Abstract
Background:
Spironolactone is a conventional drug widely in use for the treatment of heart failure and hypertension patients. On the other side recent studies have reported spironolactone can prevent growth and drug resistance in cancer stem cells (CSCs), by inhibiting DNA double-strand break (DSB) repair; suggesting its potential application in cancer therapy.Objectives:
Our study aimed at assessing the potential cytotoxicity of spironolactone in human U87-MG glioblastoma cells.Methods:
Different concentrations of spironolactone (0 - 50 μM) for 48 and 72 h were used for treatment. Cell viability assay was carried out by the 4, 5-dimethylthiazole-2-yl, 2, 5-diphenyl tetrazolium (MTT) method. Apoptosis was evaluated using annexin V/PI staining and flow cytometry and colorimetric measurement of caspase 8 and 9 activity.Results:
Our findings showed a significant dose-dependent cytotoxic effect of spironolactone with maximum effect in 30 μM (P-value < 0.05). Spironolactone can induce approximately 20% apoptotic cell death in U87-MG cancer cells which were mainly related to early apoptotic cells. Indeed, the activity of caspase 8 and 9 was significantly elevated in spironolactone-treated cells compared to mock control.Conclusions:
Findings showed the cytotoxic effect of spironolactone in U87-MG glioblastoma cancer cells in a mechanism dependent on apoptosis cell death induction. Our findings suggest the potential application of spironolactone in the treatment of glioblastoma in vitro.Keywords
1. Background
Glioblastoma is a kind of primary central nervous system tumor originating from astrocytes. It accounts for the majority of cases of malignant glioma (1, 2). In adults, mostly glioblastoma multiforme (GBM) is an aggressive primary brain tumor and is classified by the World Health Organization (WHO) as a grade IV malignant glioma (3). As the most common primary CNS malignancy, glioblastoma has an incidence rate of 3.22 per 100,000 population. Besides the advanced medicinal treatments, unfortunately, patients with glioblastoma have a poor prognosis with 5-year relative survival rates of 6.8% in the United States (reported in 2012 - 2016) (4). In this regard, finding novel therapeutic approaches is appealing to provide a better clinical outcome for these patients. Instead of drug discovery which demands time and cost, drug repositioning can be more rapid and feasible. Drug repositioning is a novel approach to reusing Food and Drug Administration (FDA)-approved drugs for treating other diseases. Moreover, it has been more advantageous concerning drug pharmacokinetics and safety profiles, that are already known (5).
Currently, several repositioned drugs including metformin, chloroquine, and disulfiram which are approved by FDA entered phase 1 and 2 clinical trials for the treatment of glioblastoma (6). Another approved drug with the potential for repositioning is spironolactone (brand name: Aldactone). Spironolactone is a mineralocorticoid receptor antagonist which has been approved by the FDA for treatment of Class III-IV heart failure and reduced ejection renal function periodically, hypertension, primary hyperaldosteronism, and management of edema (7).
2. Objectives
Recently it has been reported that spironolactone, an aldosterone antagonist, can inhibit cancer and cancer stem cells in vitro and in vivo in a mechanism dependent on homology-directed repair (HDR) inhibition and double-strand DNA break repair disruption (8, 9). Therefore, in the current study, we aimed to find out whether spironolactone repositioning is effective to inhibit the human U87-MG glioblastoma cells.
3. Methods
3.1. Reagents
Dulbecco’s modified Eagle’s medium (DMEM), trypsin-EDTA, penicillin-streptomycin (pen/strep), and fetal bovine serum (FBS) were ordered from Gibco (Grand Island, NY). The 4, 5-dimethylthiazole-2-yl, 2, 5-diphenyl tetrazolium (MTT) was from Autocell and dimethyl sulfoxide (DMSO) was procured from Sigma (St. Louis,MO, USA). The spironolactone was provided by Tocris bioscience (Bristol, UK). The apoptosis assay kit was purchased from MabTag GmbH (Germany, Gladiolenweg) and for caspase 8 and 9 activity assay the R&D systems (Minneapolis, USA) were used.
3.2. Cell Culture
The U87-MG human glioblastoma cell line was provided by the Pasteur Institute of Iran (Tehran, Iran). Cell line was cultured in DMEM supplemented with 10% FBS and 1% pen/strep antibiotics in a 5% CO2 humidified incubator at 37°C temperature. The medium was refreshed every 3 days during the experiments. This study was approved by the ethical committee of Golestan University of Medical Sciences (IR.GOUMS.REC.1397.143)
3.3. Cell Toxicity Assay (4, 5-Dimethylthiazole-2-yl, 2, 5-Diphenyl Tetrazolium (MTT) Assay)
For viability assay, the U87-MG cells were seeded in a 96-well plate with 104 densities per well. After 24 hours, the cells were incubated with different concentrations of spironolactone ranging from 0 - 50 μM for 48 and 72 h in a complete medium. For more confirmation, all the treatments were done in 5 replicates. The DMSO 1% was used as vehicle control for all experiments. Eventually, MTT solution (final concentration 0.5mg/ml) was added to each well and after 5 h, the supernatant was removed and the formazan precipitates were dissolved in 10% DMSO by gentle shaking in the darkness. The optical density was measured at 570 nm using ELISA Plate reader stat FAX 303 at 570 nm. The absorbance was normalized to the mean of DMSO 1% treated control cells and the percentage of cell viability was calculated for all wells.
3.4. Flow Cytometric Evaluation of Apoptosis
The apoptotic cell death was measured using the annexin PI staining kit and flow cytometry method. For this purpose, the U87-MG cells were seeded with a number of 3 × 105 per well in a 6-well plate and after 24 h the cells were treated with 30 μM of spironolactone or DMSO 1% for 48 hours in complete culture media. Then the cells were detached using EDTA- trypsin and were washed. The cells were stained using annexin an V/PI apoptosis assay kit (MabTag) according to the manufacturer’s instructions. Then the cells were analyzed using the flow- cytometry apparatus (BD Accuri™ C6) FL-1 (annexin V) and FL-3 (PI). The non-stained cells were used to remove the background and then early (annexin V positive cells) and late (annexin V and PI positive cell) apoptotic cells or the total apoptotic cells (early + late) were quantified.
3.5. Caspase Activity Measurement
To confirm the apoptotic cell response, the activities of caspase 8 and 9 were measured in treated cells. Briefly, the number of 104 cells per well U87-MG cells was seeded in 96 well plates and then after 24 h, the cells were treated with 30 μM of spironolactone or DMSO 1% for 48 hours in complete culture media. The non-treated cells in this experiment were used as a control for normalization. The R&D caspase 8 and 9 colorimetric assays were used according to the manufacturer’s protocol and finally, the absorbance was recorded at a wavelength of 405nm. The caspase activity was calculated as % normalized to the activity measured in non-treated (mock) cells.
3.6. Statistical Analysis
Experimental data was analyzed using SPSS software version 19 and the P-value ≤ 0.05 was considered as a significant level. The normality of data was checked with the Shapiro-Wilk test and for viability assay, the one-way ANOVA and LSD post hoc test were used for multiple comparisons. For the apoptosis and caspase activity experiments, the student’s sample t-test or Mann-Whitney U and Kruskal-Wallis tests were when appropriate.
4. Results
4.1. Spironolactone Treatment Decreases Cell Viability of U87-MG Glioblastoma Cell
To evaluate cellular toxicity, U87-MG cancer cells were treated with different concentrations of spironolactone for two different time points of 48 h and 72 h. As described before, the cell viability was measured using the MTT method. As shown in Figure 1A and B, in both time points the maximum cytotoxicity (~20%) was observed in 30 μM spironolactone concentration. Interestingly cell viability percentage significantly decreased in 30 μM spironolactone concentration compared to 1% DMSO after 48 h (P-value = 0.012) and 72 h (P-value < 0.0001) exposure time.
Furthermore, we found dose-dependent toxicity of spironolactone with a significant more toxic effect for 30 μM spironolactone compared to a lower concentration of 10 μM (for 48 h: P-value = 0.03 and for 72 h: P-value < 0.0001) (Figure 1A and B). Although the 50 μM spironolactone had significant toxicity after 72 h treatment (P-value = 0.003) compared to the 1% DMSO group, its effect was not significant in 48 h exposure time. Accordingly, 30 μM spironolactone was selected as the optimum concentration for the next experiments. Of notice, in another study, the same concentrations of spironolactone were tested in HDF normal cell line and as expected; spironolactone had no cytotoxicity in normal human dermal fibroblast (data not shown).
Cell viability of U87-MG glioblastoma cells measured by 4, 5-dimethylthiazole-2-yl, 2, 5-diphenyl tetrazolium (MTT) assay after A, 48; and B, 72 h treatment with different concentrations of spironolactone (μM). The dimethyl sulfoxide 1% was used as a control vehicle. The error bar for each point of concentration represents ± standard error (n = 5).
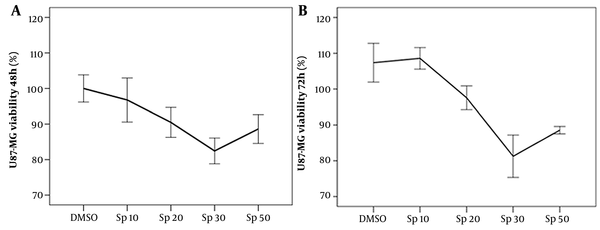
4.2. Spironolactone Treatment Induced Apoptosis in U87-MG Cells
To evaluate whether cytotoxicity occurred through the induction of apoptosis, the annexin V/PI staining was used to measure apoptosis in spironolactone-treated U87-MG glioblastoma cells. As shown in Figure 2, the percentage of apoptotic cells (annexin V + annexin V/PI-positive cells) was increased in U87-MG cells treated with 30 μM spironolactone (Figure 2A). In accordance with the MTT assay which showed 80% cellular viability, we found around 20% total apoptotic cell death in cells treated with 30 μM spironolactone which was significantly more than DMSO 1% group (Student’s t-test P-value = 0.004). Next, we analyzed the percentage of early apoptotic cells (annexin V-positive cells) and late apoptotic cells (annexin V/PI positive cells) separately and found that spironolactone toxicity was mainly related to early apoptosis induction (P-value = 0.03) but not late apoptosis (P-value = 0.1) (Figure 2B).
The apoptotic cell death evaluation of U87-MG cell treated with spironolactone. A, Shows the Annexin V positive cells in FL1-A (X axis) and PI-positive cell in FL3-A (Y axis) in three groups of non-stain control, DMSO 1% treated and 30 μM spironolactone after 48 h. B, Shows the average of total apoptotic and early or late apoptotic cells in a bar chart. * Means P-value = 0.03 and ** means P-value = 0.004.
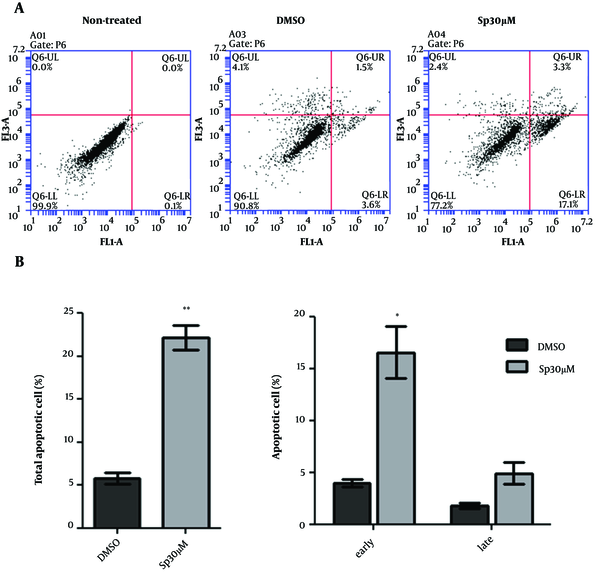
The U87-MG cells had about 2% to 4% necrotic cell death (PI positive cells) in both DMSO and spironolactone treated cell, which might be related to cellular detachment and staining process.
4.3. Spironolactone Treated Cells Had Higher Caspase Activity
The apoptotic death mechanism is associated with increased caspase activity. The activity of caspase-8 as an indicator of intrinsic apoptosis pathway and caspase-9 as an indicator of extrinsic apoptosis response were measured in mock, DMSO 1%, and 30 μM spironolactone (treatment time 48 h). Figure 3A shows that spironolactone treatment increased caspase-8 activity significantly up to an average of 1.6-fold in U87-MG cells (Mann-Whitney U test P-value = 0.03 compared to mock or DMSO 1%). The activity of caspase-9 was also enhanced up to an average of 1.25-fold in U87-MG cells after treatment with spironolactone 30 μM, however, it was significant only when comparing to the mock group (P-value = 0.03) but not to DMSO 1% group (Figure 3B, P-value = 0.05).
A, The caspase-8; and B, caspase-9 activity in U87-MG glioblastoma cells treated with spironolactone. Mock means non-treated cells. DMSO 1% was used as vehicle control and the treatment time was 48 h. * Means P-value < 0.05.
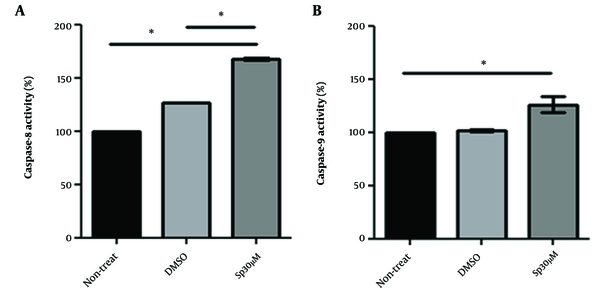
5. Discussion
Briefly, spironolactone is a pharmacologic aldosterone antagonist which is widely used for clinical conditions, for instance, high blood pressure and heart failure (10-12). Spironolactone functions as a diuretic and antihypertensive through competitive binding to the mineralocorticoid receptors in the distal convoluted renal tubule and increases sodium and water secretion while potassium is retained (7, 13).
In addition, recent studies have shown the anti-cancer role of antihypertensive drugs such as spironolactone (14). Since spironolactone is a lipophilic drug and can cross the blood-brain barrier (15), hence it can easily access the brain tumor without limitation; however, its function in brain tumor inhibition should be investigated.
Recently a novel mechanism has been discovered for spironolactone as a HDR inhibitor. In a high throughput screening study, it was revealed that spironolactone can reduce HDR through Rad51 foci formation impairment and inhibits the growth of osteosarcoma U2OS cell (9). Later, Gold et al. demonstrated that spironolactone can inhibit U2OS cancer stem cell proliferation in-vitro and in-vivo resulting in to decrease in the size and count of cancer stem cells (CSCs) in tumors of animal models, suggesting its anticancer role, especially in CSC inhibition (8).
In the present study, we reported a potential new application for an old drug, spironolactone, for inhibition of human glioblastoma U87-MG cancer cells in-vitro. Our finding illustrated that spironolactone (30 μM) alone has dose-dependent cytotoxicity and can induce cell death in a mechanism related to caspase-8/9 and apoptosis induction in U87-MG cells.
The anti-cancer effect of spironolactone was also reported previously in other cancer cells; however, it was used in combination with other agents. A study by Sanomachi et al. reported that spironolactone in combination with gemcitabine and osimertinib increases cell death and suppresses cell growth by reducing surviving synthesis in various cancer cell lines including glioblastoma CSCs (11). They also indicated this combination was effective to suppress tumorogenesis in xenograft mice models without supposed adverse effects (11). In contrast to previous studies, Aldaz et al. showed an anti-proliferative effect for mineralocorticoid receptor activation by aldosterone in some glioblastoma cancer cell lines but no anti-neoblastic effect of spironolactone was reported (16). However, compared to the present study, they used a lower concentration of spironolactone (maximum 10 uM) and they used other glioblastoma cancer cells (T98G, U251, U373, SF188, A172, LN299) but the U87-MG cell line was not tested (16). Although Aldaz et al. reported different roles with proliferation enhancement for spironolactone as a mineralocorticoid receptor antagonist in glioblastoma cancer cells but previous studies have shown that the mechanism related to the apoptosis induction by spironolactone in a cancer cell is not through mineralocorticoid receptor antagonism (8, 16).
Indeed, another novel mechanism has been discovered for spironolactone by Leung et al. they showed that spironolactone can increase NKG2DL expression in colorectal carcinoma cell lines by activating the ATM-Chk2-mediated checkpoint pathway; consequently, promoting cancer cell targeting by the NK immune cells in vitro (17). They also reported that the anti-cancer effect of spironolactone is dependent on retinoid X receptor γ (RXRγ) but not the mineralocorticoid receptor (17). Likewise, Sonder et al. demonstrated that spironolactone induces apoptosis in mononuclear cells, independent of the mineralocorticoid receptor, and possibly through inhibition of the NF-kb messaging pathway (18).
Furthermore, spironolactone can induce the proteolytic degradation of xeroderma pigmentosum group B (XPB) protein, helicase of transcription factor II-H (TFIIH) complex, which plays essential roles in both nucleotide excision DNA repair (NER) and the initiation of transcription. Thus, spironolactone treatment inhibits NER and chemo sensitizes platinum derivatives toward tumor cells (19). Regarding the important role of TFIIH in transcription initiation and also in DNA repair, it has been postulated that spironolactone may raise concerns in regard to mutagenic effect (20).
5.1. Conclusions
Altogether we found the toxic effect of spironolactone in glioblastoma U87-MG cells in a mechanism dependent on apoptosis induction, suggesting a new potential application for an existing approved drug as an anti-cancer agent for glioblastoma treatment.
References
-
1.
Dolecek TA, Propp JM, Stroup NE, Kruchko C. CBTRUS statistical report: primary brain and central nervous system tumors diagnosed in the United States in 2005-2009. Neuro Oncol. 2012;14 Suppl 5:v1-49. [PubMed ID: 23095881]. [PubMed Central ID: PMC3480240]. https://doi.org/10.1093/neuonc/nos218.
-
2.
Fisher JL, Schwartzbaum JA, Wrensch M, Wiemels JL. Epidemiology of brain tumors. Neurol Clin. 2007;25(4):867-90. vii. [PubMed ID: 17964019]. https://doi.org/10.1016/j.ncl.2007.07.002.
-
3.
Oliveira LC, Marques MP, Caseiro AJ, Rapposelli S, Batista-de-Carvalho AL, Fonseca AP. A novel approach in glioblastoma multiforme drug discovery: perturbation studies in vitro. J Appl Pharm Sci. 2019;9(4):58-65. https://doi.org/10.7324/japs.2019.90407.
-
4.
Ostrom QT, Cioffi G, Gittleman H, Patil N, Waite K, Kruchko C, et al. CBTRUS Statistical Report: Primary Brain and Other Central Nervous System Tumors Diagnosed in the United States in 2012-2016. Neuro Oncol. 2019;21(Suppl 5):v1-v100. [PubMed ID: 31675094]. [PubMed Central ID: PMC6823730]. https://doi.org/10.1093/neuonc/noz150.
-
5.
Tan SK, Jermakowicz A, Mookhtiar AK, Nemeroff CB, Schurer SC, Ayad NG. Drug Repositioning in Glioblastoma: A Pathway Perspective. Front Pharmacol. 2018;9:218. [PubMed ID: 29615902]. [PubMed Central ID: PMC5864870]. https://doi.org/10.3389/fphar.2018.00218.
-
6.
Abbruzzese C, Matteoni S, Signore M, Cardone L, Nath K, Glickson JD, et al. Drug repurposing for the treatment of glioblastoma multiforme. J Exp Clin Cancer Res. 2017;36(1):169. [PubMed ID: 29179732]. [PubMed Central ID: PMC5704391]. https://doi.org/10.1186/s13046-017-0642-x.
-
7.
Patibandla S, Heaton J, Kyaw H. Spironolactone. Florida, USA: StatPearls Publishing LLC; 2022. eng.
-
8.
Gold A, Eini L, Nissim-Rafinia M, Viner R, Ezer S, Erez K, et al. Spironolactone inhibits the growth of cancer stem cells by impairing DNA damage response. Oncogene. 2019;38(17):3103-18. [PubMed ID: 30622338]. https://doi.org/10.1038/s41388-018-0654-9.
-
9.
Shahar OD, Kalousi A, Eini L, Fisher B, Weiss A, Darr J, et al. A high-throughput chemical screen with FDA approved drugs reveals that the antihypertensive drug Spironolactone impairs cancer cell survival by inhibiting homology directed repair. Nucleic Acids Res. 2014;42(9):5689-701. [PubMed ID: 24682826]. [PubMed Central ID: PMC4027216]. https://doi.org/10.1093/nar/gku217.
-
10.
Xiang Y, Shi W, Li Z, Yang Y, Wang SY, Xiang R, et al. Efficacy and safety of spironolactone in the heart failure with mid-range ejection fraction and heart failure with preserved ejection fraction: A meta-analysis of randomized clinical trials. Medicine (Baltimore). 2019;98(13). e14967. [PubMed ID: 30921200]. [PubMed Central ID: PMC6456096]. https://doi.org/10.1097/MD.0000000000014967.
-
11.
Sanomachi T, Suzuki S, Togashi K, Sugai A, Seino S, Okada M, et al. Spironolactone, a Classic Potassium-Sparing Diuretic, Reduces Survivin Expression and Chemosensitizes Cancer Cells to Non-DNA-Damaging Anticancer Drugs. Cancers (Basel). 2019;11(10). [PubMed ID: 31614999]. [PubMed Central ID: PMC6826935]. https://doi.org/10.3390/cancers11101550.
-
12.
Kosmas CE, Silverio D, Sourlas A, Montan PD, Guzman E. Role of spironolactone in the treatment of heart failure with preserved ejection fraction. Ann Transl Med. 2018;6(23):461. [PubMed ID: 30603649]. [PubMed Central ID: PMC6312809]. https://doi.org/10.21037/atm.2018.11.16.
-
13.
Searle GD. Aldactone. Maryland, USA: U.S. Food and Drug Administration; 2014.
-
14.
Carlos-Escalante JA, de Jesus-Sanchez M, Rivas-Castro A, Pichardo-Rojas PS, Arce C, Wegman-Ostrosky T. The Use of Antihypertensive Drugs as Coadjuvant Therapy in Cancer. Front Oncol. 2021;11:660943. [PubMed ID: 34094953]. [PubMed Central ID: PMC8173186]. https://doi.org/10.3389/fonc.2021.660943.
-
15.
Schmiedek P, Sadee W, Baethmann A. Cerebral uptake of a 3 H-labelled spirolactone compound in the dog. Eur J Pharmacol. 1973;21(2):238-41. [PubMed ID: 4696105]. https://doi.org/10.1016/0014-2999(73)90232-x.
-
16.
Aldaz P, Fernandez-Celis A, Lopez-Andres N, Arozarena I. Novel Insights into the Role of the Mineralocorticoid Receptor in Human Glioblastoma. Int J Mol Sci. 2021;22(21). [PubMed ID: 34769089]. [PubMed Central ID: PMC8584062]. https://doi.org/10.3390/ijms222111656.
-
17.
Leung WH, Vong QP, Lin W, Janke L, Chen T, Leung W. Modulation of NKG2D ligand expression and metastasis in tumors by spironolactone via RXRgamma activation. J Exp Med. 2013;210(12):2675-92. [PubMed ID: 24190430]. [PubMed Central ID: PMC3832934]. https://doi.org/10.1084/jem.20122292.
-
18.
Sonder SU, Woetmann A, Odum N, Bendtzen K. Spironolactone induces apoptosis and inhibits NF-kappaB independent of the mineralocorticoid receptor. Apoptosis. 2006;11(12):2159-65. [PubMed ID: 17051331]. https://doi.org/10.1007/s10495-006-0286-3.
-
19.
Alekseev S, Ayadi M, Brino L, Egly JM, Larsen AK, Coin F. A small molecule screen identifies an inhibitor of DNA repair inducing the degradation of TFIIH and the chemosensitization of tumor cells to platinum. Chem Biol. 2014;21(3):398-407. [PubMed ID: 24508195]. https://doi.org/10.1016/j.chembiol.2013.12.014.
-
20.
Gabbard RD, Hoopes RR, Kemp MG. Spironolactone and XPB: An Old Drug with a New Molecular Target. Biomolecules. 2020;10(5). [PubMed ID: 32414008]. [PubMed Central ID: PMC7277409]. https://doi.org/10.3390/biom10050756.