Abstract
Background:
Gold nanoparticles (AuNPs), a new cancer treatment, have few side effects.Objectives:
This study aimed to investigate the effect of AuNPs on oxidative stress in HCT-116 colon cancer cells.Methods:
The viability of HCT-116 cells was evaluated using the 3-(4,5-dimethylthiazol-2,5-diphenyl) tetrazolium bromide (MTT) assay after 24 and 48 hours of incubation with different concentrations of AuNPs (0 - 50 μg/mL). The state of oxidative stress, total antioxidant capacity (TAC), total oxidative status (TOS), reactive oxygen species (ROS) levels, and the activity of antioxidant enzymes, including superoxide dismutase (SOD), glutathione peroxidase (GPX), and catalase (CAT), were determined by colorimetric and fluorometric methods.Results:
Oxidative stress parameters and ROS were significantly increased in cells treated with 25 and 50 μg/mL concentrations of AuNPs during 24 and 48 hours of incubation compared to control cells (P < 0.001). In contrast, the level of TAC and the activity of antioxidant enzymes SOD, GPX, and CAT were significantly decreased in the treated groups compared to the untreated cells after 48 hours of incubation (P < 0.05).Conclusions:
This study showed that AuNPs affect HCT-116 cancer cells by increasing ROS and oxidative stress while reducing the activity of antioxidant enzymes.Keywords
Colon Cancer HCT-116 Cells Oxidative Stress Gold Nanoparticles (AuNPs) Cell Viability
1. Background
One of the most common gastrointestinal cancers globally is colorectal cancer (1). Colon cancer is the third most common cancer and is the fourth leading cause of death worldwide (2). Approximately one million new cases of colorectal cancer are diagnosed each year, and nearly half a million people die from this disease (3). Colorectal cancer has two types: Hereditary (familial) and sporadic (4). It has been shown that approximately 80% of cases are sporadic, while the remaining 20% are hereditary (4). Common treatments for colon cancer include chemotherapy, radiation therapy, surgery, or a combination of these methods, which are usually associated with side effects (5). These side effects have led researchers to design new methods, including nanoparticle-mediated therapy, to effectively treat cancer complications (6).
Nanoparticles are less than one hundred nanometers in size and possess reliable reactivity, structure, and size, allowing them to have wide applications in medicine and drug delivery, biotechnology, the food industry, electronics, mechanical industries, manufacturing, and materials production (7, 8). Recently, gold nanoparticles (AuNPs) have received much attention in nanotechnology and biomedicine due to their unique properties (9). AuNPs are favored in biomedical studies for their ability to cross the blood-brain barrier, interact with DNA, and produce cytotoxic and genotoxic effects. AuNPs have great potential for cancer treatment, including stimulating mitochondrial respiratory chain damage, oxidative stress, and inhibition of adenosine triphosphate (ATP) synthesis, leading to DNA damage and apoptosis (10, 11). The mechanism of AuNP toxicity has been described as protein denaturation, membrane damage, DNA damage, and immune response (12).
Oxidative stress can contribute to the cytotoxicity of AuNPs through the production of reactive oxygen species (ROS) in various human cancer cells (13). It has also been shown that some nanoparticles activate inflammatory cells such as macrophages and neutrophils, leading to increased ROS production (14). Many nanoparticles exert their effectiveness by increasing the production of ROS, thus leading to oxidative stress (15). Endogenous antioxidants (EnAO) are enzymes or cofactors that eliminate ROS. Several combined protection systems are present in the antioxidant defense system expressed in mammals. Catalase (CAT), glutathione peroxidase (GPX), and superoxide dismutase (SOD) are enzymes that play a vital role in the EnAO specificity of biological systems against free radicals (16). Several studies have reported the anticancer properties of AuNPs on cancer cells such as bladder cancer T24 cells (17), HepG2 cells (18), and colon carcinoma HT-29 cell line (19). Since free radicals play an important role in causing oxidative stress and various diseases, the effect of AuNPs on oxidative stress status in the HCT-116 cell line in human colon cancer was evaluated.
2. Methods
2.1. Chemicals
Fetal Bovine Serum (FBS) from Azmayar, Bioidea, Iran; Dulbecco’s Modified Eagle’s Medium (DMEM) from Kiazist, Iran; Phosphate Buffered Saline (PBS) from DNAbiotech, Kiazist, Iran; and Dimethyl Sulfoxide (DMSO) and 3-(4,5-dimethylthiazol-2-yl)-2,5-diphenyl-2H-tetrazolium bromide (MTT) from Sigma, USA, were obtained.
2.2. Gold Nanoparticles (Au Nanoparticles)
Gold nanoparticles as citrate-stabilized AuNPs with approximate dimensions of 20 nm and a concentration of 100 μg/mL were purchased from the Iranian Nanomaterials Company (NANOSANY, Mashhad, Iran). The characteristics of the gold nanoparticles are: Au in deionized water, purity: 99.95%, APS: 18 - 22 nm, SSA: 14 m²/g, bulk density: ∼1.25 g/cm³, true density: 19.32 g/cm³, melting point: 10°C (nano-powder far below this point), boiling point: 2966°C, and morphology: Spherical.
2.3. Cell Culture
HCT-116 cells were purchased from the Pasteur Institute of Iran. The cells were cultured using high-glucose DMEM, 10% FBS, and 1% antibiotic penicillin-streptomycin in an incubator at 37°C, 5% CO2, 95% relative humidity, and pH 7.40. The cells were harvested at 70 - 80% confluency, detached from the flask using trypsin-EDTA, and centrifuged at 1300 rpm for 7 minutes. The cell residue was then suspended in a culture medium. The viability of the cells was determined using trypan blue dye with a hemocytometer slide and an optical invert microscope (Nikon Eclipse TS 100). Cells with more than 90% viability were used for the subsequent tests.
2.4. MTT Assay
The MTT assay was conducted to assess the cytotoxicity of AuNPs. Tetrazolium salt is converted to an insoluble purple formazan compound in DMSO solvent. The absorption of the purple dye was measured at 570 nm using a microplate enzyme-linked immunoassay (ELISA) reader. The cells were detached from the flask by adding trypsin, and cell count was performed using a hemocytometer slide. Then, 5 × 104 cells/well were seeded in 96-well plates and kept for 24 hours, followed by treatment with AuNPs at dosages ranging from 1.55 to 50 µg/mL. The treated cells were incubated for 24 and 48 hours, and their viability was assessed by the MTT method. After incubation, the media of the wells were replaced with 10 μL of MTT solution. The microplates were incubated in the dark for 3 - 4 hours, then the cell medium was completely displaced, and 200 μL of DMSO solution was added to each well. After 15 minutes of incubation at 37°C, the absorption of the microplates was read at 570 nm using an ELISA reader (RT-2100 C Microplate Reader, China). The experiments were run in triplicate. The cell viability was calculated using the following formula:
Viability (%) = [(OD of the AuNP treated cells − OD of the blanks) × 100] / (OD of the untreated cells − OD of the blanks).
2.5. Preparation of Cell Lysate for Determination of Oxidative Stress
To assay the activity of antioxidant enzymes CAT, GPX, and SOD after 24 and 48 hours of treatment of HCT-116 cells with AuNPs, the cell culture medium was removed, and 1 mL trypsin was added to each flask and incubated at 37°C for 3 minutes. After detaching the cells from the bottom of the flask, 2 mL media were added to the flask to counteract the effects of trypsin. The resulting suspension was then transferred to a falcon tube and centrifuged at 1500 rpm for 5 minutes, and the supernatant was discarded. The cells were washed with cold PBS buffer to remove trypsin. The cell pellet was dissolved in a cold PBS buffer containing a protease inhibitor cocktail and subjected to three freeze-thaw cycles. After 15 minutes, the suspension was centrifuged at 12000 rpm at 4°C, and the supernatant was separated and kept at -80°C.
2.6. Measurement of Reactive Oxygen Species (ROS) by Fluorimeter
In this method, 25000 HCT-116 cells were seeded in each well. After 24 hours of incubation at 37°C and 5% CO2, cells were treated with different concentrations of AuNPs. After incubation for 24 and 48 hours, the medium was removed, and the cells were washed with the medium. Then, 100 μL of reagent dichlorodihydrofluorescein diacetate (DCFDA) was added to each well and incubated for 45 minutes at room temperature. Immediately after incubation, the fluorescence was measured at Ex/Em = 485/528 nm (Multi-Mode Reader, Bio-Tek Instruments, Winooski, USA). DCFDA oxidized working solution and H2O2 were used as a positive control. All steps were performed away from light.
2.7. Total Antioxidant Capacity (TAC) Level Measurement
Total Antioxidant Capacity (TAC) was measured manually using the Ferric Reducing Antioxidant Power (FRAP) method. In this method, the antioxidant compounds in the samples reduce ferric (Fe3+) to ferrous (Fe2+), producing a blue color. The UV spectrum was documented using a spectrophotometer (One/Once, ScientificThermo, UV-Vis Spectrophotometer) at 593 nm.
2.8. Total Oxidant Status (TOS) Level Measurement
Total Oxidant Status (TOS) levels in the serum were determined by the oxidation of ferrous (Fe2+) to ferric (Fe3+) and the measurement of ferric ions using xylose orange at 546 nm.
2.9. Oxidative Stress Index (OSI)
The Oxidative Stress Index (OSI) indicates the state of oxidative stress in the cell, which is obtained by dividing TOS by TAC: OSI (Oxidative Stress Index) = TOS / TAC.
2.10. Measurement of Superoxide Dismutase (SOD) Enzyme Activity
Superoxide dismutase (SOD) enzyme activity was measured according to the kit protocol (Kiazist, Iran). SOD is an antioxidant enzyme that neutralizes superoxide ions, effectively preventing oxidative stress. The kit measures SOD enzyme activity using both colorimetric and fluorometric methods at 520 - 570 nm.
2.11. Measurement of Catalase (CAT) Enzyme Activity
The activity of catalase (CAT) in the cells was measured using the kit protocol (Kiazist, Iran). In this experiment, CAT exhibits peroxidase activity in the presence of methanol, which is then stopped by an inhibitor. The produced formaldehyde reacts with purpald to produce a purple color, and its absorbance was observed at 540 nm.
2.12. Measurement of Glutathione Peroxidase (GPX) Activity
The activity of glutathione peroxidase (GPX) in cell lysates was measured using the kit (Kiazist, Iran). The amount of dye production is inversely related to enzymatic activity. By adding more reduced glutathione (GSH), the enzyme converts it to its oxidized form (GSSG), and the amount of remaining GSH produces a yellow color with maximum absorbance at 340 nm.
2.13. Statistical Analysis
One-way ANOVA (analysis of variance) and Tukey's test, using GraphPad Prism 8.0.3, were employed to evaluate the differences between the groups. The results were reported as mean (±) standard deviation (SD). All experiments were performed in triplicate, and the statistical significance level was considered P < 0.05.
3. Results
3.1. Effects of Gold Nanoparticles (AuNPs) on the Viability of HCT-116 Cells
The effect of different concentrations (1.55, 3.1, 6.25, 12.5, 25, and 50 μg/mL) of AuNPs on HCT-116 colon cancer cells was evaluated using the MTT assay at 24 and 48 hours. According to Figure 1, cell viability decreased after 48 hours with increasing nanoparticle concentrations compared to the control group. Specifically, concentrations of 12.5, 25, and 50 μg/mL of AuNPs reduced the viability of HCT-116 cells to 68.41 ± 1.04%, 51.43 ± 1.44%, and 30.89 ± 0.84%, respectively.
Similarly, the MTT test results after 24 hours of incubation with 12.5, 25, and 50 μg/mL of AuNPs showed reduced viability of HCT-116 cells to 90.59 ± 1.50%, 83.30 ± 3.05%, and 59.98 ± 2.81%, respectively, compared to the untreated cells. These results indicate a decrease in the survival of HCT-116 cells in the presence of AuNPs, which is largely time- and concentration-dependent.
Effect of gold nanoparticles on viability of HCT-116 colon cancer cells. The results are shown as mean ± SD of three individual experiments (** P < 0.01, *** P < 0.001) compared with the control group (N = 3).
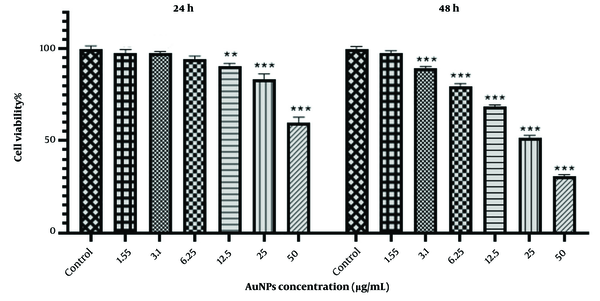
As shown in Figure 2, there is a significant difference (P < 0.001) between the 24 and 48-hour incubation periods. With increasing concentration and incubation time of AuNPs, we observed more inhibitory effects. Using the nonlinear regression method in Prism GraphPad version 8, the inhibitory concentration (IC50) obtained from the MTT test was estimated to be 24.90 μg/mL at 48 hours.
Comparison of the effects of different concentrations of gold nanoparticles (AuNPs) on the viability of 116-HCT colon cancer cell at 24h and 48h
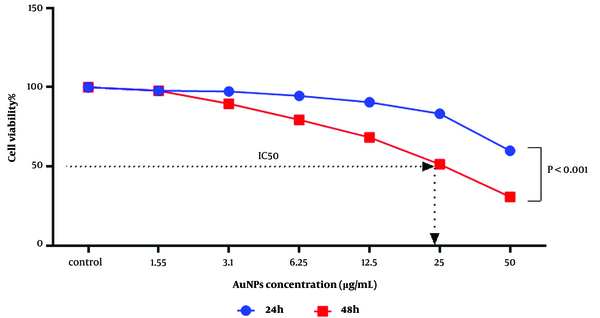
3.2. Effects of Gold Nanoparticles (AuNPs) on Morphological Changes of HCT-116 Cells
As shown in Figure 3C and D, after 48 hours of treatment with AuNPs at concentrations of 25 and 50 μg/mL, morphological changes were observed, including the deformation of adherent epithelial-shaped cells into round and suspended cells. These changes were more pronounced than in the control group and the group treated with a concentration of 12.5 μg/mL of AuNPs.
Morphology of HCT-116 colon cancer cells after treatment with gold nanoparticles for 48h. A, Control group (untreated cells) cells; B, HCT-116 cells treated with a concentration of 12.5 μg / mL; C, HCT-116 cells treated with a concentration of 25 μg/mL; D, HCT-116 cells treated with a concentration of 50 μg / mL.
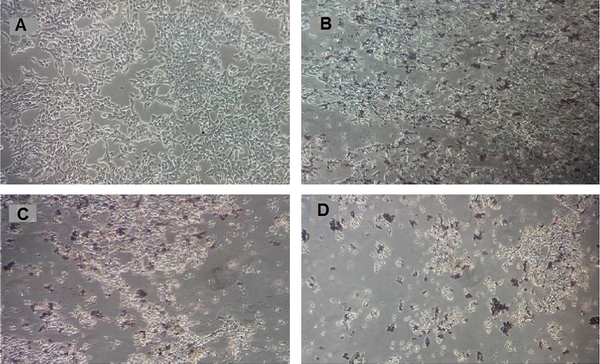
3.3. Effects of Gold Nanoparticles (AuNPs) on Reactive Oxygen Species (ROS) Production
Reactive Oxygen Species (ROS) levels were measured using a fluorimeter at 24 and 48 hours. As shown in Figure 4, treatment with AuNPs significantly increased ROS production in HCT-116 cells (P < 0.001). At 24 hours, ROS levels increased at a concentration of 50 μg/mL compared with those in untreated control cells. Additionally, there was a significant increase in ROS levels at a concentration of 50 μg/mL compared to cells treated with a concentration of 12.5 μg/mL. However, in cells treated with concentrations of 12.5 and 25 μg/mL, there was no significant difference compared to the control group. At 48 hours, the ROS level increased significantly in cells treated with 25 and 50 μg/mL concentrations compared to the control group. Treatment with a concentration of 12.5 μg/mL of AuNPs also showed an increase in ROS levels compared to the control group. Moreover, the increase in ROS levels at concentrations of 25 and 50 μg/mL compared to cells treated with 12.5 μg/mL was significant.
Effect of gold nanoparticles on reactive oxygen species (ROS) production in HCT-116 colon cancer cells. The results are shown as mean ± SD of three individual experiments ** P < 0.01, *** P < 0.001 compared to the control group (N = 3), ## P < 0.01, ### P < 0.001 compared with other groups.
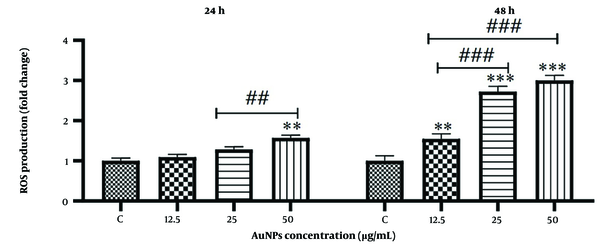
3.4. Effects of Gold Nanoparticles (AuNPs) on Oxidative Stress Indices
The results of oxidative stress indicators, including TAC, TOS, and OSI, in cells treated with different concentrations of AuNPs at 24 and 48 hours compared to the control group are shown in Figure 5. The data from this study indicate that, with the treatment of HCT-116 cells by AuNPs, the levels of ROS, TOS, and OSI increased with both higher concentrations and longer incubation times. Additionally, treatment with AuNPs significantly (P < 0.001) reduced TAC levels in all treated groups compared to the control group.
Effect of gold nanoparticles on oxidative stress in HCT-116 colon cancer cells. The results are shown as mean ± SD of three individual experiments, ** P < 0.01, *** P < 0.001 compared to the control group (N = 3), ### P < 0.001, ## P < 0.01 Compared with other groups. A, Total oxidative state (TOS); B, Total antioxidant capacity (TAC); and C, Oxidative stress index (OSI)
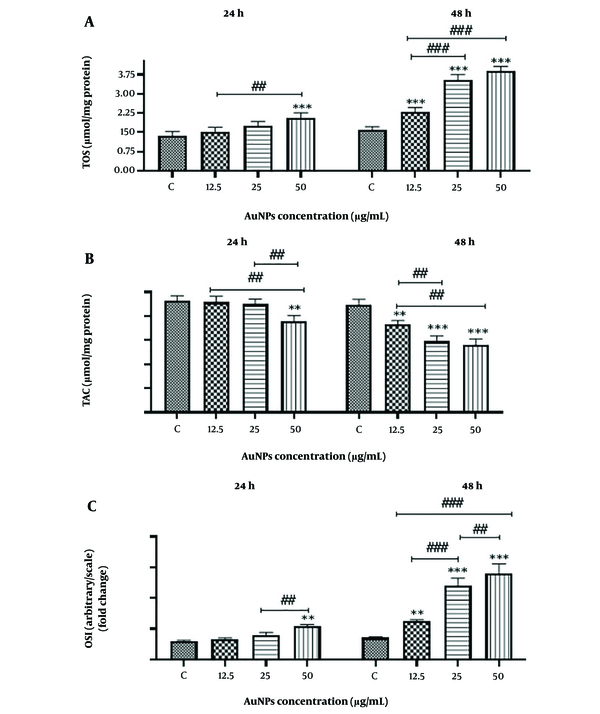
3.5. Effects of Gold Nanoparticles (AuNPs) on the Activity of Antioxidant Enzymes
As shown in Figure 6, the activity of antioxidant enzymes in HCT-116 cells decreases in a concentration- and time-dependent manner due to AuNP treatment. The decrease in HCT-116 cell viability is associated with increased oxidative stress, consistent with the results obtained from the MTT assay.
Effect of gold nanoparticles on anti-oxidant enzymes in HCT-116 colon cancer cells. The results are shown as mean ± SD of three individual experiments** P < 0.01, *** P < 0.001 compared with the control group (N = 3), ### P < 0.001, ## P < 0.01 Compared with other groups. A, Catalase (CAT); B, Glutathione peroxidase (GPX); C, Superoxide dismutase (SOD)
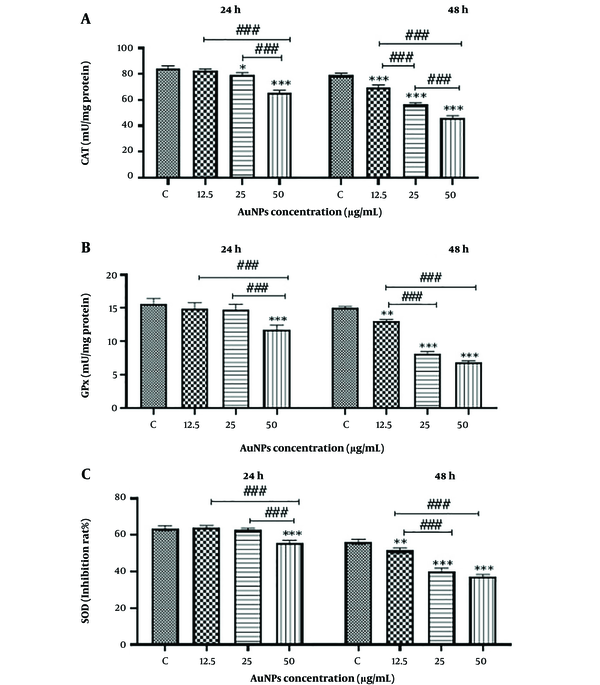
4. Discussion
An imbalance between oxidants and antioxidants, in favor of oxidants, potentially leads to oxidative stress (20). The study of oxidative stress is medically necessary because it is associated with many human diseases, including cancer (21). In this study, the potential of AuNPs in increasing ROS production was evaluated by the fluorometric method. This study showed a concentration-dependent increase in ROS production in cells treated with AuNPs. It was observed that with increasing concentration and incubation time of HCT-116 cells, ROS production increased. Consistent with our results, the study by Enea et al. in 2020, which was performed on renal cell carcinoma treated with AuNPs, also observed increased intracellular ROS (22). Additionally, a study by Martinez-Torres et al. in 2018 showed that AuNPs induced cell death in cervical and breast cancer cell lines due to oxidative stress through ROS production (23). These findings suggest that these nanoparticles can cause ROS accumulation in cells, leading to cell death due to oxidative stress.
In a study by Akbarzadeh Khiavi et al. in 2020, the effect of AuNPs on the SW-480 colon cancer cell line was investigated, showing an increase in ROS production dependent on the concentration (24). Similarly, a study by Chakraborty et al. in 2020 found that MG63 cells treated with AuNPs showed an increase in ROS production with increased mitochondrial depolarization (24, 25).
To further evaluate the state of oxidative stress in HCT-116 cells, TAC and TOS levels were determined in the study groups. The results showed a significant increase in TOS levels after 48 hours of incubation of cells with AuNPs. In addition, the TAC level in treated cells was significantly reduced, which was consistent with the results of the MTT and ROS assays. Metin Budak in 2019 analyzed the effects of different nanoparticles on the induction of oxidative stress in the HT-29 colon cancer cell line, and their results showed that the performance of nanoparticles in causing oxidative stress after 48 hours is higher than after 24 hours (26). After measuring the enzyme activities of ROS, TAC, and TOS, results showed that HCT-116 cancer cells in the presence of dose- and time-dependent AuNPs exhibit increased ROS production, elevated TOS levels, and decreased TAC levels. This indicates an increase in the activity of oxygen-free radicals. One of the characteristics of cancer cells is their ability to adapt and create a redox balance by increasing ROS levels, which provides resistance to oxidative stress inducers compared to normal cells. As a result, cancer cells continue to proliferate and develop resistance to oxidative stress inducers. Due to the inherent capacity of nanoparticles to promote ROS production, this increase can be partially neutralized by the antioxidant system (27).
To investigate the effect of AuNPs on antioxidant indices, the activity of SOD, GPX, and CAT enzymes was evaluated. The activity of these enzymes in the presence of AuNPs showed a significant decrease compared to untreated cells, with increasing concentration and incubation time. Similarly, in a study by Nellore et al. in 2012, the incubation of PC-12 cell lines with AuNPs increased MDA and LDH levels, decreased non-protein thiol and glutathione (GSH) levels, and reduced the activity of antioxidant enzymes SOD, CAT, and glutathione S-transferase (28). Furthermore, in the study by Domsa et al. in 2020, the activity of SOD and CAT in the Caco-2 cell line was measured under the influence of AuNPs. It was observed that CAT activity was significantly reduced compared to the control group, and the antioxidant activity of superoxide dismutase was also considerably reduced with AuNP treatment. In a study by Zhang et al. in 2020 on the SH-SY5Y cell line, an increase in oxidative stress and a decrease in the activity of key antioxidant enzymes, including glutathione peroxidase (GSH-Px), SOD, and CAT, were observed after prolonged exposure to coated AuNPs (29, 30). Additionally, the importance of antioxidant enzyme activity in cancer was highlighted in a study by Mateo et al. in 2014, which demonstrated that oxidative stress helps AuNPs increase cytotoxicity. In this study, two cell lines (HL-60 and HepG2) were exposed to AuNPs of different sizes (30, 50, and 90 nm) (12). In both cell lines, glutathione levels were reduced by the different sizes of AuNPs after 72 hours. However, the enzyme activity of SOD decreased only in the HepG2 cell line, and ROS activity increased in both cell lines. In contrast, a 2015 study by Kumar et al. investigated the effect of biologically synthesized AuNPs on the A431 cell line. Oxidative activity and antioxidant enzyme activity were measured using the FRAP method (31). In this study, exposure to AuNPs increased the activity of antioxidant enzymes and TAC, leading to apoptosis in the A431 cell line. The differences observed in the results of oxidative stress parameter measurements between the mentioned study and the present study could be due to physicochemical differences in the nanoparticles or different responses of the cell lines used.
Cancer cells generally have a higher antioxidant capacity and require small molecules that can reduce antioxidant defense capacity by increasing ROS levels (32, 33). The results of the present study indicate that AuNPs can increase TOS levels and decrease TAC, in addition to increasing ROS production. This, in turn, affects the activity of antioxidant enzymes, including SOD, GPX, and CAT, in HCT-116 cancer cells. As the concentration of AuNPs increases, enzyme activity decreases further, leading to apoptosis or programmed cell death. Therefore, AuNPs have significant potential to be used as an anticancer therapy for colon cancer. Further studies are needed to investigate the pathways involved in AuNPs toxicity in colon cancer cell lines.
4.1. Conclusions
Our results showed that gold nanoparticles (AuNPs) can reduce the viability and induce apoptosis in HCT116 colon cancer cells in a time- and concentration-dependent manner. Specifically, AuNPs at a concentration of 50 µg/mL induced significant oxidative stress compared to the control group in the HCT116 cell line. This study aimed to assess the importance of AuNPs in increasing oxidative stress, reducing the activity of antioxidant enzymes, and decreasing cell survival in colon cancer cells.
Our findings illustrate that AuNPs can increase TOS, enhance ROS production, reduce the activity of antioxidant enzymes such as CAT, SOD, and GPX, and decrease TAC in this cell line. It is suggested that the anticancer effects of AuNPs and the oxidative stress induced by AuNPs be investigated in other cancer cell lines. Additionally, other factors involved in oxidative stress, such as glutathione peroxidase, xanthine oxidase, glutathione reductase, H2O2, superoxide anion, etc., as well as the toxicity of AuNPs in in vivo studies, should be considered for further investigation.
References
-
1.
Pordanjani SR, Baeradeh N, Lotfi MH, Pourmohammadi B. Epidemiology of colorectal cancer: incidence, mortality, survival rates and risk factors. Razi J Med Sci. 2016.
-
2.
Lee J, Shin A, Oh JH, Kim J. The relationship between nut intake and risk of colorectal cancer: a case control study. Nutr J. 2018;17(1):37. [PubMed ID: 29514652]. [PubMed Central ID: PMC5840774]. https://doi.org/10.1186/s12937-018-0345-y.
-
3.
Boyle P, Leon ME. Epidemiology of colorectal cancer. Br Med Bull. 2002;64:1-25. [PubMed ID: 12421722]. https://doi.org/10.1093/bmb/64.1.1.
-
4.
Mao R, Krautscheid P, Graham RP, Ganguly A, Shankar S, Ferber M, et al. Genetic testing for inherited colorectal cancer and polyposis, 2021 revision: a technical standard of the American College of Medical Genetics and Genomics (ACMG). Genet Med. 2021;23(10):1807-17. [PubMed ID: 34140662]. https://doi.org/10.1038/s41436-021-01207-9.
-
5.
Qudayr A, Kabel AM, AbdElmaaboud MA, Alghamdi WY, Alghorabi A. Colorectal Cancer: New Perspectives. J Cancer Res Treat. 2018;6(3):80-3.
-
6.
O'Neal DP, Hirsch LR, Halas NJ, Payne JD, West JL. Photo-thermal tumor ablation in mice using near infrared-absorbing nanoparticles. Cancer Lett. 2004;209(2):171-6. [PubMed ID: 15159019]. https://doi.org/10.1016/j.canlet.2004.02.004.
-
7.
Duran N, Silveira CP, Duran M, Martinez DS. Silver nanoparticle protein corona and toxicity: a mini-review. J Nanobiotechnology. 2015;13:55. [PubMed ID: 26337542]. [PubMed Central ID: PMC4559354]. https://doi.org/10.1186/s12951-015-0114-4.
-
8.
Khan I, Saeed K, Khan I. Nanoparticles: Properties, applications and toxicities. J Arabian Chemistry. 2019;12(7):908-31. https://doi.org/10.1016/j.arabjc.2017.05.011.
-
9.
Jeyaraj M, Arun R, Sathishkumar G, MubarakAli D, Rajesh M, Sivanandhan G, et al. An evidence on G2/M arrest, DNA damage and caspase mediated apoptotic effect of biosynthesized gold nanoparticles on human cervical carcinoma cells (HeLa). J Materials Research Bulletin. 2014;52:15-24. https://doi.org/10.1016/j.materresbull.2013.12.060.
-
10.
Memon AR, Schroder P. Implications of metal accumulation mechanisms to phytoremediation. Environ Sci Pollut Res Int. 2009;16(2):162-75. [PubMed ID: 19067014]. https://doi.org/10.1007/s11356-008-0079-z.
-
11.
Goldsbrough P. Metal tolerance in plants: the role of phytochelatins and metallothioneins. Phytoremediation of contaminated soil and water. Crc Press; 2020. p. 221-33. https://doi.org/10.1201/9780367803148-12.
-
12.
Mateo D, Morales P, Avalos A, Haza AI. Oxidative stress contributes to gold nanoparticle-induced cytotoxicity in human tumor cells. Toxicol Mech Methods. 2014;24(3):161-72. [PubMed ID: 24274460]. https://doi.org/10.3109/15376516.2013.869783.
-
13.
Narayanan KB, Sakthivel N. Green synthesis of biogenic metal nanoparticles by terrestrial and aquatic phototrophic and heterotrophic eukaryotes and biocompatible agents. Adv Colloid Interface Sci. 2011;169(2):59-79. [PubMed ID: 21981929]. https://doi.org/10.1016/j.cis.2011.08.004.
-
14.
Manke A, Wang L, Rojanasakul Y. Mechanisms of nanoparticle-induced oxidative stress and toxicity. Biomed Res Int. 2013;2013:942916. [PubMed ID: 24027766]. [PubMed Central ID: PMC3762079]. https://doi.org/10.1155/2013/942916.
-
15.
Styskal J, Van Remmen H, Richardson A, Salmon AB. Oxidative stress and diabetes: what can we learn about insulin resistance from antioxidant mutant mouse models? Free Radic Biol Med. 2012;52(1):46-58. [PubMed ID: 22056908]. [PubMed Central ID: PMC3249484]. https://doi.org/10.1016/j.freeradbiomed.2011.10.441.
-
16.
Fuloria S, Subramaniyan V, Karupiah S, Kumari U, Sathasivam K, Meenakshi DU, et al. Comprehensive Review of Methodology to Detect Reactive Oxygen Species (ROS) in Mammalian Species and Establish Its Relationship with Antioxidants and Cancer. Antioxidants (Basel). 2021;10(1). [PubMed ID: 33477494]. [PubMed Central ID: PMC7831054]. https://doi.org/10.3390/antiox10010128.
-
17.
Wu T, Duan X, Hu C, Wu C, Chen X, Huang J, et al. Synthesis and characterization of gold nanoparticles from Abies spectabilis extract and its anticancer activity on bladder cancer T24 cells. Artif Cells Nanomed Biotechnol. 2019;47(1):512-23. [PubMed ID: 30810403]. https://doi.org/10.1080/21691401.2018.1560305.
-
18.
Nandhini JT, Ezhilarasan D, Rajeshkumar S. An ecofriendly synthesized gold nanoparticles induces cytotoxicity via apoptosis in HepG2 cells. Environ Toxicol. 2021;36(1):24-32. [PubMed ID: 32794643]. https://doi.org/10.1002/tox.23007.
-
19.
Rezaee Z, Yadollahpour A, Bayati V, Negad Dehbashi F. Gold nanoparticles and electroporation impose both separate and synergistic radiosensitizing effects in HT-29 tumor cells: an in vitro study. Int J Nanomedicine. 2017;12:1431-9. [PubMed ID: 28260889]. [PubMed Central ID: PMC5327907]. https://doi.org/10.2147/IJN.S128996.
-
20.
Sies H. Oxidative stress: oxidants and antioxidants. Exp Physiol. 1997;82(2):291-5. [PubMed ID: 9129943]. https://doi.org/10.1113/expphysiol.1997.sp004024.
-
21.
Sosa V, Moline T, Somoza R, Paciucci R, Kondoh H, L. Leonart ME. Oxidative stress and cancer: an overview. Ageing Res Rev. 2013;12(1):376-90. [PubMed ID: 23123177]. https://doi.org/10.1016/j.arr.2012.10.004.
-
22.
Enea M, Pereira E, de Almeida MP, Araujo AM, de Lourdes Bastos M, Carmo H. Gold Nanoparticles Induce Oxidative Stress and Apoptosis in Human Kidney Cells. Nanomaterials (Basel). 2020;10(5). [PubMed ID: 32455923]. [PubMed Central ID: PMC7279525]. https://doi.org/10.3390/nano10050995.
-
23.
Martinez-Torres AC, Zarate-Trivino DG, Lorenzo-Anota HY, Avila-Avila A, Rodriguez-Abrego C, Rodriguez-Padilla C. Chitosan gold nanoparticles induce cell death in HeLa and MCF-7 cells through reactive oxygen species production. Int J Nanomedicine. 2018;13:3235-50. [PubMed ID: 29910612]. [PubMed Central ID: PMC5987788]. https://doi.org/10.2147/IJN.S165289.
-
24.
Akbarzadeh Khiavi M, Safary A, Barar J, Farzi-Khajeh H, Barzegari A, Mousavi R, et al. PEGylated gold nanoparticles-ribonuclease induced oxidative stress and apoptosis in colorectal cancer cells. Bioimpacts. 2020;10(1):27-36. [PubMed ID: 31988854]. [PubMed Central ID: PMC6977588]. https://doi.org/10.15171/bi.2020.04.
-
25.
Chakraborty A, Das A, Raha S, Barui A. Size-dependent apoptotic activity of gold nanoparticles on osteosarcoma cells correlated with SERS signal. J Photochem Photobiol B. 2020;203:111778. [PubMed ID: 31931389]. https://doi.org/10.1016/j.jphotobiol.2020.111778.
-
26.
Budak M. Nanoparticles Induce Oxidative Stress in HT-29 Colon Adenocarcinoma Cell Line After 24 and 48 Hour Exposure. International Journal of Genetics and Genomics. 2019:110. https://doi.org/10.11648/j.ijgg.20190704.14.
-
27.
Panaretakis T, Shabalina IG, Grander D, Shoshan MC, DePierre JW. Reactive oxygen species and mitochondria mediate the induction of apoptosis in human hepatoma HepG2 cells by the rodent peroxisome proliferator and hepatocarcinogen, perfluorooctanoic acid. Toxicol Appl Pharmacol. 2001;173(1):56-64. [PubMed ID: 11350215]. https://doi.org/10.1006/taap.2001.9159.
-
28.
Nellore J, Pauline C, Amarnath K, Shilpa PN. Antioxidant effect of gold nanoparticles synthesised from curcuma longa restrains 1-methyl-2-phenyl pyridinium ion induced stress in PC12 cells. J Nanoneuroscience. 2012;2(1):63-74. https://doi.org/10.1166/jns.2012.1018.
-
29.
Domsa EM, Filip GA, Olteanu D, Baldea I, Clichici S, Muresan A, et al. Gold nanoparticles phytoreduced with Cornus mas extract mitigate some of gliadin effects on Caco-2 cells. J Physiol Pharmacol. 2020;71(2).
-
30.
Zhang X, Guo X, Kang X, Yang H, Guo W, Guan L, et al. Surface Functionalization of Pegylated Gold Nanoparticles with Antioxidants Suppresses Nanoparticle-Induced Oxidative Stress and Neurotoxicity. Chem Res Toxicol. 2020;33(5):1195-205. [PubMed ID: 32125152]. https://doi.org/10.1021/acs.chemrestox.9b00368.
-
31.
Kumar V, Hussain PR, Chatterjee S, Variyar PS. Evaluation of in vitro antioxidant activity and characterization of phenolic compounds of bottle gourd towards the green synthesis of gold nanoparticles and its bio-efficacy. J Int J Food Nutr Saf. 2015;6(3):125-49.
-
32.
Kim S, Yang HY, Lee HJ, Ju J. In Vitro Antioxidant and Anti-Colon Cancer Activities of Sesamum indicum L. Leaf Extract and Its Major Component, Pedaliin. Foods. 2021;10(6). [PubMed ID: 34072150]. [PubMed Central ID: PMC8228213]. https://doi.org/10.3390/foods10061216.
-
33.
Gorrini C, Harris IS, Mak TW. Modulation of oxidative stress as an anticancer strategy. Nat Rev Drug Discov. 2013;12(12):931-47. [PubMed ID: 24287781]. https://doi.org/10.1038/nrd4002.