Abstract
Context:
One of the most common aggressive and primary brain tumors is glioma. The majority of diagnoses are referred to high-grade malignant glioblastoma, which carries the worst prognosis. Still, treatment of brain tumors remains a big challenge for clinicians. This study was designed to investigate the efficacy of gene therapy in the treatment of brain cancer.Methods:
Studies use genes as a therapeutic agent in brain cancer treatment even alone or in combination with other treatment methods. Full-text papers, which met the inclusion criteria, were independently assessed by two reviewers. Disagreements were resolved by consultation with a third reviewer.Results:
Statistical analysis showed that 50% of the papers used a virus, 36% used polymers, and 14% used cells as carriers to transfect the genes as a therapeutic agent in brain tumor models. Data showed that the estimated size of the brain tumor was reduced by using co-treatment of the gene with one of the conventional therapies.Conclusions:
According to the results, co-treatment of the gene with conventional therapies could be more effective than the monotherapy methods.Keywords
Brain Cancer Glioblastoma Gene Therapy Combination Therapy Neoplasms
1. Context
One of the most common aggressive and primary brain tumors is glioma as a result of its tendency to penetrate the brain tissue and fast proliferation (1). Gliomas can be divided into two types, namely low grade (II and III) and high grade (IV) based upon histopathology diagnosis according to the classification of the World Health Organization (WHO) (2). Between these grades, the majority of diagnoses referred to high-grade malignant glioblastoma, which carries the worst prognosis. Factors that are important in the prognosis of Glioma depend on the histological grade of the tumor, the patient’s age, and Karnofsky's Performance Score (KPS) (2). These tumors possess the ability to invade surrounding tissue extensively; so, curative resection may become impossible (3). Some of the general treatments for gliomas are a combination of surgery, radiation therapy, and systemic chemotherapy (4). Malignant glioblastoma has very poor outcomes with just less than 5% of patients surviving in 5 years post-diagnosis even with the best current treatments (2). In light of these poor results, and to increase the extent of novel therapies, there is significant room for improvement and innovation in the area of managing the treatment. An application of nanotechnology which holds great promise for revolutionizing medical treatments, faster diagnosis, drug delivery, tissue regeneration, and imaging is nanomedicine (5). Nanotechnology helps deliver the drug to the targeted tissue across the blood-brain barrier (BBB), control releasing the drug, and avoid degradation processes (6). The other performance of this system is toxicity reduction of peripheral organs and biodegradability. Using nucleic acids as drugs are called gene therapy (7). Delivery or vector systems are needed to deliver the therapeutic gene (s) to the tumor mass (8). Viral and non-viral vectors are two subdivisions of delivery systems (9). The efficiency of the vector that can selectively deliver a gene to target cells with minimal toxicity is a success in gene therapy. An efficient method in gene transfection is using viral carriers although there would be a risk of not being biologically safe. Therefore, using non-viral delivery systems became an attractive alternative for humans, which received increasing attention in gene therapy. These vectors can overcome major issues of viral delivery toxicity. The non-viral carriers are easy to prepare and exhibit low cytotoxicity and they can also carry large DNA fragments. However, finding a carrier will (1) load genetic materials, (2) pass the material through cellular barriers without an immune response, (3) release it into the cell nucleus, and (4) allow the visualization of this entire process without degrading the materials remains a great challenge (7). The short-lived nature of the therapeutic DNA and the multifactorial nature of many disorders are also affecting the effectiveness of gene therapy (10). Nanoparticles were first developed in about 1970 and initially designed as carriers for vaccines and anticancer drugs (11). The first important step in the strategy of drug targeting was to reduce the uptake of the nanoparticles by the reticuloendothelial system (RES) to enhance tumor uptake (12). In this regard, coating nanoparticles (NPs) and nano-capsules with hydrophilic substances such as polypropylene block copolymers (poloxamers), chitosan, polyvinyl alcohol, and PEG have a clear benefit with minimal non-specific interaction with other proteins (12). While surface charges, molecular weight, amphiphilicity, shape, and the structure of NPs are very important in gene transfection efficiency of polymer-based NPs (10). Several cationic polymers such as poly L-lysine (PLL), polyethyleneimine (PEI), poly (amidoamine) (PAMAM), and chitosan are used as important vectors for gene delivery (10). For these reasons, a systematic review of the literature was carried out to evaluate the conventional cancer therapy methods compared to novel ones (e.g., gene therapy), especially on brain tumors.
2. Research Question
Could gene therapy be effective in brain cancer therapy according to clinical treatment and experimental studies?
3. Study Objectives
(1) Comparing the cancer therapy methods in the treatment of Glioblastoma and introducing the best one.
(2) Conducting statistical analysis of primary studies, if appropriate, to gain a more precise estimate of the effectiveness of different strategies in managing Glioblastoma.
(3) Critically appraise existing evidence and identify gaps in the literature to provide future research directions.
4. Methods
This systematic review was proposed to compare the conventional and gene therapy methods for brain cancer.
4.1. Protocol and Registration
This study was registered under Prospero registration number: CRD42016052141. The development and reporting of this systematic review were under the guidance of the Preferred Reporting Items for Systematic Review and Meta-Analysis-Protocols (PRISMA-P) statement (13).
4.2. Eligibility Criteria
In this review, studies with different neural diseases or additional treatments were excluded. Conference abstracts, letters, and other studies without detailed data were also excluded. Studies published between 2007 and 2018 in the English language that investigated therapies for brain cancers including gene therapy and conventional methods were eligible with no restriction on the brain cancer type or grade. Statistical analysis results were limited to cancer therapy methods for brain tumors worked both in vitro and in vivo. Reviews and clinical studies were also excluded.
The interventions included in the study are
(1) Cancer therapy methods e.g., surgery, chemotherapy, radiotherapy, and gene delivery methods on Glioblastoma.
(2) All kinds of studies e.g., in vitro, in vivo, and clinical.
(3) Comparing conventional therapies with gene therapy methods.
4.3. Data Sources
Meeting abstracts and relevant full publications were identified by electronic searching of 5 online databases, including MEDLINE, EMBASE, Web of Science, ScienceDirect, and the COCHRANE Library (from their inception to two months before submission).
4.4. Search Strategy
Searches were conducted, using both text words and exploded Medical Subject Heading (MeSH) terms: (cancers OR neoplasms) AND (therapy OR therapeutics) AND (gene delivery OR gene transfer techniques OR biolistic) AND (brain tumor OR brain neoplasms) AND (chemotherapy) AND (surgery) AND (radiotherapy) AND (combination therapy). The search strategy was specified for each dataset based on its rules. For the PubMed dataset, it was Gene delivery AND Brain cancer AND Combination therapy, for EMBASE dataset, it was 'gene delivery' in All fields AND 'brain cancer' AND 'combination therapy' as Emtree-exploded, for COCHRANE Library, it was gene delivery AND brain cancer AND combination therapy in All text, for Web of Science library, gene delivery AND brain cancer AND combination therapy in title/keyword/abstract were used and for ScienceDirect dataset, the following strategy was used in Expert search; “gene delivery” AND “brain cancer” AND “combination therapy” in all sources. References were managed in EndNote (version X7) and duplicates were removed and, then, exported to Microsoft Excel (version 2010) spreadsheet to complete the search process.
4.5. Study Selection and Data Collection
Two independent reviewers selected the included citations based on the title and abstract of the papers. Two reviewers independently assessed the full-text papers with the inclusion criteria and disagreements were resolved by consulting with a third reviewer. The minimum follow-up time for included studies was 18 months.
Data were collected from full-text papers based on the method mentioned in the Cochrane Consumers Handbook (14). The following information was recorded for each study: Basic characteristics (author, title, year of publication, and study design), intervention data (method, dose, and time of gene transduction), and outcome data (follow-up time).
4.6. Data Extraction and Management
A data extraction form was developed and the relevant data were extracted from the included studies. The method of the treatment (in vitro studies, in vivo studies, and statistical studies), the gold standard, and outcome variables (safety and survival probability) were extracted as detailed information in the results.
5. Results
5.1. Included Trials
The search was conducted in November 2018 and 80 records were obtained through PubMed, using Gene delivery AND Brain cancer AND Combination therapy, 58 records through EMBASE using 'gene delivery' in All fields AND 'brain cancer' AND 'combination therapy' as Emtree-exploded, 29 records through COCHRANE Library using gene delivery AND brain cancer AND combination therapy in All text, 77 records through Web of Science library using gene delivery AND brain cancer AND combination therapy in title/keyword/abstract and 27 records through ScienceDirect using the following strategy in Expert search; “gene delivery” AND “brain cancer” AND “combination therapy” in all sources (Figure 1).
Literature search and inclusion on process
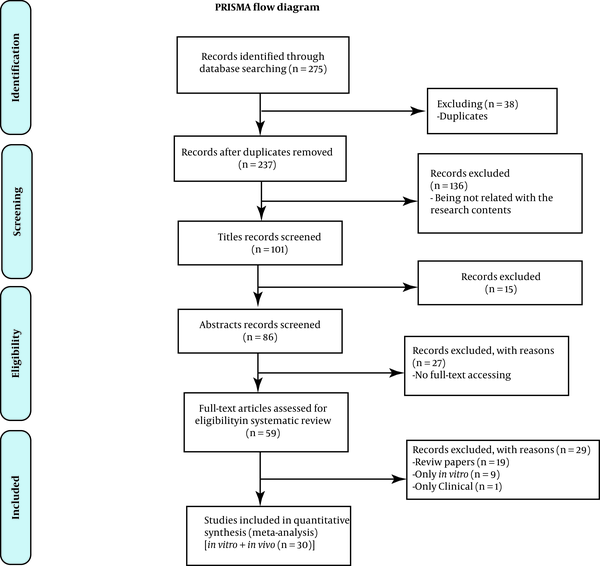
5.2. Methodological Quality
First of all, studies were categorized according to the subject, type, methodology, and result parameters that were summarized in Appendix 1 in Supplementary File. Subjects were grouped in virus, cell, and polymer-mediated gene therapy, chemotherapy, and multiple-delivery. Generally, there were 59 papers, 18 of which were virus-mediated, 8 cell-mediated, and 22 in polymer-mediated gene therapy. Eight of the papers used multiple-delivery methods for brain tumor treatment and 3 papers used chemotherapy techniques.
According to Appendix 1 in Supplementary File, from 59 full-text papers, 19 were review and 40 were original (Appendix 2 in Supplementary File). Statistical analysis showed that 50% of the papers used a virus, 36% used polymers, and 14% used cells as carriers to transfect genes as a therapeutic agent in the brain tumor model.
5.3. Included Trials
Review papers were omitted from quantitative synthesis (excluded papers n = 19) and only original papers (n = 40) were analyzed due to the methodology of the study. One of these original studies was about the clinical environment and 9 of them worked only in vitro (Appendix 3 in Supplementary File); so, they were excluded, and only original papers (n = 30) that worked in both in vitro and in vivo environments together were selected for statistical analysis study.
The 30 selected papers were discussed according to their main results (Table 1). These papers examined the effect of gene therapy combined with conventional methods in brain cancer therapy. We just focused on in vivo part of the results because the in vivo results are more extended to clinical trials.
Summary of the Studies Included in Statistical Analysis
Studies | Animal Model | Treatment Group | Control Group | Main Results | Course of Treatment |
---|---|---|---|---|---|
Yamini et al. (15) | N = 70 | Ad. Egr-TNF+TMZ+IR | 1. Ad. Egr. TNF | Animal survivalists increased when Ad. Egr-TNF+TMZ+IR as a triple treatment was used. | 31 days |
2. IR | |||||
3. TMZ | |||||
4. Ad. Egr. TNF +IR | |||||
5. IR+TMZ | |||||
6. Ad.Egr. TNF+TMZ | |||||
7. PBS | |||||
Lamfers et al. (16) | N = 42 | Ad5-D24RGD+ WBI | 1. Ad5. D24RGD | These selected tumor models underscore effectiveness and significance in assessing the effects of combination therapies. | 31 days |
2. Total body irradiation (TBI) | |||||
3. Whole brain irradiation (WBI) | |||||
4. Ad5. D24RGD+TBI | |||||
5. PBS | |||||
Wu et al. (17) | N = 66 | CED of BD-C225 + i.v. BPA + BNCT | 1. Irradiated control | The combination group showed the best results. | 30 days |
2. i.v. BPA+BNCT | |||||
3. i.t. of BD. C225 + BNCT | |||||
4. CED of BD. C225 + BNCT | |||||
5. PBS | |||||
Maguire et al. (18) | N = 12 | AAV-hIFN-β | AAV. empty vector (AAV. ev) | Treatment with AAV-hIFN-β prevented aggressive glioma growth. | 23 days |
Szentirmai et al. (19) | N = 28 | Ad-VEGFR2+Ad-ES | 1. Ad. Fc | Combination treatment of Ad-VEGFR2+Ad-ES revealed the smallest microvessel density and decreased tumor growth. | 14 days |
2. Ad. VEGFR2 | |||||
3. Ad. ES | |||||
Hingtgen et al. (20) | N = 21 | Neural stem cell-S_TRAIL+TMZ | 1. AAV. S. TRAIL+TMZ | A combination of the clinically relevant TMZ and S-TRAIL increased potency for glioblastoma patients. | 12 days |
2. PBS | |||||
Sonabend et al. (21) | N = 21 | pmIL-12/PPC+BCNU | 1. Murine plasmid encoding IL. 12 (pmIL. 12)/ polyethyleneimine covalently modified with methoxy polyethylene glycol and cholesterol (PPC) | BCNU chemotherapy and the antitumor properties of pmIL-12/PPC have synergistic effects. | 40 days |
2. PBS | |||||
Matsuda et al. (22) | N = 21 | HVJ+Eg5 siRNA | 1. Eg5 siRNA | HVJ+Eg5 siRNA treated group showed abundant apoptotic cells in the tumor tissues. | 80 days |
2. PBS | |||||
Jeong et al. (23) | N = 21 | Ad-stTRAIL+BCNU | 1. Ad. stTRAIL | Combination therapy was more effective than individual therapy. | 45 days |
2. PBS | |||||
Lun et al. (24) | N = 35 | vvDD-EGFP+ cyclophosphamide | 1. Dead virus (oncolytic) | The combination group showed more proportion surviving time. | 40 days |
2. vvDD. EGFP | |||||
3. Cyclophosphamide | |||||
Khan et al. (25) | N = 21 | Thymidine kinase 1 (toTK1)/AZT | 1. Prodrug zidovudine (AZT) | Combination therapy of the TK1/AZT suicide gene showed a better effect. | 20 days |
2. PBS | |||||
Lun et al. (26) | N = 28 | JX-594m+Rapamycin | 1. Rapamycin | JX-594m+Rapamycin combination showed the best results. | 21 days |
2. JX. 594m | |||||
3. PBS | |||||
Ho et al. (27) | N = 28 | pG8-FasL+pG8-FADD +TMZ | 1. TMZ | Combination therapy of pG8-FasL+pG8-FADD +TMZ showed more survival percentage. | 19 days |
2. pG8. FasL+pG8. FADD | |||||
3. PBS | |||||
Kato et al. (28) | N = 28 | TMZ/ LipoTrust/MGMT-siRNA | 1. TMZ | Combination of TMZ and siRNA was found to decrease tumor growth significantly. | 9 days |
2. LipoTrust/MGMT. siRNA (DNA repair protein O . methylguanine. DNA methyltransferase) | |||||
3. PBS | |||||
Thomas et al. (29) | N = 70 | T cell +MYXV+ anti-IFN | 1. MYXV | The group that received the triple combination treatment lived significantly longer. | 15 days |
2. MYXV+anti. IFNβ | |||||
3. T cells | |||||
4. T cells+ anti. IFNβ | |||||
5. T cell +MYXV | |||||
6. PBS | |||||
Seol et al. (30) | N = 28 | F3.CE/CPT-11 | 1. F3.C3 cells | F3.CE/CPT-11 group showed significantly prolonged survival periods. | 25 days |
2. CPT. 11 prodrug | |||||
3. PBS | |||||
Kim et al. (9) | N = 28 | MK886+MSC-TRAIL | 1. MK886 | Combination therapy of the MK886+MSC-TRAIL group had a better effect. | 28 days |
2. MSC. TRAIL | |||||
3. PBS | |||||
Candolfi et al. (10) | N = 14 | Ad.TK+Ad.Flt3L | PBS | Ad.TK+Ad.Flt3L intracranial administration in the brain was safe. | 28 days |
Ryu et al. (31) | N = 28 | MSCs-TK+VPA | 1. Intraperitoneal injection of VPA | The apoptotic cell death in intracranial gliomas was enhanced in the combination therapy of MSCs-TK+VPA. | 38 days |
2. MSCs infected with AD. HSV. TK (MSCs. TK) | |||||
3. PBS | |||||
Han et al. (32) | N = 35 | BCNU/PLA wafer + Tf-PLA-BCNU NPs | 1. PLA NPs | The combination group showed significant results in the survival ratio. | 15 days |
2. BCNU/PLA wafer | |||||
3. Tf. PLA. BCNU NPs | |||||
4. PBS | |||||
Liu et al. (33) | N = 72 | DGPT/ pORF-hTRAIL NPs | 1. DOX | DGDPT/pORF-hTRAIL NPs treatment group showed the best results. | 22 days |
2. T7. modified co. delivery nanoparticles (DGDPT/pORF. hTRAIL) NPs | |||||
3. DGDP/pORF. hTRAIL NPs | |||||
4. DGDPT/pGL. 3 | |||||
5. PBS | |||||
Hickey et al. (34) | N = 42 | 1-alloreactive CTL (alloCTL) (2) | PBS (2) | A viable strategy in the treatment of established breast tumors in the brain is combining cellular and suicide genes. | 75 days |
2-peripheral blood mononuclear cells (PBMC) (2) | |||||
Tu et al. (35) | N = 82 | TF + LPS + Ra | 1. LPS (lipopolysaccharide) + Ra | Radiosurgery enhanced treatment with TF + LPS and selectively induce thrombosis of the capillaries and medium-sized vessels of glioblastoma. | 18 days |
2. TF (soluble tissue factor) + Ra | |||||
3. Coadministration TF+LPS | |||||
4. PBS + Ra | |||||
Li et al. (1) | N = 35 | DPC/DOX-Trail | 1. DOX | DPC/DOX-Trail treatment group showed more median survival time and also body weight showed a slow decrease after the 27th day. | 25 days |
2. DPC (DGL. PEG. 10)/Trail | |||||
3. DP (DGL. PEG)/DOX. Trail | |||||
4. PBS | |||||
Yang et al. (36) | N = 20 | At-Lp/siRNA/DTX | 1. Angiopep. 2/tLyP. 1 dual peptides. modified liposomes (At. Lp)/ scrambled siRNAs (siN.C.) | The most effective anti-tumor growth effect was reported for the Co-delivery system (At-Lp/siRNA/DTX). | 10 days |
2. At. Lp/siRNA | |||||
3. At. Lp/ Docetaxel (DTX) | |||||
4. PBS | |||||
Qian et al. (37) | N = 42 | DOX-loaded and miR-21i-complexed sCPM3 (D-sCPM3/miR-21i) | 1. Star. branched copolymer micelles (sCPM3) | Co-delivery of drugs and genes via polymer nanoparticles showed the maximal synergistic/combined effect of drug and gene therapies. | 22 days |
2. DOX | |||||
3. D. sCPM3 | |||||
4. miR. 21i. complexed sCPM3 (sCPM3/miR. 21i | |||||
5. PBS | |||||
Mangraviti et al. (38) | N = 48 | NP-GFP-HSV-tk+GCV | 1. Ganciclovir (GCV) | The median survival of the NP-GFP-HSV-tk+GCV group was significantly longer compared to the untreated group. | 15 days |
2. HSV. tk+GCV | |||||
3. NP. GFP+GCV | |||||
4. PBS | |||||
Park et al. (39) | N = 21 | pHSVtk/R7L10-Cur | 1. pEmpty/R7L10. Cur | Curcumin in combination with the HSVtk gene increased the therapeutic effect on the suppression of tumor growth. | 21 days |
2. PBS | |||||
Chow et al. (40) | N = 40 lateral ventricle N = 16 hippocampus | AAV- mTSG (mouse- homolog tumor suppressor gene) | 1. AAV. vector | In the AAV- mTSG group tumors were developed. | 120 days |
2. PBS | |||||
Jaime‐Ramirez et al. (41) | N = 20 | OV-ChaseM+TMZ | 1. OV. ChaseM | Combination therapy reduced glioma cell aggregation and increase apoptosis cells. | 22 days |
2. TMZ | |||||
3. PBS |
5.4. Analysis of the Total Gene Therapy Effectiveness
Results showed that 50% of the studies used viruses and 36% of them used polymers and only 14% of them used cells as the carrier. The statistical analysis showed statistically significant differences between the treatment and control groups in using viruses, cells, and polymers as carriers. The survivability in groups receiving co-treatment of each carrier in gene therapy + chemotherapy was higher than in the groups receiving only gene or chemotherapy as monotherapy; 53% of virus-mediated studies reported that the brain tumor was removed (100%), while 33% of them reported an 85 to 90% reduction in brain tumor size, and only 14% of the included studies had 70 to 80% tumor size reduction in the animals. Only 4 studies were included in the statistical analysis that used cells as the carrier in the gene therapy. The brain tumor was removed in 75% of these studies and only 25% of them showed a 75% reduction in brain tumor size. Polymers showed that they could act as a better carrier for transfecting genes or drugs into cells. According to the included studies in this systematic review, in 73% of the included studies, a brain tumor was removed; 27% of the included studies reported a 50% reduction in brain tumor size.
6. Discussion
Gene therapy is one of the novel treatments for many diseases including cancer. However, the carriers and targets must be properly selected to optimize this method (42). Studies included in this review have introduced viruses, cells, and polymers to improve gene therapy. Using viruses as vectors could be a promising method in gene therapy because of their safety and lacking major side effects (43, 44). However, gene therapy for malignant glioma with non-replicating viruses has been disappointing. A method that was found to potentiate the anti-tumor effect of virus-mediated oncolysis is systemic chemotherapy, which may be beneficial in glioma patients but has not been explored yet (43). HVJ-E was applied as a non-viral vector for drug delivery by using inactivated Sendai virus. HVJ-E can deliver DNA, siRNA, anti-cancer drugs, and proteins into cells both in vitro and in vivo. Thereafter, the combination of the HVJ-E and macromolecules showed more anti-tumor effects (22).
Cells were applied in cancer therapy in the form of targets and vehicles. Studies showed the advantages of the immortalized and genetically engineered stem cells in gene therapy of brain tumors. These cells selectively migrate into brain tumors and reduce tumor growth both in vitro and in vivo. In an investigation, genetically engineered neural stem cells were used as therapeutics (30).
Lipids, saccharides, and peptide-based polymers were also used as the carrier in gene and drug delivery too much. Among these polymers, lipophosphids (liposomes) could transfer into the cell and also escape from endosomes as well, because of the similarity to the cell membrane (36). Nanoparticles also showed interesting results in drug and gene delivery. For example, star-branched copolymer micelles in combination with genes and chemotherapy had maximal synergistic effects (37).
Overall, according to the statistical analysis results, all of the studies propose that co-treatment of the gene with one of the conventional therapies like radiotherapy or chemotherapy could be more effective in brain cancers than monotherapy methods. In addition, all of the in vitro results were under in vivo ones and confirmed the co-treatment therapy benefits.
6.1. Conclusions
The present systematic review showed that there were more studies on using gene therapy alone or in combination with other conventional methods that confirmed the effects of the genes on the treatment of brain cancer in the in vitro and in vivo environment. In addition, the results suggested the best methods of drug delivery and compared them with each other. However, there was not yet fully explored literature available on using gene therapy as mono or co-therapy in the clinical trial and most of these approaches are purely investigational and will never be tested in a large clinical trial. Generally, the only effective treatment for glioblastoma is still chemo-radiation. These findings bold the research gaps and offer many prospects for further clinical studies. Therefore, this study suggests using gene therapy in the clinical trial as a co-treatment for a variety of cancer therapies to a better understanding of the advantages of gene therapy on the survival probability and life quality of the patients.
References
-
1.
Li J, Guo Y, Kuang Y, An S, Ma H, Jiang C. Choline transporter-targeting and co-delivery system for glioma therapy. Biomaterials. 2013;34(36):9142-8. [PubMed ID: 23993342]. https://doi.org/10.1016/j.biomaterials.2013.08.030.
-
2.
Gutenberg A, Bock HC, Bruck W, Doerner L, Mehdorn HM, Roggendorf W, et al. MGMT promoter methylation status and prognosis of patients with primary or recurrent glioblastoma treated with carmustine wafers. Br J Neurosurg. 2013;27(6):772-8. [PubMed ID: 23662801]. https://doi.org/10.3109/02688697.2013.791664.
-
3.
Berger LA, Riesenberg H, Bokemeyer C, Atanackovic D. CNS metastases in non-small-cell lung cancer: current role of EGFR-TKI therapy and future perspectives. Lung Cancer. 2013;80(3):242-8. [PubMed ID: 23453646]. https://doi.org/10.1016/j.lungcan.2013.02.004.
-
4.
Yin AA, Cheng JX, Zhang X, Liu BL. The treatment of glioblastomas: a systematic update on clinical Phase III trials. Crit Rev Oncol Hematol. 2013;87(3):265-82. [PubMed ID: 23453191]. https://doi.org/10.1016/j.critrevonc.2013.01.007.
-
5.
Puntel M, A K, Farrokhi C, Vanderveen N, Paran C, Appelhans A, et al. Safety profile, efficacy, and biodistribution of a bicistronic high-capacity adenovirus vector encoding a combined immunostimulation and cytotoxic gene therapy as a prelude to a phase I clinical trial for glioblastoma. Toxicol Appl Pharmacol. 2013;268(3):318-30. [PubMed ID: 23403069]. [PubMed Central ID: PMC3641940]. https://doi.org/10.1016/j.taap.2013.02.001.
-
6.
Chen X, Zang W, Xue F, Shen Z, Zhang Q. Bioinformatics analysis reveals potential candidate drugs for different subtypes of glioma. Neurol Sci. 2013;34(7):1139-43. [PubMed ID: 23053832]. https://doi.org/10.1007/s10072-012-1198-3.
-
7.
Liu G, Swierczewska M, Lee S, Chen X. Functional Nanoparticles for Molecular Imaging Guided Gene Delivery. Nano Today. 2010;5(6):524-39. [PubMed ID: 22473061]. [PubMed Central ID: PMC3004232]. https://doi.org/10.1016/j.nantod.2010.10.005.
-
8.
Ohadi M, Rasouli R, Darzi-Eslam E, Jafari A, Ehsani P. Expression of Shigella flexneri ipaB gene in tobacco. Avicenna J Med Biotechnol. 2013;5(2):118-24.
-
9.
Kim SM, Woo JS, Jeong CH, Ryu CH, Lim JY, Jeun SS. Effective combination therapy for malignant glioma with TRAIL-secreting mesenchymal stem cells and lipoxygenase inhibitor MK886. Cancer Res. 2012;72(18):4807-17. [PubMed ID: 22962275]. https://doi.org/10.1158/0008-5472.CAN-12-0123.
-
10.
Candolfi M, King GD, Yagiz K, Curtin JF, Mineharu Y, Muhammad AK, et al. Plasmacytoid dendritic cells in the tumor microenvironment: immune targets for glioma therapeutics. Neoplasia. 2012;14(8):757-70. [PubMed ID: 22952428]. [PubMed Central ID: PMC3431182]. https://doi.org/10.1593/neo.12794.
-
11.
Przystal JM, Umukoro E, Stoneham CA, Yata T, O'Neill K, Syed N, et al. Proteasome inhibition in cancer is associated with enhanced tumor targeting by the adeno-associated virus/phage. Mol Oncol. 2013;7(1):55-66. [PubMed ID: 22951279]. [PubMed Central ID: PMC3553581]. https://doi.org/10.1016/j.molonc.2012.08.001.
-
12.
Lima FR, Kahn SA, Soletti RC, Biasoli D, Alves T, da Fonseca AC, et al. Glioblastoma: therapeutic challenges, what lies ahead. Biochim Biophys Acta. 2012;1826(2):338-49. [PubMed ID: 22677165]. https://doi.org/10.1016/j.bbcan.2012.05.004.
-
13.
Moher D, Liberati A, Tetzlaff J, Altman DG. Preferred reporting items for systematic reviews and meta-analyses: the PRISMA statement. PLoS Med. 2009;6(7). e1000097. [PubMed ID: 19621072]. [PubMed Central ID: PMC2707599]. https://doi.org/10.1371/journal.pmed.1000097.
-
14.
Higgins JP, Thomas J, Chandler J, Cumpston M, Li T, Page MJ, et al. Cochrane handbook for systematic reviews of interventions. John Wiley & Sons; 2011.
-
15.
Yamini B, Yu X, Pytel P, Galanopoulos N, Rawlani V, Veerapong J, et al. Adenovirally delivered tumor necrosis factor-alpha improves the antiglioma efficacy of concomitant radiation and temozolomide therapy. Clin Cancer Res. 2007;13(20):6217-23. [PubMed ID: 17947489]. https://doi.org/10.1158/1078-0432.CCR-07-1421.
-
16.
Lamfers ML, Idema S, Bosscher L, Heukelom S, Moeniralm S, van der Meulen-Muileman IH, et al. Differential effects of combined Ad5- delta 24RGD and radiation therapy in in vitro versus in vivo models of malignant glioma. Clin Cancer Res. 2007;13(24):7451-8. [PubMed ID: 18094429]. https://doi.org/10.1158/1078-0432.CCR-07-1265.
-
17.
Wu G, Yang W, Barth RF, Kawabata S, Swindall M, Bandyopadhyaya AK, et al. Molecular targeting and treatment of an epidermal growth factor receptor-positive glioma using boronated cetuximab. Clin Cancer Res. 2007;13(4):1260-8. [PubMed ID: 17317838]. https://doi.org/10.1158/1078-0432.CCR-06-2399.
-
18.
Maguire CA, Meijer DH, LeRoy SG, Tierney LA, Broekman ML, Costa FF, et al. Preventing growth of brain tumors by creating a zone of resistance. Mol Ther. 2008;16(10):1695-702. [PubMed ID: 18714312]. [PubMed Central ID: PMC2863297]. https://doi.org/10.1038/mt.2008.168.
-
19.
Szentirmai O, Baker CH, Bullain SS, Lin N, Takahashi M, Folkman J, et al. Successful inhibition of intracranial human glioblastoma multiforme xenograft growth via systemic adenoviral delivery of soluble endostatin and soluble vascular endothelial growth factor receptor-2: laboratory investigation. J Neurosurg. 2008;108(5):979-88. [PubMed ID: 18447716]. [PubMed Central ID: PMC4459889]. https://doi.org/10.3171/JNS/2008/108/5/0979.
-
20.
Hingtgen S, Ren X, Terwilliger E, Classon M, Weissleder R, Shah K. Targeting multiple pathways in gliomas with stem cell and viral delivered S-TRAIL and Temozolomide. Mol Cancer Ther. 2008;7(11):3575-85. [PubMed ID: 19001440]. [PubMed Central ID: PMC2748233]. https://doi.org/10.1158/1535-7163.MCT-08-0640.
-
21.
Sonabend AM, Velicu S, Ulasov IV, Han Y, Tyler B, Brem H, et al. A safety and efficacy study of local delivery of interleukin-12 transgene by PPC polymer in a model of experimental glioma. Anticancer Drugs. 2008;19(2):133-42. [PubMed ID: 18176109]. https://doi.org/10.1097/CAD.0b013e3282f24017.
-
22.
Matsuda M, Yamamoto T, Matsumura A, Kaneda Y. Highly efficient eradication of intracranial glioblastoma using Eg5 siRNA combined with HVJ envelope. Gene Ther. 2009;16(12):1465-76. [PubMed ID: 19675593]. https://doi.org/10.1038/gt.2009.99.
-
23.
Jeong M, Kwon YS, Park SH, Kim CY, Jeun SS, Song KW, et al. Possible novel therapy for malignant gliomas with secretable trimeric TRAIL. PLoS One. 2009;4(2). e4545. [PubMed ID: 19229339]. [PubMed Central ID: PMC2641005]. https://doi.org/10.1371/journal.pone.0004545.
-
24.
Lun XQ, Jang JH, Tang N, Deng H, Head R, Bell JC, et al. Efficacy of systemically administered oncolytic vaccinia virotherapy for malignant gliomas is enhanced by combination therapy with rapamycin or cyclophosphamide. Clin Cancer Res. 2009;15(8):2777-88. [PubMed ID: 19351762]. https://doi.org/10.1158/1078-0432.CCR-08-2342.
-
25.
Khan Z, Knecht W, Willer M, Rozpedowska E, Kristoffersen P, Clausen AR, et al. Plant thymidine kinase 1: a novel efficient suicide gene for malignant glioma therapy. Neuro Oncol. 2010;12(6):549-58. [PubMed ID: 20154339]. [PubMed Central ID: PMC2940637]. https://doi.org/10.1093/neuonc/nop067.
-
26.
Lun X, Chan J, Zhou H, Sun B, Kelly JJ, Stechishin OO, et al. Efficacy and safety/toxicity study of recombinant vaccinia virus JX-594 in two immunocompetent animal models of glioma. Mol Ther. 2010;18(11):1927-36. [PubMed ID: 20808290]. [PubMed Central ID: PMC2990519]. https://doi.org/10.1038/mt.2010.183.
-
27.
Ho IA, Ng WH, Lam PY. FasL and FADD delivery by a glioma-specific and cell cycle-dependent HSV-1 amplicon virus enhanced apoptosis in primary human brain tumors. Mol Cancer. 2010;9:270. [PubMed ID: 20942909]. [PubMed Central ID: PMC2964619]. https://doi.org/10.1186/1476-4598-9-270.
-
28.
Kato T, Natsume A, Toda H, Iwamizu H, Sugita T, Hachisu R, et al. Efficient delivery of liposome-mediated MGMT-siRNA reinforces the cytotoxity of temozolomide in GBM-initiating cells. Gene Ther. 2010;17(11):1363-71. [PubMed ID: 20520650]. https://doi.org/10.1038/gt.2010.88.
-
29.
Thomas DL, Doty R, Tosic V, Liu J, Kranz DM, McFadden G, et al. Myxoma virus combined with rapamycin treatment enhances adoptive T cell therapy for murine melanoma brain tumors. Cancer Immunol Immunother. 2011;60(10):1461-72. [PubMed ID: 21656158]. [PubMed Central ID: PMC4378695]. https://doi.org/10.1007/s00262-011-1045-z.
-
30.
Seol HJ, Jin J, Seong DH, Joo KM, Kang W, Yang H, et al. Genetically engineered human neural stem cells with rabbit carboxyl esterase can target brain metastasis from breast cancer. Cancer Lett. 2011;311(2):152-9. [PubMed ID: 21868150]. https://doi.org/10.1016/j.canlet.2011.07.001.
-
31.
Ryu CH, Park KY, Kim SM, Jeong CH, Woo JS, Hou Y, et al. Valproic acid enhances anti-tumor effect of mesenchymal stem cell mediated HSV-TK gene therapy in intracranial glioma. Biochem Biophys Res Commun. 2012;421(3):585-90. [PubMed ID: 22525671]. https://doi.org/10.1016/j.bbrc.2012.04.050.
-
32.
Han L, Ren Y, Long L, Zhong Y, Shen C, Pu P, et al. Inhibition of C6 glioma in vivo by combination chemotherapy of implantation of polymer wafer and intracarotid perfusion of transferrin-decorated nanoparticles. Oncol Rep. 2012;27(1):121-8. [PubMed ID: 21922149]. https://doi.org/10.3892/or.2011.1459.
-
33.
Liu S, Guo Y, Huang R, Li J, Huang S, Kuang Y, et al. Gene and doxorubicin co-delivery system for targeting therapy of glioma. Biomaterials. 2012;33(19):4907-16. [PubMed ID: 22484049]. https://doi.org/10.1016/j.biomaterials.2012.03.031.
-
34.
Hickey MJ, Malone CC, Erickson KL, Lin A, Soto H, Ha ET, et al. Combined alloreactive CTL cellular therapy with prodrug activator gene therapy in a model of breast cancer metastatic to the brain. Clin Cancer Res. 2013;19(15):4137-48. [PubMed ID: 23780889]. [PubMed Central ID: PMC3939036]. https://doi.org/10.1158/1078-0432.CCR-12-3735.
-
35.
Tu J, Hu Z, Chen Z. A combination of radiosurgery and soluble tissue factor enhances vascular targeting for experimental glioblastoma. Biomed Res Int. 2013;2013:390714. [PubMed ID: 24307995]. [PubMed Central ID: PMC3838847]. https://doi.org/10.1155/2013/390714.
-
36.
Yang ZZ, Li JQ, Wang ZZ, Dong DW, Qi XR. Tumor-targeting dual peptides-modified cationic liposomes for delivery of siRNA and docetaxel to gliomas. Biomaterials. 2014;35(19):5226-39. [PubMed ID: 24695093]. https://doi.org/10.1016/j.biomaterials.2014.03.017.
-
37.
Qian X, Long L, Shi Z, Liu C, Qiu M, Sheng J, et al. Star-branched amphiphilic PLA-b-PDMAEMA copolymers for co-delivery of miR-21 inhibitor and doxorubicin to treat glioma. Biomaterials. 2014;35(7):2322-35. [PubMed ID: 24332459]. https://doi.org/10.1016/j.biomaterials.2013.11.039.
-
38.
Mangraviti A, Tzeng SY, Kozielski KL, Wang Y, Jin Y, Gullotti D, et al. Polymeric nanoparticles for nonviral gene therapy extend brain tumor survival in vivo. ACS Nano. 2015;9(2):1236-49. [PubMed ID: 25643235]. [PubMed Central ID: PMC4342728]. https://doi.org/10.1021/nn504905q.
-
39.
Park JH, Han J, Lee M. Thymidine kinase gene delivery using curcumin loaded peptide micelles as a combination therapy for glioblastoma. Pharm Res. 2015;32(2):528-37. [PubMed ID: 25158647]. https://doi.org/10.1007/s11095-014-1482-4.
-
40.
Chow RD, Guzman CD, Wang G, Schmidt F, Youngblood MW, Ye L, et al. AAV-mediated direct in vivo CRISPR screen identifies functional suppressors in glioblastoma. Nat Neurosci. 2017;20(10):1329-41. [PubMed ID: 28805815]. [PubMed Central ID: PMC5614841]. https://doi.org/10.1038/nn.4620.
-
41.
Jaime-Ramirez AC, Dmitrieva N, Yoo JY, Banasavadi-Siddegowda Y, Zhang J, Relation T, et al. Humanized chondroitinase ABC sensitizes glioblastoma cells to temozolomide. J Gene Med. 2017;19(3). [PubMed ID: 28087981]. [PubMed Central ID: PMC5382089]. https://doi.org/10.1002/jgm.2942.
-
42.
Goncalves GAR, Paiva RMA. Gene therapy: advances, challenges and perspectives. Einstein (Sao Paulo). 2017;15(3):369-75. [PubMed ID: 29091160]. [PubMed Central ID: PMC5823056]. https://doi.org/10.1590/S1679-45082017RB4024.
-
43.
Rainov NG, Heidecke V. Clinical development of experimental virus-mediated gene therapy for malignant glioma. Anticancer Agents Med Chem. 2011;11(8):739-47. [PubMed ID: 21707496]. https://doi.org/10.2174/187152011797378724.
-
44.
Brouwer E, Havenga MJ, Ophorst O, de Leeuw B, Gijsbers L, Gillissen G, et al. Human adenovirus type 35 vector for gene therapy of brain cancer: improved transduction and bypass of pre-existing anti-vector immunity in cancer patients. Cancer Gene Ther. 2007;14(2):211-9. [PubMed ID: 17082793]. https://doi.org/10.1038/sj.cgt.7701010.