Abstract
Background:
Combination cancer therapy is a promising strategy which employs multiple therapeutic agents with different mechanisms of action along with minimal intolerable side effects. For example, a combination of radiotherapy with gene therapy can overcome the development of resistance to therapeutic doses of irradiation (IR) and normal tissue damages caused by high-dose radiation. Recent studies have revealed radio-resistance in non-small cell lung cancer (NSCLC) cells. In this study, for the first time, subunit B of cytolethal distending toxin (cdtB)-expressing plasmid was introduced as a sensitizer of the cells to IR with a high efficacy.Methods:
A vector expressing cdtB suicide gene of human periodontal bacterium Aggregatibacter actinomycetemcomitans was constructed and then transfected into A549 cell line. In the next step, cells transfected with pcDNA3.1/cdtB were irradiated and its growth inhibitory effect was evaluated in NSCLC cancer in vitro by MTT (3-(4, 5-methylthiazol-2-yl) -2, 5-diphenyl-tetrazolium bromide) assay. Terminal deoxyribonucleotidyl transferase-mediated dUTP nick end labeling (TUNEL) assay were carried out in order to examine the apoptosis induction by a combination of IR with cdtB.Results:
Our data indicated significant cell death in NSCLC cells in comparison with controls with an increase from 5% in response to IR up to 73.27% for combination of IR with cdtB. Moreover, the result of TUNEL assay showed significant differences in the number of apoptotic cells among the different affected groups.Conclusions:
Our results confirmed that cdtB-expressing plasmid sensitizes NSCLC cells to IR and significantly increases the efficacy of radiotherapy and therefore, combining toxin with IR has a synergistic effect on NSCLC.Keywords
Combination Cancer Therapy Radioresistance Suicide Gene Synergistic
1. Background
Radiation therapy or radiotherapy is a local treatment that uses ionizing radiation for cancer management (1). The first use of radiation therapy for cancer treatment was reported in the late 19th century (2). More than half of all cancer patients worldwide receive radiotherapy as part of their treatment (3).
However, there is a wide variety of clinical practices from different patients with various types or even with the same type of tumors. In spite of progress in radiation therapy, there remain problems for anticancer therapy such as development of resistance to therapeutic doses of irradiation and normal tissue damages caused by high-dose radiation (4). One of the methods used to overcome this obstacle is a combination of radiotherapy with biological therapy including gene therapy. Radiation-gene therapy developed in recent years is coupling therapeutic gene with radiation (5).
Concept of gene therapy involves the transfer and expression of genetic material into cells for the therapeutic purposes (6). Gene therapy may lead to additional radio-sensitization or cell killing.
There are three different strategies of gene therapy including the replacement of a defective tumor suppression gene, transfer of a gene that produce selective cytotoxicity and the delivery of a gene to stimulate the immune system (4). Combined radiotherapy and gene therapy enhance antitumor effects through different ways including improving transfection/ transduction efficiency, transgene integration. On the other hand, gene therapy, may interfere with repair of radiation-induced DNA damage and increase DNA susceptibility to radiation damage in cancer cells. In addition, each of them targets various stages of the cell cycle (4).
Cytolethal distending toxin (CDT) is a conserved bacterial genotoxin which induces DNA damage and causes G2/M cell cycle arrest (7). Moreover, “M” and “G2” phases are most radiosensitive phases and thereby suggesting CDT as a potent agent in sensitizing of cells to IR (4). CDT is the first bacterial toxin that induces DNA single strand breaks (SSBs) and double strand breaks (DSBs). This toxin is produced by several gram negative bacteria, such as Escherichia coli (EcCDT), Aggregatibacter actinomycetemcomitans (AaCDT), Haemophilus ducreyi (HdCDT), Shigella dysenteriae, Campylobacter jejuni (CjCDT), and enterohepatic Helicobacter cinaedi. The members of the CDT family are A-B2-type exotoxins composed of cdtA, cdtB, and cdtC subunits which are encoded by a single operon with three genes. CdtB is the enzymatically active subunit of CDT (8).
Since non-small cell lung cancer (NSCLC) is close to 85 percent of lung tumors (9) and relatively resistant to radiotherapy, combination of various therapeutic agents with different mechanisms of action has been extensively used in the management of many types of solid malignancies including NSCLC (1, 10, 11). Hence, in this study, we investigate the effects of Gene therapy with bacterial CDT sequence of Aggregatibacter actinomycetemcomitans on radio-resistant A549 cells. The results of the study indicated that CDT can overcome radiation resistance of A549 cells by damaging the DNA of cancer cells and interfering with cell cycle progression similar with radiotherapy. Therefore, this combination therapy can be developed as a unique treatment for radio-resistance NSCLC.
2. Methods
2.1. Construction of Plasmid With cdtB Gene
In order to build recombinant plasmid, XhoI and XbaI double digestion was performed to cut CdtB fragment and pcDNA3.1+basic plasmid, followed by ligating into the recombinant expression vector, pcDNA3.1+/cdtB. Ampicillin resistance screening was performed to screen the recombinant plasmid, followed by plasmid extraction using an Accuprep®Nano-Plus plasmid mini extraction kit (BIONEER, Korea) and confirmed by DNA sequencing. Recombinant plasmids containing the cdtB under control of promoter, the universal viral cytomegalovirus, was used for expression of exogenous cdtB in lung cancer cell line.
2.2. Cell Cultures and Transfection
A549 Cell line was purchased from the Iranian biological resource center (IBRC), Tehran, Iran. The cells were cultured in DMEM/F12 medium (Gibco) supplemented with 5% fetal bovine serum (FBS), 100 units/mL penicillin, and 100 μg/mL streptomycin (Sigma, USA), and maintained at 37°C in a humidified atmosphere with 5% CO2. Cells with 80% confluency were transfected with Lipofectamine 2000 (Invitrogen), according to the protocol provided by the manufacturer. Briefly, DNA and Lipofectamine 2000 were diluted in 50 μL DMEM/F12 medium without FBS, separately and then were incubated for 5 minutes at room temperature. Mixtures were combined and incubated at room temperature. After 20 minutes, complexes were added to the cells containing DMEM/F12 medium without FBS and incubated for 12 hours. Following incubation, the medium was replaced with fresh growth medium (including FBS).
After a 24 hours transfection, target cells were irradiated and then the efficiency of combination therapy in inhibition of the cells was investigated by MTT and tunel assays.
2.3. Ionizing Radiation
Target cells were irradiated at room temperature in ambient air using the ELEKTA Linear Accelerator which generates high energy X-rays at the indicated doses (2 Gy). Sham-irradiated cells were handled parallel with irradiated cells. The plate was placed on a water phantom that delivers the exact doses. The height of the culture medium in the plate was 0.5 cm and therefore the cells attached to the plate were at a depth of 0.5 cm. The filed size was 20cm × 15 cm and source to medium surface distance was 100 cm.
2.4. MTT Assay
To examine the effect of pcDNA3.1+/cdtB and irradiation on the viability of A549 cells, the cell viability was assessed by ability of viable cells to reduce 3-(4, (5-dimethylthiasol-2-yl)-2, 4,-diphenyltetrazolium bromide) (MTT).
After incubation, 10 μL aliquots of MTT solution (5 mg/mL in PBS) were added to each well and re-incubated for 3 hours at 37°C. After completing the incubation, the supernatants were carefully aspirated and 100 μL aliquots of dimethylsulfoxide (DMSO) were added to each well to dissolve the formazan crystals. The culture plates were placed on an ELISA (enzyme-linked immunosorbent assay) reader and the absorbance was measured at 570 nm. The amount of color produced is directly proportional to the number of viable cells. All assays were performed in three replicates for each pcDNA3.1+/cdtB, pcDNA3.1+/cdtB+ with irradiation, irradiation and control, and then means ± SD values were calculated. The percentage of reduction of cell proliferation was calculated according to the following formula: cell inhibition (%) = (1-A570of treated cells/A570 of control cells) × 100%.
Cells incubated in culture medium alone served as a control for cell viability (untreated wells).
Terminal deoxyribonucleotidyl transferase-mediated dUTP nick end labeling (TUNEL) assay.
TUNEL method was used for measuring 3’-hydroxyI ends of DNA fragments formed during programmed cell death using a DeadEnd™ Colorimetric TUNEL System (Promega Corporation, Madison, WI, USA). Both treated and control cells were added to the poly-L-lysine-coated slides and were fixed in 4% paraformaldehyde at room temperature and then TUNEL-staining method was performed according to the manufacturer’s instructions. Coverslips were observed with a light microscope at a magnification of 40 ×.
2.5. Statistical Analysis
Student t-test was carried out using the SPSS 23.0 software (SPSS Inc., Chicago, USA) for comparisons among average percentages of different groups with untreated cell.
All values are expressed as means ± standard deviations. P value of < 0.05 is considered to be statistically significant.
3. Results
3.1. Cdt B Increases the Sensitivity of A549 Cells to IR and Induces Apoptosis
Our data demonstrated that cdtB-expressing plasmid synergistically enhanced the sensitivity of A549 cells to IR. Firstly, the cells were transfected with pcDNA3.1/cdtB and then irradiated. The viability was determined using MTT assay. The cell inhibition in response to IR at 2 Gy for the cells and that of cells transfected with cdtB-expressing plasmid were 5% and 51%, respectively while in case the cells were treated with a combination of IR with cdtB, the cell inhibition significantly increased to 73.27%. Nevertheless, combination therapy showed the better efficacy than irradiated cell alone (P < 0.001). These results are shown in Figure 1.
Inhibition of A549 Cell Proliferation by Combination Therapy. Cell Viability Was Determined by MTT Assay Which Significantly Reduced in pcDNA3.1+/cdtB Combined with IR Group Compared to IR Alone, Data Are Presented as Mean ± SD (Normalized to Control Cells ) Graph Showing % cell Inhibition.***: P < 0.001
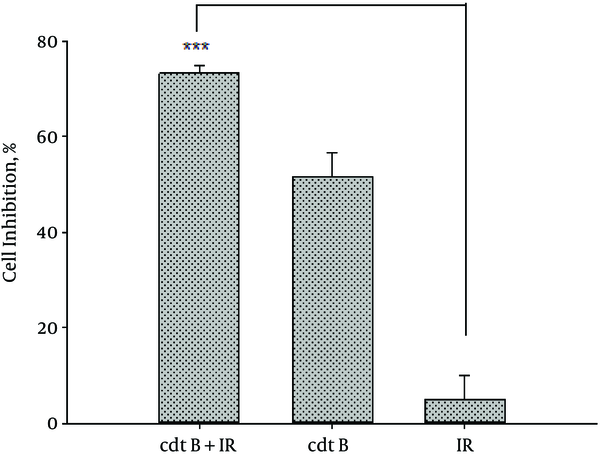
Apoptosis induction by a combination of IR with cdtB was analyzed using terminal deoxynucleotidyl transferase dUTP nick end labeling (TUNEL) staining in A549 cells.
As shown in Figure 2, the comparison of the treated cells using a combination of cdtB and IR with untreated cells and IR group cells as control by the TUNEL staining clearly shows differences among the groups. Apoptotic cells were not observed in the controls. Unlike control groups, after treating with a combination of IR with cdtB, the apoptotic bodies (brown nuclei stained) were significantly presented among the cells.
Apoptosis Induction in A549 by Combination Therapy: DNA Fragmentation Was Examined, Using an in Situ Cell Death Detection Assay (TUNEL). In Contrast to the Untreated cells and Irradiated cell, Apoptotic Bodies (Brown Nuclei Stained) Were Seen in the Treated Cells with a Combination of IR with cdtB
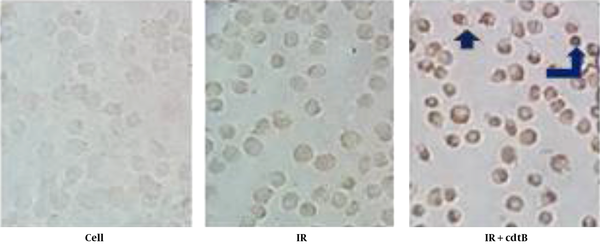
4. Discussion
In the current study, we utilized a novel cdtB-expressing plasmid constructed of a CDT bacterial genotoxin secreted by Aggregatibacter actinomycetemcomitans for inducing apoptosis and inhibiting proliferation of A549 cell line by combining with radiation and for overcoming radio-resistance NSCLC. The suicide vector was transferred onto A549 cells and the expression of suicide gene was successfully confirmed at mRNA level and then the potent cytotoxicity of the combination treatment was confirmed by the results of the MTT and Tunel assays.
Lung cancer with 26% of all female cancer deaths and 28% of all male cancer deaths is the most common cause of cancer death. NSCLC is the dominant type of lung cancer (12, 13). Most often, patients suffer from advanced disease with distant metastasis at time of common diagnosis and therefore chance of surgical resection will be reduced. The radiotherapy or radiochemotherapy is the main therapy (14); however, NSCLCs are of the greater attention with respect to high tolerance to radiotherapy in comparison with SCLC (15). Anticancer agents such as 5-fluorouracil and gemcitabine that interfere with the metabolism of nucleic acids are extensively used to overcome the problem of radio resistance, although significant side effects associated with these anti-cancer drugs are also frequently reported (16).
In this regard, bacterial toxin which causes DNA damage, may have radiosensitizing effects on tumor cell lines. The CDT family have been widely studied for their biomedical applications in cancer therapy. For example, CdtB toxin, produced by cdtB-expressing plasmid followed by sonoporation induced growth inhibition of a human gingival squamous carcinoma cell line (17). In another study, chitosan/heparin nanoparticle-encapsulated CdtB enhanced cell-cycle arrest at G2/M, followed by apoptosis, but not in primary gastric epithelial cells (18).
In addition, anthrax toxin system combined with the enzymatic activity of CdtB efficiently killed various human tumor cells and showed very low toxicity in mouse experiments and impressive antitumor effects in a lung carcinoma model, with a high cure rate (19).
CDT blocks cell proliferation and induces a cell cycle arrest depending on the DNA damage response (DDR) (8). Recently, Chih-Ho Lai et al, have reported that CDT derived from the C. jejuni effectively induce a significant therapeutic effect on radio resistant prostate cancer (PCa) cells by which it can enhance the sensitivity of PCa to radiotherapy (20).
The results of aforementioned studies along with our results confirmed that cdtB-expressing plasmid can sensitize these cells to IR and therefore combining toxin with IR has a synergistic effect on NSCLC. It is believed that cdtB-expressing plasmid may potentially be combined with radiotherapy for synergistic effect which can be used in the treatment of NSCLC. Moreover, targeted gene therapy strategy using specific promoter will protect healthy cells and reduce its side effects. However, in vivo studies will be necessary for confirming the conclusions of the in vitro analyses.
Acknowledgements
References
-
1.
Gupta S, Koru-Sengul T, Arnold SM, Devi GR, Mohiuddin M, Ahmed MM. Low-dose fractionated radiation potentiates the effects of cisplatin independent of the hyper-radiation sensitivity in human lung cancer cells. Mol Cancer Ther. 2011;10(2):292-302. [PubMed ID: 21216938]. https://doi.org/10.1158/1535-7163.MCT-10-0630.
-
2.
Connell PP, Hellman S. Advances in radiotherapy and implications for the next century: a historical perspective. Cancer Res. 2009;69(2):383-92. [PubMed ID: 19147546]. https://doi.org/10.1158/0008-5472.CAN-07-6871.
-
3.
Delaney G, Jacob S, Featherstone C, Barton M. The role of radiotherapy in cancer treatment: estimating optimal utilization from a review of evidence-based clinical guidelines. Cancer. 2005;104(6):1129-37. [PubMed ID: 16080176]. https://doi.org/10.1002/cncr.21324.
-
4.
Teh BS, Aguilar-Cordova E, Vlachaki MT, Aguilar L, Mai WY, Caillouet J, et al. Combining radiotherapy with gene therapy (from the bench to the bedside): a novel treatment strategy for prostate cancer. Oncologist. 2002;7(5):458-66. [PubMed ID: 12401909]. https://doi.org/10.1634/theoncologist.7-5-458.
-
5.
Kaliberov SA, Buchsbaum DJ. Chapter seven--Cancer treatment with gene therapy and radiation therapy. Adv Cancer Res. 2012;115:221-63. [PubMed ID: 23021246]. https://doi.org/10.1016/B978-0-12-398342-8.00007-0.
-
6.
Rabkin SD, Mineta T, Miyatake S, Yazaki T. Gene therapy: targeting tumor cells for destruction. Hum Cell. 1996;9(4):265-76. [PubMed ID: 9183657].
-
7.
Grasso F, Frisan T. Bacterial Genotoxins: Merging the DNA Damage Response into Infection Biology. Biomolecules. 2015;5(3):1762-82. [PubMed ID: 26270677]. https://doi.org/10.3390/biom5031762.
-
8.
Bezine E, Vignard J, Mirey G. The cytolethal distending toxin effects on Mammalian cells: a DNA damage perspective. Cells. 2014;3(2):592-615. [PubMed ID: 24921185]. https://doi.org/10.3390/cells3020592.
-
9.
Dong JC, Gao H, Zuo SY, Zhang HQ, Zhao G, Sun SL, et al. Neuropilin 1 expression correlates with the Radio-resistance of human non-small-cell lung cancer cells. J Cell Mol Med. 2015;19(9):2286-95. [PubMed ID: 26147006]. https://doi.org/10.1111/jcmm.12623.
-
10.
Sak A, Wurm R, Elo B, Grehl S, Pottgen C, Stuben G, et al. Increased radiation-induced apoptosis and altered cell cycle progression of human lung cancer cell lines by antisense oligodeoxynucleotides targeting p53 and p21(WAF1/CIP1). Cancer Gene Ther. 2003;10(12):926-34. [PubMed ID: 14712319]. https://doi.org/10.1038/sj.cgt.7700649.
-
11.
Brattstrom D, Bergqvist M, Hesselius P, Wagenius G, Brodin O. Different fraction schedules and combinations with chemotherapy in radiation treatment of non-small cell lung cancer. Anticancer Res. 2000;20(3B):2087-90. [PubMed ID: 10928157].
-
12.
Jemal A, Siegel R, Ward E, Murray T, Xu J, Smigal C, et al. Cancer statistics, 2006. CA Cancer J Clin. 2006;56(2):106-30. [PubMed ID: 16514137]. https://doi.org/10.3322/canjclin.56.2.106.
-
13.
Siegel R, Naishadham D, Jemal A. Cancer statistics, 2013. CA Cancer J Clin. 2013;63(1):11-30. [PubMed ID: 23335087]. https://doi.org/10.3322/caac.21166.
-
14.
Hansen HH. Treatment of advanced non-small cell lung cancer. BMJ. 2002;325(7362):452-3. [PubMed ID: 12202311]. https://doi.org/10.1136/bmj.325.7362.452.
-
15.
Zhang WC, Shyh-Chang N, Yang H, Rai A, Umashankar S, Ma S, et al. Glycine decarboxylase activity drives non-small cell lung cancer tumor-initiating cells and tumorigenesis. Cell. 2012;148(1-2):259-72. [PubMed ID: 22225612]. https://doi.org/10.1016/j.cell.2011.11.050.
-
16.
Meike S, Yamamori T, Yasui H, Eitaki M, Matsuda A, Inanami O. 8-Aminoadenosine enhances radiation-induced cell death in human lung carcinoma A549 cells. J Radiat Res. 2011;52(4):456-63. [PubMed ID: 21785234]. https://doi.org/10.1269/jrr.10188.
-
17.
Iwanaga K, Tominaga K, Yamamoto K, Habu M, Maeda H, Akifusa S, et al. Local delivery system of cytotoxic agents to tumors by focused sonoporation. Cancer Gene Ther. 2007;14(4):354-63. [PubMed ID: 17273182]. https://doi.org/10.1038/sj.cgt.7701026.
-
18.
Lai CK, Lu YL, Hsieh JT, Tsai SC, Feng CL, Tsai YS, et al. Development of chitosan/heparin nanoparticle-encapsulated cytolethal distending toxin for gastric cancer therapy. Nanomedicine (Lond). 2014;9(6):803-17. [PubMed ID: 24024568]. https://doi.org/10.2217/nnm.13.54.
-
19.
Bachran C, Hasikova R, Leysath CE, Sastalla I, Zhang Y, Fattah RJ, et al. Cytolethal distending toxin B as a cell-killing component of tumor-targeted anthrax toxin fusion proteins. Cell Death Dis. 2014;5. e1003. [PubMed ID: 24434511]. https://doi.org/10.1038/cddis.2013.540.
-
20.
Lai CH, Chang CS, Liu HH, Tsai YS, Hsu FM, Yu YL, et al. Sensitization of radio-resistant prostate cancer cells with a unique cytolethal distending toxin. Oncotarget. 2014;5(14):5523-34. [PubMed ID: 25015118]. https://doi.org/10.18632/oncotarget.2133.