Abstract
Background:
Despite substantial efforts to leverage natural killer (NK) cells in cancer immunotherapy, challenges associated with limited cell numbers and sources persist. In this study, our objective is to differentiate NK cells from cord blood hematopoietic stem cells (CB-HSCs) and assess their anti-tumor effects.Methods:
Cord blood samples were obtained from pregnant women undergoing normal delivery. Mononuclear cells (MNC) were isolated by gradient centrifugation. Subsequently, HSCs were isolated using the MACs cell separation kit. The isolated HSCs were cultured in NK cell differentiation and expansion media for 21 days and 7 days, respectively. The NK cells were examined for expression of activating markers, cytokine secretion and cytolytic effects by flow cytometry, ELISA and XTT tests, respectively.Results:
High-purity HSCs and NK cells were obtained in this study. The CB-HSCs-derived NK cells exhibited significantly higher expression of NKG2D, NKp30, NKp44 and NKp46 receptors (at day 28 of treatment) after treatment by IL-15 and IL-2, compared to the early differentiated NK cells (day 21). NK cells derived from CB-HSCs treated with the combination of IL-15 and IL-2 could robustly lyse breast cancer MCF-7 and K562 cells. Also, the obtained NK cells were able to release higher amounts of TNF-α and IFN-γ cytokines in response to tumor cell experiences.Conclusions:
Our findings showed that functionally active CB-HSCs-derived NK cells can be successfully generated ex vivo using a cytokine cocktail without a need for stroma for potential use in the cancer immunotherapy.Keywords
Cord Blood Haematopoietic Stem Cells Natural Killer Cells Breast Cancer Immunotherapy
1. Background
Natural killer (NK) cells play a vital role in the innate immune system with the capability to eradicate diverse tumor cells without a need for prior antigen sensitization (1, 2). Indeed, these cells express a repertoire of inhibitory and activating receptors, enabling them to recognize malignant transformation (3). Due to their unique characteristics, during the last decades several attempts have been made to use NK cells in treating leukemia, melanoma, renal cell carcinoma, etc. (4). For this purpose, NK cells have been isolated from different sources including peripheral blood (PB), umbilical cord blood (CB), etc. (4, 5). However, since NK cells constitute approximately 10% and 20% of the lymphocyte’s population in PB and CB, the number of NK cells isolated from these sources is limited and may not be sufficient for multiple infusions (6, 7). On the contrary, NK cells have only an approximate half-life of 7 days (8), thus multiple infusions are necessary to provide long-lasting therapeutic results.
To circumvent the above-mentioned hurdles in NK cell numbers and availability, several preclinical and clinical studies have attempted to exploit hematopoietic stem cell (HSC)-derived NK cells for adoptive immunotherapy (9). For this end, previous studies have explored the anti-tumor activities of NK cells derived from various HSC sources, including bone marrow, fetal liver, and spleen (9). There is strong evidence showing that these HSCs-derived NK cell products are safe, and produce anti-tumor effects; however, consistent tumoricidal effects are yet to be seen (9). Indeed, although promising results have been obtained, but as different sources of HSCs have distinct characteristics, thus NK cells derived from different HSC sources might be different in their anti-tumor potency. Accordingly, research in this field is still ongoing to identify more clinically efficient MSCs to isolate NK cells as well as to develop potential strategies to produce functionally active higher numbers of NK cells for cancer immunotherapy (10).
2. Objectives
In this study, we adopted a protocol for the development and expansion of CD56+ NK cells from cord blood hematopoietic stem cells (CB-HSCs). Numerous studies have utilized either CD34+ HSCs from BM or CB for the differentiation of NK cells. However, in most cases, the culture systems are not applicable for large-scale use, and still some protocols reported are unable to produce high NK cell numbers.
3. Methods
3.1. Cell Lines and Hematopoietic Stem Cells Isolation
Cell lines including K562 and MCF-7 were obtained from Iranian Pasteur Institute and cultured in 10% fetal bovine serum supplemented RPMI1640 containing 1% penicillin/streptomycin, and incubated at 37°C, 5% CO2 with 95% humidity. Samples of CB were obtained from the mothers who underwent normal full-term delivery at the time of birth. A written informed consent letter has been taken under the approval of the ethical committee (IR.TUMS.HORCSCT.REC.1399.022). For HSCs isolation, CB samples were stored at room temperature and then were processed within the next 24 h. In the first step, the collected samples were used to isolate mononuclear cells (MNC) using Ficoll-Hypaque (GE Healthcare, Uppsala, Sweden) density gradient centrifugation. Then, CD34+ HSCs were isolated from MNCs using a commercial immunomagnetic bead separation kit (Miltenyi Biotech, Bergisch Gladbach, Germany) based on the instructions provided by the manufacturer. Afterward, the CD34+ cells were analyzed by flow cytometry for the expression of CD34 and CD45.
3.2. NK Cell Differentiation from Umbilical Cord Blood Stem Cells
For NK cell differentiation, the freshly isolated CD34+ HSCs from CB were cultured for 21 days (3 weeks) in the differentiation media as described elsewhere (11). In detail, CD34+ CB cells were cultured at a NOTCH ligand-coated plate in the NK cell differentiation media containing the cytokine cocktail of 25 ng/mL IL-7, 25 ng/mL stem cell factor (SCF), 25 ng/mL TPO, and 50 ng/mL IL-15 (Table 1). The cells were cultured in the differentiation media containing cytokines under incubation at 37°C, 5% CO2 in a humidified atmosphere, and were replenished with new fresh media every 3 days. After 21 days of incubation under the differentiation condition, the cells were washed and further incubated for expansion (7 days) with the addition of IL-15 and IL-2 cytokines. Finally, the differentiated NK cells were analyzed for the phenotypic features as described in the following section.
The Conditions Employed for the Differentiation and Expansion of Natural Killer Cells from Cord Blood Hematopoietic Stem Cells
Variables | Concentration (ng/mL) |
---|---|
Differentiation phase | |
SCF | 25 |
TPO | 25 |
rhIL-7 | 25 |
rhIL-15 | 50 |
Expansion phase | |
IL-15 | 50 ng |
IL-2 | 500 IU |
3.3. Phenotyping of CB-Derived NK Cells
Phenotypic analysis was done to characterize the NK cells derived from CB-HSCs. For this purpose, the following monoclonal antibodies were employed: Anti-CD3 (BioLegend), anti-CD16 (BioLegend), anti-CD56 (BioLegend), anti-NKp30 (BioLegend), anti-NKp44 (BioLegend), and anti-NKp46 (BioLegend). The cells were stained by the above-mentioned fluorochrome-conjugated mAbs under incubation at 4°C for 30 min with, washed and then were resuspended in isotone diluent (Beckman Coulter) and analyzed using the Coulter FC500 flow cytometer (Beckman Coulter). Of note, the numbers and purity of the NK cells were tested by forward and side scatters and by gating on CD45+ cells, respectively. The obtained NK cells were tested for the expression of activating receptors after differentiation (day 21) and expanding period (day 28). The CB samples were taken from 9 donors and at least a set of triplicate experiments were done for each donor.
3.4. NK Cell-Mediated Cytotoxicity Assay
The tumor lytic potential of the CB-HSC-derived-NK cells against K562 and MCF-7 cell lines was measured by XTT (Sigma) assay as described elsewhere (12, 13). In this regard, 0.25 mg/mL of XTT solution was prepared in PBS. A 0.05 mg/mL solution of (2,3)-dimethoxy-5-methyl- (1,4)-benzoquinone (coenzyme Q; Sigma) was also prepared. For each experiment, the fresh stock solutions of XTT were prepared containing 1 mL XTT with 8 μL coenzyme Q. A. Afterward, for cytotoxic tests, 1 × 104 cells/well of target cells (K562 cells and MCF-7 cells) were transferred into U-bottom 96-well culture plates and coincubated with effector cells (NK cells) with different effector to target cell ratios (20:1, 10:1, 5:1, and 1:1). Control groups of effector and target cells were also included. The cultures were incubated for 4 h and then centrifuged for 10 min by 3000 rpm. The supernatants were discarded and replaced by 150 μL of XTT stock solution containing coenzyme Q. The plates were shaken for 1 h at 37°C and then were centrifuged 10 min at 3000 rpm. Finally, 100 μL supernatant of each well was collected into another 96-well plate to measure the absorbance at 490 nm (Universal Microplate Reader, ELx 800 UV). The cytotoxic percentage was calculated using the following formula:
3.5. Apoptosis Assay
Natural killer cell-mediated apoptosis of tumor cells was tested using Annexin V-FITC/PI kit (BD, Bioscience, 556,547). The effector: Target ratio of 10: 1 was selected and the cells were incubated for 6 hours. Afterward, the flow-cytometry analysis was done to determine the percentage of apoptotic cells.
3.6. Chemokine Release Assay
The TNF-α and IFN-γ production by tumor cell-experienced NK cells in their supernatant was measured by ELISA (Pierce Endogen, Rockford, IL, USA). The absorbance of 450 nm by ELISA reader (Titertek, Huntsville, Alabama, USA) was considered as the concentration results.
3.7. Statistical Analysis
Data are presented as the mean standard deviation. The SPSS 16.0 software package was used to analyze the data. The student T-test was employed for comparing quantitative data. P-value < 0.05 was considered as significant.
4. Results
4.1. Isolation of HSC from Cord Blood
As previously stated, freshly collected cord blood, obtained within the last 48 hours, was employed for the isolation of CD34+ + HSCs. For this purpose, MNCs were isolated from cord blood cells by density gradient centrifugation and then a Miltenyi MACs kit was employed to isolate the CD34+ HSCs. The obtained HSCs were tested for the expression of CD45 and CD34, and as shown in Figure 1, the isolated HSCs were highly pure (CD34+ and CD45-).
Human cord blood hematopoietic stem cells (CB-HSCs) were isolated from MNCs derived from cord blood and examined by flow cytometry for the expression of CD34 and CD45 (FITC-A:CD45, and APC-A:CD34).
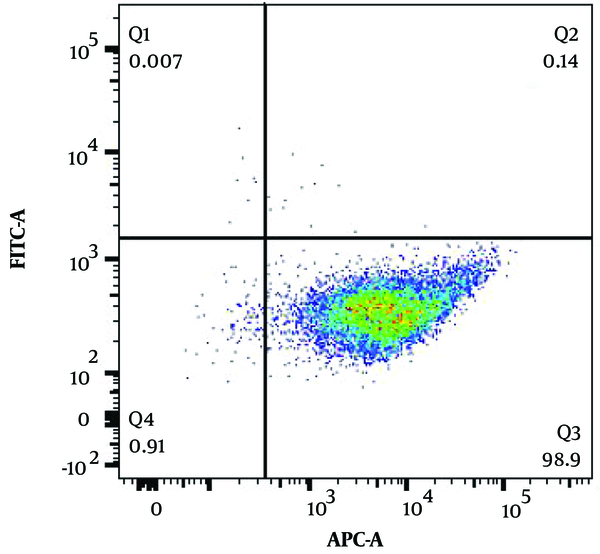
4.2. CD34+CB-HSCs Differentiate to NK Cells
The isolated HSCs from cord blood samples were then used for the NK cell differentiation. The cells were cultured under the conditions summarized in Table 1.
Enriched CD34+ HSCs were cultured in 15 ug/mL Notch ligand (Sino Biological)-coated culture plates containing the above-detailed cytokines that support differentiation and proliferation of HSC toward the NK cell lineage. The differentiated cells were also co-cultured with EBV-LCL cells for maximum expansion. At day 21 of the differentiation period, most of the obtained cells had an NK cell phenotype, which was demonstrated by flow cytometry as CD3-CD56+ (Figure 2).
The isolated hematopoietic stem cells (HSCs) were incubated under the differentiation condition media for 21 days and then were examined for natural killer (NK) cells markers (CD3 and CD56) by flow cytometry.
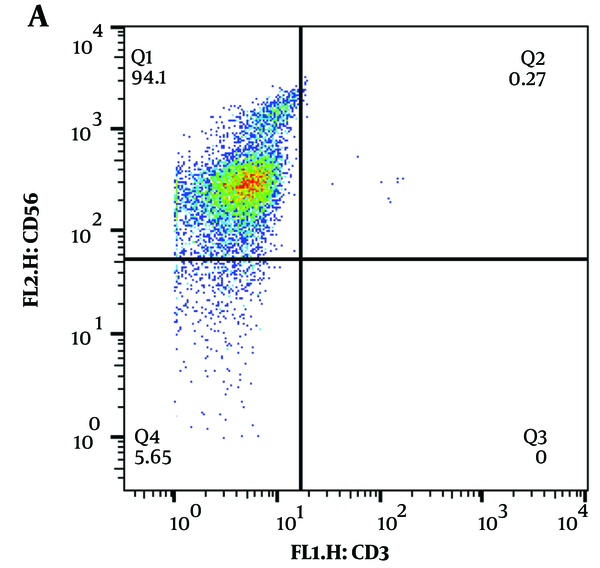
The differentiated NK cells-derived from CB-HSCs at day 21 were also tested for the expression of key NK cells activating receptors. As presented in Figure 3, there are significant differences between expanded (incubated for activation) and unexpanded (early differentiated) NK cells in terms of NKG2D, NKp30, NKp44 and NKp46, however, no significant changes were observed in the expression of the inhibitory CD158a receptor.
The expression of activating and inhibitory receptors on the natural killer (NK) cells derived from cord blood hematopoietic stem cells (CB-HSCs). Expanded (activated cells: Day 28) and unexpanded (early differentiated: Day 21) NK cells were examined by flow cytometry and the mean ± SD of at least of triplicate experiments are presented. *: P < 0.05; **: P < 0.01
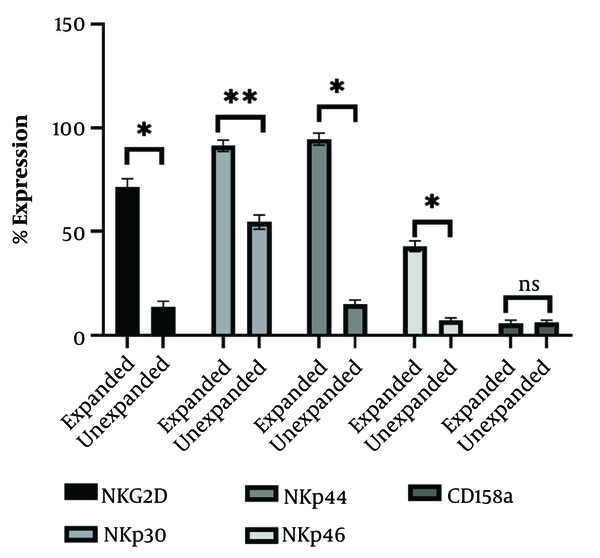
4.3. CB-HSC-Derived NK Cells Exert Anti-tumor Activities
We have also performed functional assays to test the potential anti-tumor activity of the CB-HSCs-derived NK cells. To this end, the obtained NK cells (effector cells) cocultured with the breast cancer MCF7 cells and K562 cells (target cells) at different effector: Target ratios. Based on the obtained results, CB-HSC-derived NK cells were capable of inducing cytotoxic effects on both K562 and MCF7 cells (Figure 4). We observed that with the increase of effector to target ratio (E: T) to 20:1 remarkable cytotoxic effect was achieved.
The cytotoxic activity of the cord blood hematopoietic stem cell (CB-HSC)-derived natural killer (NK) cells against K562 and MCF7 tumor cells. Different effector: Target ratios (1:1, 5:1, 10:1, 20:1) were employed in the experiments. The mean ± SD of the at least two triplicate experiments are presented.
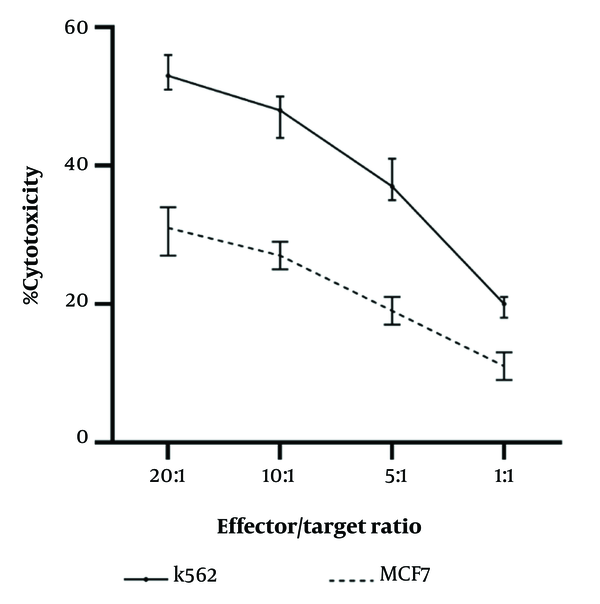
We also tested whether CB-HSC-derived NK cell-induced cytotoxicity is mediated by apoptosis. As shown in Figure 5, the Annexin V/PI assay results demonstrate that NK cells were able to kill MCF-7 cells (detected as early apoptosis 28.4%). Moreover, NK cell-induced late apoptosis rate was 12.8% in MCF-7 breast cancer cells.
Cord blood hematopoietic stem cell (CB-HSC)-derived natural killer (NK) cells induce apoptosis in MCF7 cells. Annexin V/PI testing was done and the graph was a representative of triplicate experiments. Annexin V+ cells were identified as early apoptosis, while Annexin V+/PI+ cells were defined as late apoptosis.
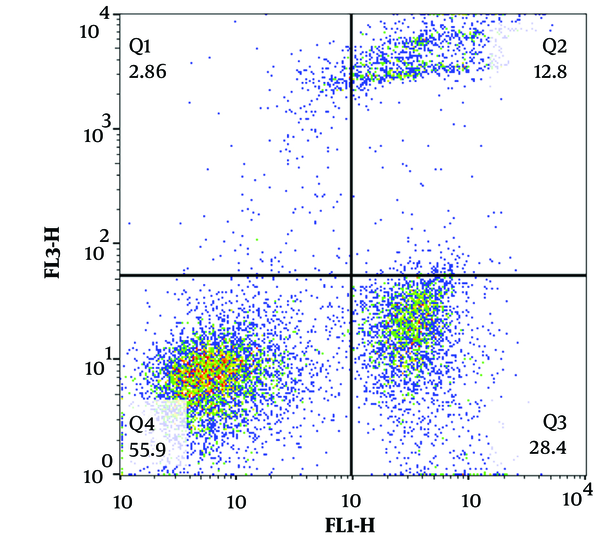
4.4. CB-HSC-Derived NK Cells Release IFN-γ and TNF-α
We also tested the expression of key cytokines released by NK cells. In this regard, CB-HSC-derived NK cells were experienced with tumor cells and then their supernatant was examined for the release of IFN-γ and TNF-α. As can be found in Figure 6, CB-HSC-derived NK cells could release significant amounts of IFN-γ and TNF-α in response to their exposure to the tumor cells.
Cytokine release by cord blood hematopoietic stem cell (CB-HSC)-derived natural killer (NK) cells. Isolated NK cells were exposed to the tumor cells and then their supernatant was examined for the release of the IFN-γ and TNF-α. The results are the mean ± SD of the triplicate experiments.
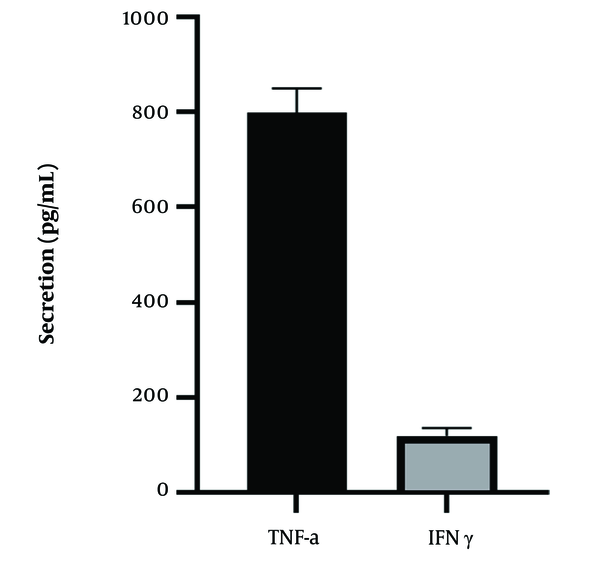
5. Discussion
Although the use of NK cells in cancer immunotherapy has gained strong attention during the last decades, attaining large-scale off-the-shelf products was the main challenge. To circumvent this obstacle in natural killer cell-based immunotherapy, numerous attempts have been made to use stem cells to produce NK cells. There are several reports in the literature that demonstrate that CB-HSC could differentiate into NK cells with potential anti-tumor activity, however different methods are described (14). Therefore, in the present study we aimed to set up a protocol to differentiate natural killer cells from umbilical cord blood haematopoietic stem cells and evaluate their anti-tumor activity against breast cancer human tumor cells.
In the present study, we employed a cytokine cocktail to differentiate CD34+CB-HSCs toward NK cells. We isolated CD34+ cells from CB with high purity (Figure 1) and then used them for the differentiation of NK cells. As shown in Table 1 and Figure 2, CD34+ cells were cultured for 21 days under the differentiation condition to produce NK cells (CD56+ CD3- cells). Our results showed that the cytokine cocktail used in this study is capable of differentiating NK cells. Previous studies have also reported that cord blood CD34+ cells can be differentiated from NK cells. For instance, Pinho et al. have employed a cocktail of IL-2, IL-3, IL-7, SCF and Flt3-L cytokines to differentiate NK cell from CB-CD34+ cells (15). They found that this cytokine cocktail can induce NK cells differentiation, however, the culture condition does not affect the expression of KIRs and NCRs (15). Others have also shown that a cocktail of cytokines including FlT3-L, SCF, IL-7 and IL-15 in combination with IL-21 or IL-12 could improve maximum differentiation of NK cells and promote their cytokine release, cytolytic function and the expression of KIRs (16). The addition of other soluble factors (TPO, G-CSF, IL-6) has also been suggested to further support precursor proliferation from CB samples (17-19). In another study, different combinations of cytokines and feeder cells were employed to differentiate NK cells from HSCs. It was found that the presence of FlT3-L, SCF, IL-7, and IL-15 as well as feeder OP9-DL1 cells could lead to the generation of NK cells (20). Regarding the previous reports and our findings it can be inferred that a cytokine cocktail could provide a condition for NK cell differentiation without a need for feeder stroma.
Although the differentiation of NK cells from CB is of importance, whether the differentiated cells are functional or not should be considered. Here, we employed both IL-15 and IL-2 to expand the differentiated NK cells. As shown in Figure 3, the cytokine-expanded NK cells express significantly elevated levels of the activating NKG2D, NKp30, NKp44 and NKp46 receptors. Similar to our findings, others have also shown that the use of expansion protocol in HSC-derived NK cells could increase their numbers as well as functionality. For instance, Spanholtz et al. revealed that expanded CD34+CB cells could easily differentiate into CD56+CD3- NK cells at least with a mean expansion rate of > 2,000 fold and more than 90% purity (18). It was also shown that the low dose of human IL-15 drives CB-NK cell expansion in vivo (21). For example, CB-NK cells treated with IL-15 were shown to potently inhibit K562 cells growth in vivo, demonstrating that IL-15 could improve the anti-tumor activity of CB-NK (21). There are reports showing that such a response can also be achieved by co-administration of NK cell and IL-2 (22, 23). Considering this, we have also included IL-2 to further improve CB-HSC-derived NK cells functionality. Previous studies have also demonstrated that the combination of IL-2 and IL-15 could robustly increase the expression of activating receptors including NKp30, NKp44 and NKp46 and NKG2D on NK cells (11). This was confirmed by our findings demonstrating that IL-15 and IL-2 treated -CB-HSCs-derived NK cells expressed higher levels of NKG2D, NKp30, NKp44 and NKp46 compared to those differentiated NK cells that were not treated for expansion.
Examining the literature, it can be conceived that IL-12 might be the best combination with IL-15, however, the results achieved are not consistent. For example, a previous study showed that the replacement of IL-2 by IL12 could enhance IFN-γ production and the anti-tumor activity of HSC-NK cells against AML cells in vitro and in vivo (24). It was also shown that IL-12 could increase the number of NKG2A- and KIRs-expressing HSC-NK cells, which are more sensitive to the stimulation of target cells (24). However, in contrast, other studies have shown that NK cells derived from CB-CD34+ and PB-CD34+ cells did not respond similarly to this cytokine, and IL-12 could not improve the cytolytic activity of the CB-CD34+-NK cells compared to those PB-CD34+NK cells (25).
We have also examined the anti-tumor effects of the NK cells derived from CB-HSCs. Two tumor cell lines, including NK-sensitive MHC-I-deficient K562 leukemia cells, and MCF7 a human breast cancer cell line, were used to examine the anti-tumor effects of the CB-HSC-derived NK cells. The results showed that the obtained NK cells could remarkably destroy tumor cells, however, the antitumor effects of the CB-HSC-derived NK cells were more obvious at higher effector-to-target rations (Figures 4 and 5). Interestingly, the CB-HSC-derived NK cells were also able to produce higher amounts of TNF-α and IFN-γ in response to exposure to K562 cells (Figure 6). Consistent with our findings, previous studies have also shown that CB-HSCs-derived NK cells release higher levels of IFN-γ and could lyse tumor cells more robustly than PB-HSCs-derived NK cells (25, 26).
5.1. Conclusions
In this study, CD34+ HSCs isolated from cord blood were tested to differentiate into NK cells. Our findings revealed that the CB-HSC-derived NK cells are capable of killing tumor cells. Interestingly, the results revealed that treatment of newly differentiated NK cells with a pair of IL-15 and IL-2 for at least 7 days could robustly increase their expression of activating NKG2D, NKp30, NKp44 and NKp46 receptors, promoting their anti-tumor activities. However, further research to set a large-scale production protocol is needed. Moreover, future research on the capability of CB-HSCs-derived NK cells to produce CAR-NK cells would also be of interest. In conclusion, our results support that CB-HSCs-derived NK cells could be considered a promising immunotherapeutic option in cancer treatment.
Acknowledgements
References
-
1.
Hosseini F, Ahmadvand M, Karimi R, Mousavi SA, Ai J, Nikbakht M. Cancer immunotherapy via stem cell-derived NK cells. Immunotherapy. 2023;15(12):963-73. [PubMed ID: 37337708]. https://doi.org/10.2217/imt-2022-0224.
-
2.
Hosseini R, Sarvnaz H, Arabpour M, Ramshe SM, Asef-Kabiri L, Yousefi H, et al. Cancer exosomes and natural killer cells dysfunction: biological roles, clinical significance and implications for immunotherapy. Mol Cancer. 2022;21(1):15. [PubMed ID: 35031075]. [PubMed Central ID: PMC8759167]. https://doi.org/10.1186/s12943-021-01492-7.
-
3.
Sivori S, Vacca P, Del Zotto G, Munari E, Mingari MC, Moretta L. Human NK cells: surface receptors, inhibitory checkpoints, and translational applications. Cell Mol Immunol. 2019;16(5):430-41. [PubMed ID: 30778167]. [PubMed Central ID: PMC6474200]. https://doi.org/10.1038/s41423-019-0206-4.
-
4.
Laskowski TJ, Biederstadt A, Rezvani K. Natural killer cells in antitumour adoptive cell immunotherapy. Nat Rev Cancer. 2022;22(10):557-75. [PubMed ID: 35879429]. [PubMed Central ID: PMC9309992]. https://doi.org/10.1038/s41568-022-00491-0.
-
5.
Heipertz EL, Zynda ER, Stav-Noraas TE, Hungler AD, Boucher SE, Kaur N, et al. Current Perspectives on "Off-The-Shelf" Allogeneic NK and CAR-NK Cell Therapies. Front Immunol. 2021;12:732135. [PubMed ID: 34925314]. [PubMed Central ID: PMC8671166]. https://doi.org/10.3389/fimmu.2021.732135.
-
6.
Kennedy PR, Felices M, Miller JS. Challenges to the broad application of allogeneic natural killer cell immunotherapy of cancer. Stem Cell Res Ther. 2022;13(1):165. [PubMed ID: 35414042]. [PubMed Central ID: PMC9006579]. https://doi.org/10.1186/s13287-022-02769-4.
-
7.
Kang S, Gao X, Zhang L, Yang E, Li Y, Yu L. The Advances and Challenges of NK Cell-Based Cancer Immunotherapy. Curr Oncol. 2021;28(2):1077-93. [PubMed ID: 33652996]. [PubMed Central ID: PMC8025748]. https://doi.org/10.3390/curroncol28020105.
-
8.
Domogala A, Blundell M, Thrasher A, Lowdell MW, Madrigal JA, Saudemont A. Natural killer cells differentiated in vitro from cord blood CD34(+) cells are more advantageous for use as an immunotherapy than peripheral blood and cord blood natural killer cells. Cytotherapy. 2017;19(6):710-20. [PubMed ID: 28428057]. https://doi.org/10.1016/j.jcyt.2017.03.068.
-
9.
Luevano M, Madrigal A, Saudemont A. Generation of natural killer cells from hematopoietic stem cells in vitro for immunotherapy. Cell Mol Immunol. 2012;9(4):310-20. [PubMed ID: 22705914]. [PubMed Central ID: PMC4012863]. https://doi.org/10.1038/cmi.2012.17.
-
10.
Zeng J, Tang SY, Toh LL, Wang S. Generation of "Off-the-Shelf" Natural Killer Cells from Peripheral Blood Cell-Derived Induced Pluripotent Stem Cells. Stem Cell Reports. 2017;9(6):1796-812. [PubMed ID: 29173894]. [PubMed Central ID: PMC5785702]. https://doi.org/10.1016/j.stemcr.2017.10.020.
-
11.
Spanholtz J, Tordoir M, Eissens D, Preijers F, van der Meer A, Joosten I, et al. High log-scale expansion of functional human natural killer cells from umbilical cord blood CD34-positive cells for adoptive cancer immunotherapy. PLoS One. 2010;5(2):e9221. [PubMed ID: 20169160]. [PubMed Central ID: PMC2821405]. https://doi.org/10.1371/journal.pone.0009221.
-
12.
Hosseini R, Asef-Kabiri L, Sarvnaz H, Ghanavatinejad A, Rezayat F, Eskandari N, et al. Blockade of exosome release alters HER2 trafficking to the plasma membrane and gives a boost to Trastuzumab. Clin Transl Oncol. 2023;25(1):185-98. [PubMed ID: 36018441]. https://doi.org/10.1007/s12094-022-02925-5.
-
13.
Hosseini Ghatar R, Soltantoyeh T, Bahadori M, Khoshnoodi J, Golsaz Shirazi F, Jeddi Tehrani M, et al. Polyclonal Antibody against Different Extracellular Subdomains of HER2 Induces Tumor Growth Inhibition in vitro. Iran J Immunol. 2017;14(3):200-14. [PubMed ID: 28919583].
-
14.
Shokouhifar A, Anani Sarab G, Yazdanifar M, Fereidouni M, Nouri M, Ebrahimi M. Overcoming the UCB HSCs -Derived NK cells Dysfunction through Harnessing RAS/MAPK, IGF-1R and TGF-beta Signaling Pathways. Cancer Cell Int. 2021;21(1):298. [PubMed ID: 34098947]. [PubMed Central ID: PMC8185927]. https://doi.org/10.1186/s12935-021-01983-z.
-
15.
Pinho MJ, Punzel M, Sousa M, Barros A. Ex vivo differentiation of natural killer cells from human umbilical cord blood CD34+ progenitor cells. Cell Commun Adhes. 2011;18(3):45-55. [PubMed ID: 21906011]. https://doi.org/10.3109/15419061.2011.610911.
-
16.
Damele L, Spaggiari GM, Parodi M, Mingari MC, Vitale M, Vitale C. Cord Blood-Derived Natural Killer Cell Exploitation in Immunotherapy Protocols: More Than a Promise? Cancers (Basel). 2022;14(18):4439. [PubMed ID: 36139598]. [PubMed Central ID: PMC9496735]. https://doi.org/10.3390/cancers14184439.
-
17.
Hoogstad-van Evert JS, Cany J, van den Brand D, Oudenampsen M, Brock R, Torensma R, et al. Umbilical cord blood CD34(+) progenitor-derived NK cells efficiently kill ovarian cancer spheroids and intraperitoneal tumors in NOD/SCID/IL2Rg(null) mice. Oncoimmunology. 2017;6(8):e1320630. [PubMed ID: 28919991]. [PubMed Central ID: PMC5593716]. https://doi.org/10.1080/2162402X.2017.1320630.
-
18.
Spanholtz J, Preijers F, Tordoir M, Trilsbeek C, Paardekooper J, de Witte T, et al. Clinical-grade generation of active NK cells from cord blood hematopoietic progenitor cells for immunotherapy using a closed-system culture process. PLoS One. 2011;6(6):e20740. [PubMed ID: 21698239]. [PubMed Central ID: PMC3116834]. https://doi.org/10.1371/journal.pone.0020740.
-
19.
Sivori S, Cantoni C, Parolini S, Marcenaro E, Conte R, Moretta L, et al. IL-21 induces both rapid maturation of human CD34+ cell precursors towards NK cells and acquisition of surface killer Ig-like receptors. Eur J Immunol. 2003;33(12):3439-47. [PubMed ID: 14635054]. https://doi.org/10.1002/eji.200324533.
-
20.
Hernandez DC, Juelke K, Muller NC, Durek P, Ugursu B, Mashreghi MF, et al. An in vitro platform supports generation of human innate lymphoid cells from CD34(+) hematopoietic progenitors that recapitulate ex vivo identity. Immunity. 2021;54(10):2417-24-e5. [PubMed ID: 34453879]. https://doi.org/10.1016/j.immuni.2021.07.019.
-
21.
Cany J, van der Waart AB, Tordoir M, Franssen GM, Hangalapura BN, de Vries J, et al. Natural killer cells generated from cord blood hematopoietic progenitor cells efficiently target bone marrow-residing human leukemia cells in NOD/SCID/IL2Rg(null) mice. PLoS One. 2013;8(6):e64384. [PubMed ID: 23755121]. [PubMed Central ID: PMC3673996]. https://doi.org/10.1371/journal.pone.0064384.
-
22.
Woll PS, Grzywacz B, Tian X, Marcus RK, Knorr DA, Verneris MR, et al. Human embryonic stem cells differentiate into a homogeneous population of natural killer cells with potent in vivo antitumor activity. Blood. 2009;113(24):6094-101. [PubMed ID: 19365083]. [PubMed Central ID: PMC2699231]. https://doi.org/10.1182/blood-2008-06-165225.
-
23.
Fujisaki H, Kakuda H, Shimasaki N, Imai C, Ma J, Lockey T, et al. Expansion of highly cytotoxic human natural killer cells for cancer cell therapy. Cancer Res. 2009;69(9):4010-7. [PubMed ID: 19383914]. [PubMed Central ID: PMC2716664]. https://doi.org/10.1158/0008-5472.CAN-08-3712.
-
24.
Cany J, van der Waart AB, Spanholtz J, Tordoir M, Jansen JH, van der Voort R, et al. Combined IL-15 and IL-12 drives the generation of CD34(+)-derived natural killer cells with superior maturation and alloreactivity potential following adoptive transfer. Oncoimmunology. 2015;4(7):e1017701. [PubMed ID: 26140247]. [PubMed Central ID: PMC4485802]. https://doi.org/10.1080/2162402X.2015.1017701.
-
25.
Luevano M, Domogala A, Blundell M, Jackson N, Pedroza-Pacheco I, Derniame S, et al. Frozen cord blood hematopoietic stem cells differentiate into higher numbers of functional natural killer cells in vitro than mobilized hematopoietic stem cells or freshly isolated cord blood hematopoietic stem cells. PLoS One. 2014;9(1):e87086. [PubMed ID: 24489840]. [PubMed Central ID: PMC3906137]. https://doi.org/10.1371/journal.pone.0087086.
-
26.
Perez SA, Mahaira LG, Sotiropoulou PA, Gritzapis AD, Iliopoulou EG, Niarchos DK, et al. Effect of IL-21 on NK cells derived from different umbilical cord blood populations. Int Immunol. 2006;18(1):49-58. [PubMed ID: 16291655]. https://doi.org/10.1093/intimm/dxh348.