Abstract
Background:
The primary method for addressing dental problems traditionally involves the removal and replacement of damaged tissue with synthetic substitutes. However, these materials often lack the physical and chemical properties of natural teeth and are prone to mechanical failures. Recent advancements in tissue engineering have highlighted an alternative approach that allows for the regeneration of teeth using scaffolds.Objectives:
This study explored the viability of human gingival fibroblast cells in a scaffold composed of polyhydroxybutyrate, chitosan, and bioglass nanoparticles (PHB/chitosan/nBG).Methods:
This study is experimental and was conducted in a laboratory setting. Scaffolds of PHB, PHB/chitosan, and PHB/chitosan/nBG were previously prepared from PHB polymer, chitosan, and bioglass using the electrospinning method. Human gingival fibroblast cells (C165) were acquired from the Pasteur Institute and cultured. Twenty thousand cells were seeded onto each scaffold and into the wells of a 24-well plate for periods of 3, 5, and 7 days. Subsequently, cell viability and proliferation were assessed using the MTT assay method, with each experiment repeated three times.Results:
Cell viability and proliferation on the PHB/chitosan/nBG scaffold demonstrated a significant increase compared to the other scaffolds (P ≤ 0.01).Conclusions:
The PHB/chitosan/nBG scaffold was found to be non-toxic to human gingival fibroblast cells and exhibited good biocompatibility.Keywords
Hydroxybutyrate Bioglass Chitosan Fibroblast Tissue Engineering
1. Background
Preserving teeth is a paramount objective in dentistry (1). Techniques such as root canal treatment have been developed to achieve this goal (2). However, there are cases where severely damaged teeth cannot be preserved and must be extracted. In these instances, alternatives like dental implants and prostheses can replace natural teeth (3, 4). Recently, advances in cell therapy and biomaterial engineering have provided opportunities to repair damaged tissues and address various medical conditions. In tissue engineering, porous materials are carefully designed to act as extracellular matrices or scaffolds, which support cell growth. These scaffolds must possess certain characteristics, including non-toxicity, biocompatibility, degradability, non-immunogenicity, ease of preparation, suitable physical and mechanical properties, stability, and a three-dimensional (3D) structure that mimics natural tissue (5).
Many materials, including both natural and synthetic polymers, have been used to create tissue engineering scaffolds. Among these, polyhydroxyalkanoates, which are biodegradable polymers, have been utilized alone or in combination for medical applications such as tissue repair and controlled drug delivery. These polyesters are synthesized by microorganisms through various methods (6-8). The polyhydroxyalkanoate family includes PHB, noted for its longer degradation time compared to polymers in the polyalphahydroxy acids group (9). Polyhydroxybutyrate is known for its excellent biocompatibility with different cell types. However, it has limitations, such as hydrophobicity and a slow degradation rate, which can limit its use in producing tissue engineering scaffolds (10, 11). One way to overcome these drawbacks is by integrating natural polymers.
Chitin and chitosan polymers are natural amino polysaccharides characterized by their unique structures and distinctive properties (12). Chitosan is known for its biocompatibility, biodegradability, and non-toxic nature, and can be degraded by various enzymes (13). Its positive surface charge promotes cell growth, and its hydrophilic nature facilitates cell adhesion, proliferation, and differentiation (14, 15). However, chitosan is notably weak and mechanically unstable (16). To improve its mechanical and biological characteristics, researchers have combined chitosan with other biocompatible materials such as hydroxyapatite, bioglass, collagen, or gelatin (17). Bioactive glasses are extensively studied for their ability to bond with surrounding tissues, primarily due to the formation of a hydroxyapatite carbonate layer on their surface, which shows greater biocompatibility than calcium phosphates (18).
In a pioneering study, a 3D scaffold made of polyhydroxybutyrate/poly (hydroxybutyrate-co-hydroxyvalerate) (PHBV) was produced using the electrospinning technique. The resulting fibrous scaffolds displayed increased hydrophilicity and tensile strength compared to films made from the same material and provided a conducive substrate for the proliferation of human osteoblast cells and mouse fibroblasts (L929) (19, 20). In another study, a PHB/poly (L-lactide-co-ε-caprolactone) (PLCL) composite scaffold was fabricated through electrospinning, showing enhanced hydrophilicity and tensile strength relative to a PHB scaffold alone. Cell testing indicated strong adhesion and proliferation of olfactory ensheathing cells within the scaffold (21). Separately, Veleirinho et al. developed a PHBV/chitosan scaffold by electrospinning for skin regeneration. This scaffold demonstrated excellent wound healing performance in mice, supported by fibroblast adhesion, cell viability, and proliferation as assessed with L929 cells (22).
2. Objectives
Given the need for a biocompatible scaffold in dental injury restoration, this study explored the viability of human gingival fibroblast cells in a PHB/chitosan/nanobioglass nanocomposite scaffold prepared via the electrospinning method.
3. Methods
This study is classified as experimental laboratory research.
3.1. Preparation of Scaffolding
The preparation of the scaffolding involved dissolving PHB polymer in trifluoroacetic acid solvent at 40°C for 20 minutes. Subsequently, chitosan was added to the solution at a concentration of 15% by weight, along with bioactive glass nanoparticles at 10% by weight. The mixture was then stirred for 60 minutes, followed by immediate electrospinning. The electrospinning process was conducted at an electric potential of 17 kV, with a distance of 14 cm between the spinneret and the collector. This process produced scaffolds with a thickness of 0.3 mm and a diameter of 8 mm. These scaffolds were subsequently sterilized using 70° ethanol and UV radiation (19). FTIR analysis, along with tests for water absorption and degradability of the scaffolds, were performed in a previous study (19).
3.2. Cell Survival and Proliferation in Porous Scaffolds
Gingival fibroblast cells (C165), obtained from the Pasteur Institute, were used to assess cell survival and proliferation within porous scaffolds. Initially, the thawed cells were cultured in DMEM (Dulbecco's Modified Eagle Medium, produced by BIO-IDEA Iran), supplemented with 10% Fetal Bovine Serum (Invitrogen CO.) and 1% penicillin-streptomycin (Sigma). The scaffolds tested (PHB, PHB/Chitosan, and PHB/Chitosan/nBG) were placed in the wells of a 24-well plate, and 20 000 cells were then transferred onto each scaffold using 50 microliters of culture medium. After a 20-minute incubation period to allow for cell adhesion, 1 mL of culture medium containing serum was added to each well. The plates were then placed in an incubator set at 37 degrees Celsius, with an atmosphere of 5% CO2 and 95% relative humidity (19).
3.3. Methyl Thiazol Tetrazolium Assay
To evaluate the viability and proliferation of gingival fibroblast cells on the scaffolds, an Methyl Thiazol Tetrazolium assay (MTT assay) was performed on days 3, 5, and 7. After removing the culture medium and washing with PBS, approximately 400 µL of fresh culture medium and 40 µL of MTT solution (5 mg/mL) were added to each well and incubated for 4 hours. Subsequently, the culture medium was carefully removed, and 400 µL of Dimethyl Sulfoxide, Sigma (DMSO) was added to each well and left in the dark for 2 hours at room temperature. After mixing well by pipetting, 100 µL of the solution was transferred to a well of a 96-well plate, and the optical density (OD) of the samples was measured at a wavelength of 540 nm using an ELISA reader (19).
4. Results
The results of the MTT assay indicated that cell viability on the third day in the PHB/chitosan/nBG scaffold was significantly different from the other two scaffolds (P ≤ 0.01), with fibroblast proliferation being higher in this scaffold. On the fifth day, cell survival and proliferation in the PHB/chitosan/nBG scaffold (about 86%) showed a significant increase compared to the other two groups (P ≤ 0.01). In contrast, the PHB/Chitosan scaffold experienced a decrease in cell viability (about 28%). On the seventh day, the PHB/chitosan/nBG scaffold continued to exhibit the highest cell survival, while the PHB/Chitosan scaffold showed the lowest (P ≤ 0.01) (Figure 1).
Results of the viability and proliferation of fibroblast cells show a significant increase in viability in the PHB/chitosan/nBG scaffold compared to the PHB and PHB/chitosan scaffolds. A, PHB scaffold; B, PHB/chitosan scaffold; C, PHB/chitosan/nBG scaffold; * PHB/chi and PHB/chi/nBG compared with PHB; ** PHB/chi and PHB/chi/nBG compared with PHB; *** PHB/chi and PHB/chi/nBG compared with PHB; ## PHB/chi/nBG compared with PHB/chi; ### PHB/chi/nBG compared with PHB/chi.
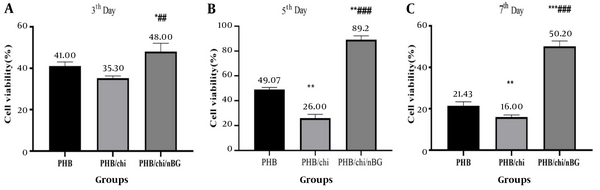
5. Discussion
One critical factor influencing tissue engineering is the selection of scaffold types. In practice, scaffolds used in tissue engineering feature a three-dimensional structure that provides mechanical support to cells. These scaffolds must be biocompatible, display favorable mechanical properties, and be capable of withstanding forces and responding to stressors similarly to the target tissue. Additionally, they should maintain a controlled degradation rate, offer appropriate pore sizes and morphology for cell growth, facilitate sterilization, support grafting, and be suitable for tissue engineering applications (5). Numerous organisms produce PHB, which has demonstrated high biocompatibility and low toxicity across various cell types (23).
However, despite its advantages, PHB has limitations, including hydrophilicity and a slow degradation rate, which restrict its utility in producing tissue engineering scaffolds (24). The results from our previous study indicate that the hydrophilicity and degradation rate of PHB scaffolds are lower compared to those of chitosan and bioglass scaffolds. One study showed that approximately 18% of PHB scaffolds decompose within six months under physiological conditions (25). Consequently, this research incorporated chitosan, a natural polymer, with PHB. The results from our previous study suggest that water absorption and degradation are influenced by the polymer's hydrophilicity and the presence of carboxyl and hydroxyl groups in its structure. These factors contribute to an increase in the degradation rate over time and the formation of shorter polymer chains with acidic ends.
Semi-crystalline polymers like PHB, when exposed to an aqueous solution, initially allow water to penetrate the amorphous regions of the crystal, leading to random chain scission due to ester bond hydrolysis. Over time, hydrolysis progresses to the crystalline regions (19). Our previous study observed that adding bioglass and chitosan, including hydrophilic nanoparticles to the scaffold, increased water absorption and weight loss over time.
5.1. Cell Viability Assessment
The assessment reveals that the scaffold containing bioglass and chitosan exhibits excellent biocompatibility and provides a suitable platform for cell growth and proliferation. Therefore, it can be asserted that the growth and proliferation of fibroblast cells in the scaffolds are influenced by chemical properties, specifically hydrophilicity, and structural properties such as porosity and fiber size. The more optimal these properties are, the more conducive they are to cell attachment and growth.
Comparatively, the scaffold incorporating bioglass nanoparticles displays greater viability than the scaffold without nanoparticles. The evidence supports the conclusion that nanoparticles facilitate cell growth and adhesion. In a study conducted by Yang et al. in 2009, a PCL/gelatin/nanohydroxyapatite composite scaffold was created to evaluate the functionality of dental pulp stem cells (DPSC). Following in vitro and in vivo assessments, they determined that this scaffold is a suitable substrate for the proliferation of DPSCs and their differentiation into odontoblast-like cells (26).
Furthermore, Ramier et al. electrospun a PHB/Gelatin/nHA nanocomposite scaffold, which enhanced the growth and proliferation of human mesenchymal cells (hMSC) while promoting bone formation properties (27).
5.2. Conclusions
The results of this research show that the PHB/chitosan/nBG scaffold has no toxic effects on human gingival fibroblast cells and exhibits good biocompatibility.
Acknowledgements
References
-
1.
Cosyn J, Thoma DS, Hammerle CH, De Bruyn H. Esthetic assessments in implant dentistry: objective and subjective criteria for clinicians and patients. Periodontol 2000. 2017;73(1):193-202. [PubMed ID: 28000279]. https://doi.org/10.1111/prd.12163.
-
2.
Chybowski EA, Glickman GN, Patel Y, Fleury A, Solomon E, He J. Clinical Outcome of Non-Surgical Root Canal Treatment Using a Single-cone Technique with Endosequence Bioceramic Sealer: A Retrospective Analysis. J Endod. 2018;44(6):941-5. [PubMed ID: 29606401]. https://doi.org/10.1016/j.joen.2018.02.019.
-
3.
Pjetursson BE, Heimisdottir K. Dental implants - are they better than natural teeth? Eur J Oral Sci. 2018;126 Suppl 1:81-7. [PubMed ID: 30178552]. https://doi.org/10.1111/eos.12543.
-
4.
Chopra A, Sivaraman K, Narayan AI, Balakrishnan D. Etiology and classification of food impaction around implants and implant-retained prosthesis. Clin Implant Dent Relat Res. 2019;21(2):391-7. [PubMed ID: 30702201]. https://doi.org/10.1111/cid.12716.
-
5.
Kwon SG, Kwon YW, Lee TW, Park GT, Kim JH. Recent advances in stem cell therapeutics and tissue engineering strategies. Biomater Res. 2018;22:36. [PubMed ID: 30598836]. [PubMed Central ID: PMC6299977]. https://doi.org/10.1186/s40824-018-0148-4.
-
6.
Kirillova A, Yeazel TR, Asheghali D, Petersen SR, Dort S, Gall K, et al. Fabrication of Biomedical Scaffolds Using Biodegradable Polymers. Chem Rev. 2021;121(18):11238-304. [PubMed ID: 33856196]. https://doi.org/10.1021/acs.chemrev.0c01200.
-
7.
Chen GQ, Wu Q. The application of polyhydroxyalkanoates as tissue engineering materials. Biomater. 2005;26(33):6565-78. [PubMed ID: 15946738]. https://doi.org/10.1016/j.biomaterials.2005.04.036.
-
8.
Williams SF, Martin DP, Horowitz DM, Peoples OP. PHA applications: addressing the price performance issue: I. Tissue engineering. Int J Biol Macromol. 1999;25(1-3):111-21. [PubMed ID: 10416657]. https://doi.org/10.1016/s0141-8130(99)00022-7.
-
9.
Misra SK, Valappil SP, Roy I, Boccaccini AR. Polyhydroxyalkanoate (PHA)/inorganic phase composites for tissue engineering applications. Biomacromolecules. 2006;7(8):2249-58. [PubMed ID: 16903667]. https://doi.org/10.1021/bm060317c.
-
10.
Zhang F, King MW. Biodegradable Polymers as the Pivotal Player in the Design of Tissue Engineering Scaffolds. Adv Healthc Mater. 2020;9(13). e1901358. [PubMed ID: 32424996]. https://doi.org/10.1002/adhm.201901358.
-
11.
Jacquel N, Lo C, Wei Y, Wu H, Wang SS. Isolation and purification of bacterial poly(3-hydroxyalkanoates). Biochem Eng J. 2008;39(1):15-27. https://doi.org/10.1016/j.bej.2007.11.029.
-
12.
Singh R, Shitiz K, Singh A. Chitin and chitosan: biopolymers for wound management. Int Wound J. 2017;14(6):1276-89. [PubMed ID: 28799228]. [PubMed Central ID: PMC7949833]. https://doi.org/10.1111/iwj.12797.
-
13.
Matica A, Menghiu G, Ostafe V. Biodegradability of chitosan based products. New Frontiers Chem. 2017;26(1).
-
14.
Ahsan SM, Thomas M, Reddy KK, Sooraparaju SG, Asthana A, Bhatnagar I. Chitosan as biomaterial in drug delivery and tissue engineering. Int J Biol Macromol. 2018;110:97-109. [PubMed ID: 28866015]. https://doi.org/10.1016/j.ijbiomac.2017.08.140.
-
15.
Croisier F, Jérôme C. Chitosan-based biomaterials for tissue engineering. Eur Polym J. 2013;49(4):780-92. https://doi.org/10.1016/j.eurpolymj.2012.12.009.
-
16.
Jung YJ, An BJ, Choi HW, Kim HS, Lee YH. Preparation and characterization of chitosan/cellulose acetate blend film. J Korean Soc Dye Finish. 2007;19:10-7.
-
17.
Islam MM, Shahruzzaman M, Biswas S, Nurus Sakib M, Rashid TU. Chitosan based bioactive materials in tissue engineering applications-A review. Bioact Mater. 2020;5(1):164-83. [PubMed ID: 32083230]. [PubMed Central ID: PMC7016353]. https://doi.org/10.1016/j.bioactmat.2020.01.012.
-
18.
Montazeri M, Karbasi S, Foroughi MR, Monshi A, Ebrahimi-Kahrizsangi R. Evaluation of mechanical property and bioactivity of nano-bioglass 45S5 scaffold coated with poly-3-hydroxybutyrate. J Mater Sci Mater Med. 2015;26(2):62. [PubMed ID: 25631260]. https://doi.org/10.1007/s10856-014-5369-z.
-
19.
Foroughi MR, Karbasi S, Khoroushi M, Khademi AA. Polyhydroxybutyrate/chitosan/bioglass nanocomposite as a novel electrospun scaffold: fabrication and characterization. J Porous Mater. 2017;24(6):1447-60. https://doi.org/10.1007/s10934-017-0385-2.
-
20.
Sombatmankhong K, Suwantong O, Waleetorncheepsawat S, Supaphol P. Electrospun fiber mats of poly(3‐hydroxybutyrate), poly(3‐hydroxybutyrate‐co‐3‐hydroxyvalerate), and their blends. J Polym Sci Part B: Polym Phys. 2006;44(19):2923-33. https://doi.org/10.1002/polb.20915.
-
21.
Daranarong D, Chan RT, Wanandy NS, Molloy R, Punyodom W, Foster LJ. Electrospun polyhydroxybutyrate and poly(L-lactide-co-epsilon-caprolactone) composites as nanofibrous scaffolds. Biomed Res Int. 2014;2014:741408. [PubMed ID: 24900983]. [PubMed Central ID: PMC4034502]. https://doi.org/10.1155/2014/741408.
-
22.
Veleirinho B, Coelho DS, Dias PF, Maraschin M, Ribeiro-do-Valle RM, Lopes-da-Silva JA. Nanofibrous poly(3-hydroxybutyrate-co-3-hydroxyvalerate)/chitosan scaffolds for skin regeneration. Int J Biol Macromol. 2012;51(4):343-50. [PubMed ID: 22652216]. https://doi.org/10.1016/j.ijbiomac.2012.05.023.
-
23.
Mohapatra S, Mohanty D, Mohapatra S, Sharma S, Dikshit S, Kohli I, et al. Biomedical Application of Polymeric Biomaterial: Polyhydroxybutyrate. Bioresource Utilization and Management. Cambridge, MA: Apple Academic Press; 2021. p. 111-24. https://doi.org/10.1201/9781003057826-6.
-
24.
Amini S, Salehi H, Setayeshmehr M, Ghorbani M. Natural and synthetic polymeric scaffolds used in peripheral nerve tissue engineering: Advantages and disadvantages. Polyme Adv Technol. 2021;32(6):2267-89. https://doi.org/10.1002/pat.5263.
-
25.
Abd-Elmeguid A, Yu DC, Kline LW, Moqbel R, Vliagoftis H. Dentin matrix protein-1 activates dental pulp fibroblasts. J Endod. 2012;38(1):75-80. [PubMed ID: 22152625]. https://doi.org/10.1016/j.joen.2011.10.005.
-
26.
Yang X, Yang F, Walboomers XF, Bian Z, Fan M, Jansen JA. The performance of dental pulp stem cells on nanofibrous PCL/gelatin/nHA scaffolds. J Biomed Mater Res A. 2010;93(1):247-57. [PubMed ID: 19557787]. https://doi.org/10.1002/jbm.a.32535.
-
27.
Ramier J, Grande D, Bouderlique T, Stoilova O, Manolova N, Rashkov I, et al. From design of bio-based biocomposite electrospun scaffolds to osteogenic differentiation of human mesenchymal stromal cells. J Mater Sci Mater Med. 2014;25(6):1563-75. [PubMed ID: 24584668]. https://doi.org/10.1007/s10856-014-5174-8.