymal stromal cells and human amniotic epithelial cells, have displayed neurotrophic factors secretion ability, promoting nerve regeneration (54). These factors consist of neurotrophin-3, brain-derived neurotrophic factors, nerve growth factors, ciliary neurotrophic factor, and glial cell-derived neurotrophic factor. In previous studies, the hAM was used in the acellular form in nerve tissue engineering. However, recent studies showed that it contains cells with a high potential to promote nerve regeneration. Banerjee et al. demonstrated that viable hAM could be differentiated towards the Schwann cell lineage and secrete neurotrophic factors (55). Some studies showed that isolation of the repaired nerve by using the amnion could protect the repaired site from external inflammatory events (43). In some studies, wrapped amnion sheets protected the neurorrhaphy site from adhesion and promoted functional recovery (45).
In this study, we used the viable form of the hAM and the acellular one with ADSCs seeding. According to the functional recovery test, there was no significant difference between the experimental and Control groups at the end of the study. Thus, the results indicated that adding adipose-derived mesenchymal stem cells to the human amniotic membrane failed to cause any significant changes in nerve recovery outcome, although it causes further fibrosis tissue. This study showed that the hAM could facilitate nerve regeneration due to its growth factors and anti-scarring, anti-inflammatory, and low immunogenicity properties.
On the other hand, recent studies have focused on the role of stem cells in peripheral nerve repair. Kingham et al. (56) demonstrated that the treatment of adipose-derived mesenchymal stem cells with a combination of Schwann cell mitogenic could express the p75 neurotrophic receptor, glial fibrillary acidic protein, and glial cell markers S100B. Other studies showed positive results regarding the high quantity of peripheral nerve injuries (57, 58) and revealed that AM/ADMSCs produce numerous growth factors, which play a key role in the peripheral nervous system (45).
In the present study, the improvement of the SFI index in the AM group was comparable with that of the Control group at 8 and 12 weeks after surgery. The same result was reported in Mohammad et al. (2000) study (20). Forootan et al. (2011) showed that end-to-end anastomosis with amniotic membrane coverage had a better improvement in SFI index compared to the simple end-to-end anastomosis (59). Thus, their results were in agreement with ours regarding the neurosupportive role of the amniotic membrane. In another study, Sadraie et al. showed similar results (60).
5.1. Conclusions
Our study showed the role of the hAM in the promotion of nerve regeneration in sciatic nerve injury with a gap, but adding adipose-derived mesenchymal stem cells not only had no extra benefit, but also caused more tissue scar.
References
-
1.
Millesi H. Nerve grafting. Clin Plast Surg. 1984;11(1):115-20. https://doi.org/10.1016/s0094-1298(20)31826-5.
-
2.
Mackinnon SE. New directions in peripheral nerve surgery. Ann Plast Surg. 1989;22(3):257-73. [PubMed ID: 2660716]. https://doi.org/10.1097/00000637-198903000-00013.
-
3.
Fernandez E, Pallini R, Lauretti L, Scogna A. Neurosurgery of the peripheral nervous system: Injuries, degeneration, and regeneration of the peripheral nerves. Surg Neurol. 1997;48(5):446-7. https://doi.org/10.1016/s0090-3019(96)00545-9.
-
4.
Seckel BR. Enhancement of peripheral nerve regeneration. Muscle Nerve. 1990;13(9):785-800. [PubMed ID: 2233865]. https://doi.org/10.1002/mus.880130904.
-
5.
Daly W, Yao L, Zeugolis D, Windebank A, Pandit A. A biomaterials approach to peripheral nerve regeneration: bridging the peripheral nerve gap and enhancing functional recovery. J R Soc Interface. 2012;9(67):202-21. [PubMed ID: 22090283]. [PubMed Central ID: PMC3243399]. https://doi.org/10.1098/rsif.2011.0438.
-
6.
Wood MD, Kemp SW, Weber C, Borschel GH, Gordon T. Outcome measures of peripheral nerve regeneration. Ann Anat. 2011;193(4):321-33. [PubMed ID: 21640570]. https://doi.org/10.1016/j.aanat.2011.04.008.
-
7.
Lundborg G. Nerve injury and repair: Regeneration, reconstruction, and cortical remodeling. Elsevier/Churchill Livingstone; 2004.
-
8.
Meek MF, Coert JH, Robinson PH. Poor results after nerve grafting in the upper extremity: Quo vadis? Microsurgery. 2005;25(5):396-402. [PubMed ID: 16032723]. https://doi.org/10.1002/micr.20137.
-
9.
Weber RV, Mackinnon SE. Bridging the neural gap. Clin Plast Surg. 2005;32(4):605-16. viii. [PubMed ID: 16139631]. https://doi.org/10.1016/j.cps.2005.05.003.
-
10.
Bradl M, Linington C. Animal models of demyelination. Brain Pathol. 1996;6(3):303-11. [PubMed ID: 8864286]. [PubMed Central ID: PMC7161775]. https://doi.org/10.1111/j.1750-3639.1996.tb00857.x.
-
11.
Chen ZL, Yu WM, Strickland S. Peripheral regeneration. Annu Rev Neurosci. 2007;30:209-33. [PubMed ID: 17341159]. https://doi.org/10.1146/annurev.neuro.30.051606.094337.
-
12.
Koltzenburg M, Bendszus M. Imaging of peripheral nerve lesions. Curr Opin Neurol. 2004;17(5):621-6. [PubMed ID: 15367867]. https://doi.org/10.1097/00019052-200410000-00013.
-
13.
Misgeld T. Death of an axon: Studying axon loss in development and disease. Histochem Cell Biol. 2005;124(3-4):189-96. [PubMed ID: 16133121]. https://doi.org/10.1007/s00418-005-0036-6.
-
14.
Stoll G, Trapp BD, Griffin JW. Macrophage function during Wallerian degeneration of rat optic nerve: clearance of degenerating myelin and Ia expression. J Neurosci. 1989;9(7):2327-35. [PubMed ID: 2787393]. [PubMed Central ID: PMC6569766].
-
15.
Stoll G, Griffin JW, Li CY, Trapp BD. Wallerian degeneration in the peripheral nervous system: participation of both Schwann cells and macrophages in myelin degradation. J Neurocytol. 1989;18(5):671-83. [PubMed ID: 2614485]. https://doi.org/10.1007/BF01187086.
-
16.
Verma P, Chierzi S, Codd AM, Campbell DS, Meyer RL, Holt CE, et al. Axonal protein synthesis and degradation are necessary for efficient growth cone regeneration. J Neurosci. 2005;25(2):331-42. [PubMed ID: 15647476]. [PubMed Central ID: PMC3687202]. https://doi.org/10.1523/JNEUROSCI.3073-04.2005.
-
17.
Tomita K, Kubo T, Matsuda K, Yano K, Tohyama M, Hosokawa K. Myelin-associated glycoprotein reduces axonal branching and enhances functional recovery after sciatic nerve transection in rats. Glia. 2007;55(14):1498-507. [PubMed ID: 17705198]. https://doi.org/10.1002/glia.20566.
-
18.
Cullheim S, Wallquist W, Hammarberg H, Lindå H, Piehl F, Carlstedt T, et al. Properties of motoneurons underlying their regenerative capacity after axon lesions in the ventral funiculus or at the surface of the spinal cord. Brain Res Rev. 2002;40(1-3):309-16. https://doi.org/10.1016/s0165-0173(02)00213-8.
-
19.
Alluin O, Wittmann C, Marqueste T, Chabas JF, Garcia S, Lavaut MN, et al. Functional recovery after peripheral nerve injury and implantation of a collagen guide. Biomaterials. 2009;30(3):363-73. [PubMed ID: 18929405]. https://doi.org/10.1016/j.biomaterials.2008.09.043.
-
20.
Mohammad J, Shenaq J, Rabinovsky E, Shenaq S. Modulation of peripheral nerve regeneration: a tissue-engineering approach. The role of amnion tube nerve conduit across a 1-centimeter nerve gap. Plast Reconstr Surg. 2000;105(2):660-6. [PubMed ID: 10697174]. https://doi.org/10.1097/00006534-200002000-00027.
-
21.
Battiston B, Geuna S, Ferrero M, Tos P. Nerve repair by means of tubulization: literature review and personal clinical experience comparing biological and synthetic conduits for sensory nerve repair. Microsurgery. 2005;25(4):258-67. [PubMed ID: 15934044]. https://doi.org/10.1002/micr.20127.
-
22.
Johnson EO, Soucacos PN. Nerve repair: Experimental and clinical evaluation of biodegradable artificial nerve guides. Injury. 2008;39 Suppl 3:S30-6. [PubMed ID: 18722612]. https://doi.org/10.1016/j.injury.2008.05.018.
-
23.
Wrede L. Bridging nerve defects by means of stitches and vein segments. Deutsches Med Wochenschrift. 1909;35:1125-34.
-
24.
Maeda T, Mackinnon SE, Best TJ, Evans PJ, Hunter DA, Rajiv Midha RT. Regeneration across ‘stepping-stone’ nerve grafts. Brain Research. 1993;618(2):196-202. https://doi.org/10.1016/0006-8993(93)91266-u.
-
25.
Lundborg G, Dahlin LB, Danielsen NP, Hansson HA, Larsson K. Reorganization and orientation of regenerating nerve fibres, perineurium, and epineurium in preformed mesothelial tubes - an experimental study on the sciatic nerve of rats. J Neurosci Res. 1981;6(3):265-81. [PubMed ID: 7299843]. https://doi.org/10.1002/jnr.490060302.
-
26.
Lundborg G, Dahlin LB, Danielsen N, Gelberman RH, Longo FM, Powell HC, et al. Nerve regeneration in silicone chambers: Influence of gap length and of distal stump components. Exp Neurol. 1982;76(2):361-75. https://doi.org/10.1016/0014-4886(82)90215-1.
-
27.
Sabongi RG, Fernandes M, Dos Santos JB. Peripheral nerve regeneration with conduits: use of vein tubes. Neural Regen Res. 2015;10(4):529-33. [PubMed ID: 26170802]. [PubMed Central ID: PMC4424734]. https://doi.org/10.4103/1673-5374.155428.
-
28.
Chiu DT, Janecka I, Krizek TJ, Wolff M, Lovelace RE. Autogenous vein graft as a conduit for nerve regeneration. Surgery. 1982;91(2):226-33. [PubMed ID: 7058501].
-
29.
Kong JM, Zhong SZ, Bo S, Zhu SX. Experimental study of bridging the peripheral nerve gap with skeletal muscle. Microsurgery. 1986;7(4):183-9. [PubMed ID: 3796271]. https://doi.org/10.1002/micr.1920070411.
-
30.
Mackinnon SE, Dellon AL. A study of nerve regeneration across synthetic (Maxon) and biologic (collagen) nerve conduits for nerve gaps up to 5 cm in the primate. J Reconstr Microsurg. 1990;6(2):117-21. [PubMed ID: 2352218]. https://doi.org/10.1055/s-2007-1006810.
-
31.
Rath S, Green CJ. Selectivity of distal reinnervation of regenerating mixed motor and sensory nerve fibres across muscle grafts in rats. British Journal of Plastic Surgery. 1991;44(3):215-8. https://doi.org/10.1016/0007-1226(91)90130-c.
-
32.
Ansselin AD, Fink T, Davey DF. Peripheral nerve regeneration through nerve guides seeded with adult Schwann cells. Neuropathol Appl Neurobiol. 1997;23(5):387-98. [PubMed ID: 9364464].
-
33.
O'Neill AC, Randolph MA, Bujold KE, Kochevar IE, Redmond RW, Winograd JM. Preparation and integration of human amnion nerve conduits using a light-activated technique. Plast Reconstr Surg. 2009;124(2):428-37. [PubMed ID: 19644257]. https://doi.org/10.1097/PRS.0b013e3181af010c.
-
34.
Mackinnon SE, Hudson AR, Falk RE, Hunter DA. The nerve allograft response--an experimental model in the rat. Ann Plast Surg. 1985;14(4):334-9. [PubMed ID: 3873203]. https://doi.org/10.1097/00000637-198504000-00006.
-
35.
Seckel BR, Chiu TH, Nyilas E, Sidman RL. Nerve regeneration through synthetic biodegradable nerve guides: regulation by the target organ. Plast Reconstr Surg. 1984;74(2):173-81. [PubMed ID: 6379712]. https://doi.org/10.1097/00006534-198408000-00001.
-
36.
William Bora F, Bednar JM, Osterman A, Brown MJ, Sumner AJ. Prosthetic nerve grafts: A resorbable tube as an alternative to autogenous nerve grafting. J Hand Surg. 1987;12(5):685-92. https://doi.org/10.1016/s0363-5023(87)80048-5.
-
37.
Fields R, Le Beau JM, Longo FM, Ellisman MH. Nerve regeneration through artificial tubular implants. Prog Neurobiol. 1989;33(2):87-134. https://doi.org/10.1016/0301-0082(89)90036-1.
-
38.
Heath CA, Rutkowski GE. The development of bioartificial nerve grafts for peripheral-nerve regeneration. Trends Biotechnol. 1998;16(4):163-8. https://doi.org/10.1016/s0167-7799(97)01165-7.
-
39.
Davis GE, Blaker SN, Engvall E, Varon S, Manthorpe M, Gage FH. Human amnion membrane serves as a substratum for growing axons in vitro and in vivo. Science. 1987;236(4805):1106-9. [PubMed ID: 3576223]. https://doi.org/10.1126/science.3576223.
-
40.
Akle CA, Welsh KI, Adinolfi M, Leibowitz S, McColl I. Immunogenicity of human amniotic epithelial cells after transplantation into volunteers. Lancet. 1981;318(8254):1003-5. https://doi.org/10.1016/s0140-6736(81)91212-5.
-
41.
Gray KJ, Shenaq SM, Engelmann UH, Fishman IJ, Jeraj K, Spira M. Use of human amnion for microvascular interpositional grafts. Plast Reconstr Surg. 1987;79(5):778-85. [PubMed ID: 3575523]. https://doi.org/10.1097/00006534-198705000-00018.
-
42.
Kim SS, Sohn SK, Lee KY, Lee MJ, Roh MS, Kim CH. Use of human amniotic membrane wrap in reducing perineural adhesions in a rabbit model of ulnar nerve neurorrhaphy. J Hand Surg Eur Vol. 2010;35(3):214-9. [PubMed ID: 20007422]. https://doi.org/10.1177/1753193409352410.
-
43.
Meng H, Li M, You F, Du J, Luo Z. Assessment of processed human amniotic membrane as a protective barrier in rat model of sciatic nerve injury. Neurosci Lett. 2011;496(1):48-53. [PubMed ID: 21511004]. https://doi.org/10.1016/j.neulet.2011.03.090.
-
44.
Mligiliche N, Endo K, Okamoto K, Fujimoto E, Ide C. Extracellular matrix of human amnion manufactured into tubes as conduits for peripheral nerve regeneration. J Biomed Mater Res. 2002;63(5):591-600. [PubMed ID: 12209905]. https://doi.org/10.1002/jbm.10349.
-
45.
Riccio M, Marchesini A, Pugliese P, De Francesco F. Nerve repair and regeneration: Biological tubulization limits and future perspectives. J Cell Physiol. 2019;234(4):3362-75. [PubMed ID: 30206940]. https://doi.org/10.1002/jcp.27299.
-
46.
Kitada M. Mesenchymal cell populations: development of the induction systems for Schwann cells and neuronal cells and finding the unique stem cell population. Anat Sci Int. 2012;87(1):24-44. [PubMed ID: 22237924]. https://doi.org/10.1007/s12565-011-0128-4.
-
47.
Scuteri A, Miloso M, Foudah D, Orciani M, Cavaletti G, Tredici G. Mesenchymal stem cells neuronal differentiation ability: a real perspective for nervous system repair? Curr Stem Cell Res Ther. 2011;6(2):82-92. [PubMed ID: 21190538]. https://doi.org/10.2174/157488811795495486.
-
48.
De Francesco F, Ricci G, D'Andrea F, Nicoletti GF, Ferraro GA. Human adipose stem cells: From bench to bedside. Tissue Eng Part B Rev. 2015;21(6):572-84. [PubMed ID: 25953464]. https://doi.org/10.1089/ten.TEB.2014.0608.
-
49.
Nicoletti GF, De Francesco F, D'Andrea F, Ferraro GA. Methods and procedures in adipose stem cells: state of the art and perspective for translation medicine. J Cell Physiol. 2015;230(3):489-95. [PubMed ID: 25294367]. https://doi.org/10.1002/jcp.24837.
-
50.
Jang S, Cho HH, Cho YB, Park JS, Jeong HS. Functional neural differentiation of human adipose tissue-derived stem cells using bFGF and forskolin. BMC Cell Biol. 2010;11:25. [PubMed ID: 20398362]. [PubMed Central ID: PMC2867791]. https://doi.org/10.1186/1471-2121-11-25.
-
51.
Aghayan HR, Goodarzi P, Baradaran-Rafii A, Larijani B, Moradabadi L, Rahim F, et al. Bacterial contamination of amniotic membrane in a tissue bank from Iran. Cell Tissue Bank. 2013;14(3):401-6. [PubMed ID: 23095908]. https://doi.org/10.1007/s10561-012-9345-x.
-
52.
Aghayan HR, Goodarzi P, Arjmand B. GMP-compliant human adipose tissue-derived mesenchymal stem cells for cellular therapy. Methods Mol Biol. 2015;1283:93-107. [PubMed ID: 25138723]. https://doi.org/10.1007/7651_2014_112.
-
53.
Liang HS, Liang P, Xu Y, Wu JN, Liang T, Xu XP, et al. Denuded human amniotic membrane seeding bone marrow stromal cells as an effective composite matrix stimulates axonal outgrowth of rat neural cortical cells in vitro. Acta Neurochir (Wien). 2009;151(9):1113-20. [PubMed ID: 19404575]. https://doi.org/10.1007/s00701-009-0322-5.
-
54.
Terenghi G. Peripheral nerve regeneration and neurotrophic factors. J Anat. 1999;194 ( Pt 1):1-14. [PubMed ID: 10227662]. [PubMed Central ID: PMC1467889]. https://doi.org/10.1046/j.1469-7580.1999.19410001.x.
-
55.
Banerjee A, Nurnberger S, Hennerbichler S, Riedl S, Schuh CM, Hacobian A, et al. In toto differentiation of human amniotic membrane towards the Schwann cell lineage. Cell Tissue Bank. 2014;15(2):227-39. [PubMed ID: 24166477]. https://doi.org/10.1007/s10561-013-9401-1.
-
56.
Kingham PJ, Kalbermatten DF, Mahay D, Armstrong SJ, Wiberg M, Terenghi G. Adipose-derived stem cells differentiate into a Schwann cell phenotype and promote neurite outgrowth in vitro. Exp Neurol. 2007;207(2):267-74. [PubMed ID: 17761164]. https://doi.org/10.1016/j.expneurol.2007.06.029.
-
57.
Faroni A, Terenghi G, Reid AJ. Adipose-derived stem cells and nerve regeneration: Promises and pitfalls. Int Rev Neurobiol. 2013;108:121-36. [PubMed ID: 24083433]. https://doi.org/10.1016/B978-0-12-410499-0.00005-8.
-
58.
Walocko FM, Khouri RJ, Urbanchek MG, Levi B, Cederna PS. The potential roles for adipose tissue in peripheral nerve regeneration. Microsurgery. 2016;36(1):81-8. [PubMed ID: 26773850]. [PubMed Central ID: PMC5089369]. https://doi.org/10.1002/micr.22480.
-
59.
Forootan SK, Yazdani Abde A, Akbari H, Fatemi MJ, Pedram MS, Mosavi SJ. [Comparison between the effect of amniotic membrane and vein conduit in nerve repair-animal study]. Iran J Surg. 2011;18(4). Persian.
-
60.
Sadraie SH, Parivar K, Arabi F, Moattari M, Kaka G, Mansouri K. Study of transected sciatic nerve repair by amniotic membrane with betamethasone in adult albino wistar rats. Arch Iran Med. 2016;19(9):612-7. [PubMed ID: 27631175].