Abstract
Background:
Colorectal cancer (CRC) is one of the most prevalent cancers in the world. Cancer stem cells (CSC) have been reported in many human tumors and are thought to be responsible for tumor initiation, therapy resistance, progression, relapse, and metastasis. Recent studies revealed that microRNAs (miRNA) play important roles in maintaining stemness of embryonic stem cells and CSCs.Objectives:
With regard to the crucial role of cancer stem cells in colon cancer initiation and maintenance, the miRNA expression profile in primary and cell line cancer stem cells compared to non-stem cells cancer cells were evaluated.Methods:
In this study, a population of colon CSCs from primary colon cancer and human HT-29 colonic adenocarcinoma cell line was isolated from serum free medium and their microRNA profiles were evaluated using miRNA PCR array.Results:
The isolated cells with high expression of EPCAM+/CD44+ markers showed greater colony-forming efficiency and higher tumorigenic potential. Furthermore, expression of ‘‘stemness’’ genes including C-myc, Klf4, Nanog, Sox2 and Oct4 was higher in isolated cancer stem cells. Moreover, miRNA expression profile of colon CSCs was performed using miRNA PCR array. There are 39 differentially expressed miRNAs, 24 of which had lower mean expression in the cancer stem cells samples. By contrast, 15 miRNAs had higher expression levels in CSCs samples. Of these, miR-495, miR-125, and miR-199a were the most significantly up-regulated miRNAs.Conclusions:
Our results suggest that miRNAs might play important roles in maintenance and regulation of colon CSCs and specific miRNA expression signatures may contribute to cancer initiation and expansion.Keywords
1. Background
Colorectal cancer (CRC) is the third most common cause of cancer-related deaths in the world (1). The incidence of CRC is increasing in a number of Asian countries such as Iran during recent years (2, 3). Despite significant improvements in clinical therapy, most patients still die due to therapeutic resistance and recurrence of disease.
Cancer stem cells are unspecialized cells defined by two functional characteristics including stemness maintenance and multipotency. Some evidence indicates that “cancer stem cells” may be involved in tumor maintenance, therapeutic resistance, tumor progression, and distant metastasis. The CSCs have been identified in several solid tumors, including CRC (4). Despite their potential clinical significance, regulation of intrinsic CSC properties at the molecular level is poorly understood.
MicroRNAs (miRNAs) are a class of small noncoding RNAs that regulate gene expression by binding to 3’ un-translated regions of the complementary mRNA. This interaction leads to mRNA degradation or inhibition of protein synthesis (5). Previous studies have shown that miRNAs participate in several biological functions including development, cellular proliferation, differentiation and apoptosis. Moreover, new evidence has suggested that miRNAs could be involved in maintaining stemness of embryonic stem cells and CSCs. To date, several studies have described the role of miRNAs in cancer stem cell of lung, breast, hepatocellular, gastric, prostate, pancreatic, and Glioma (6). Experimental facts have suggested that some of the miRNAs including miR-126 (7), miR-181 (8), miR-372, miR-373 (9), miR-17-92 (10, 11), miR-21 and miR-205 (12) regulate the key properties of cancer stem cells including cell-cycle exit and differentiation, pro-survival and anti-stress mechanisms, epithelial-mesenchymal transitions (EMT) and migration and invasion.
Recent data suggest that miRNAs could implicate in sustaining stemness of colorectal CSCs; however, the role of miRNAs in human colorectal CSCs (CRCSCs) is still poorly understood. Investigation of miRNA expression profiles in colon CSCs and their potential role is crucial for elucidating characters of colon CSCs, which will help to develop anticancer drugs or novel therapeutic methods to target CSCs.
2. Objectives
The aim of this study was to investigate the miRNA expression profile in cancer stem cells compared to non-stem cells cancer cells
3. Methods
3.1. Tumor Cell Preparation
Colon cancer tissues were obtained from 3 patients (stage II, female, age range 58 - 65 years) after signing informed consent according to the ethics committee of Tarbiat Modares University. The histological diagnosis was based on microscopic features of carcinoma cells by expert pathologist that determines the histological types and grades of tumors. The studied patients did not receive any form of neoadjuvant therapy prior to surgery. Cancer tissues were intensively washed in PBS solution contains antibiotics and incubated overnight in DMEM/F12 (GIBCO) supplemented with penicillin (500 U/mL), streptomycin (500 mg/mL) (Invitrogen), and amphotericin B (1.25 mg/mL) (Sigma). Tumor tissue was mechanically and enzymatically dissociated after 2-hour incubation with Collagenase Type IV (1.5 mg/mL) (Invitrogen) at 37°C. Then, the single cells were used to be cultured in DMEM/F12 supplemented with 10% fetal bovine serum (FBS, GIBCO), to obtain primary colon cells, considered as parent cells and to be cultured in stem cell medium DMEM/F12 to obtain spheroids.
3.2. Cell Line and Culture Conditions
The human colonic adenocarcinoma cell line HT-29 was purchased from Iranian biological resource center (IBRC). Cells were cultured in DMEM/F-12 supplemented with 10% FBS and 1% penicillin/streptomycin in a humidified incubator at 37°C and 5% CO2. HT-29 Cells were cultured in completed media considered as parent cells. Cells were suspended using trypsinization (0.25% trypsin/EDTA (GIBCO) for 3 - 5 minutes).
3.3. Generation of Colonospheres
Single-cell suspensions from primary tumors and HT-29 cell line were cultured in ultra-low attachment 6 well plates in stem cell medium DMEM/F12 supplemented with 6 mg/mL Glucose, 5 mM HEPES, 4 mg/mL Heparin, 1%B27 (50X, invitrogen) , 1% (w/v) of non-essential amino acids (GIBCO), 1% (w/v) of L-glutamine (GIBCO), 10 ng/mL bFGF, 20 ng/mL EGF, 1% (w/v) of sodium pyruvate, 100 mg/mL apotransferrin, 25 mg/ml insulin, 9.6 mg/mL putrescin, 30 nM sodium selenite, and 20 nM progesterone (Sigma-Aldrich) 1% penicillin/streptomycin (GIBCO) to obtain spheroids. To evaluate the differentiation potential of stem cell-like cells, spheroids were cultured in DMEM/F12 supplemented with 10% FBS. All cell culture was carried out at 37°C in a 5% CO2 humidified incubator.
3.4. Clonogenic Assay
For colony formation assay, single-cell suspensions from colonospheres and parent cell-derived primary and HT-29 cells were seeded out at density of 500 cells/mL in a 12-well plate to form colonies for 5 - 7 days. Colonies were fixed with glutaraldehyde (6.0% v/v), stained with crystal violet (0.5% w/v) and colonies with over 50 cells were manually counted using a stereomicroscope.
3.5. Flowcytometry
Single cell suspension from colonospheres and parent cell-derived primary and HT-29 cells were stained according to protocol. Briefly, cells were detached by trypsin and re-suspended in PBS containing 1% BSA. After 10 minutes, cells were labeled with anti-human/mouse CD44-APC (eBioscience) and anti-human EPCAM-PE (Abcam). The cells stained with rat IgG 2b isotype control APC and mouse IgG 1 isotype control PE served as gating control. Flow-cytometry analysis was performed using FACS CantoII.
3.6. RNA Extraction and Quantitative Real-Time RT-PCR
Total RNA was extracted from the parent and colonosphere-derived primary, and HT-29 cells using high pure RNA isolation kit (Roche) according to the manufacturer’s instructions. Quantitative reverse transcriptase PCR was performed using light cycler RNA Master SYBR Green I (QIAGEN) on light cycler Roche version 3.5. The following genes were tested: c-myc, Klf4, Nanog, Sox2 and Oct4. The primer sequences and PCR conditions are summarized in Table 1. The relative amount of each mRNA was normalized to HGPRT. The fold-change from colonospheres relative to the control (parent) was calculated using 2-ΔΔCtmethod.
The Primer Sequences and PCR Conditionsa
Gene Name | Primer Sequences |
---|---|
c-myc | F: 5'-AGCGACTCTGAGGAGGAAC-3' |
R:5'-CTGCGTAGTTGTGCTGATG-3' | |
Sox-2 | F:5'-GGACTGAGAGAAAGAAGAGGAG-3' |
R:5'-GAAAATCAGGCGAAGAATAAT-3' | |
Oct-4 | F:5'-CGCCGTATGAGTTCTGTG-3' |
R: 5'-GGTGATCCTCTTCTGCTTC-3' | |
Nanog | F:5'-GCTAAGGACAACATT GATAGAAG-3' |
R:5'-CTTCATCACCAATTCGTACTTG-3' | |
Klf-4 | F: 5'-CCCAATTACCCATCCTTCC-3' |
R:5'-GTGCCTGGTCAGTTCATC-3' | |
HGPRT | F:5'-CCTGGCGTCGTGATTATGG-3' |
R:5'-TCAGTCCTGTCCATAATTAGTCC-3' |
3.7. In Vivo Xenografts Assay
To investigate whether tumor spheroid cells retain tumorigenic potential, single-cell suspensions from colonosphere and parent-derived primary and HT-29 cells were subcutaneously injected to 6 to 8 week-old nude mice. Cells were prepared at 1:1 ratio of PBS: collagen in a total volume of 100 µL, before injection. Mice were purchased from animal laboratory of Imam Khomeini Hospital and were maintained under standard conditions according to the guidelines of the animal care committee of cancer institute. In vivo studies were approved by the Animal ethics committee, Tarbiat Modares University. A portion of the tumor tissues was fixed in 10% formaldehyde and stained with H&E for histopathological examination.
3.8. MiRNA PCR Array
Total RNA from spheroid and parental cells-drived primary and cell line was extracted using Trizol (Invitrogen) and cDNA was synthesized by Universal cDNA Synthesis Kit II (Exiqon , Denmark ) according to the manufacturer’s instructions. MicroRNA expression profiling was performed using real-time PCR in 384-well format by the miRCURY LNA™ Universal RT MicroRNA PCR kit (Exiqon, Denmark) on Roche LC480. Expression levels of the microRNAs were measured using the comparative Ct method and normalized by miR-199a as endogenous control.
3.9. Statistical Analysis
Data are presented as means ± standard error of the mean (SEM). Statistical analysis was performed using SPSS 15 and GraphPad Prism 3.0. The paired t-test was used to analyze the median value of expression between the groups. Statistical significance was determined at P < 0.05. All tests were performed in triplicate.
4. Results
4.1. Generation and Validation of Spheroid Cells
According to previous experiments, we showed that single cells from primary tumors and HT-29 cell line formed spheroid colonies under serum free condition or stem cell media (13, 14) (Figure 1A). To investigate the capability of colon spheres differentiation, these cells were cultivated in 10% FBS medium. After one day, floating undifferentiated cells were attached to the plastic, gradually migrating from tumor spheres and differentiated into large and adherent cells.
Generation and Characterization of Spheroid Cells From Primary Tumors and HT-29 Cell Line
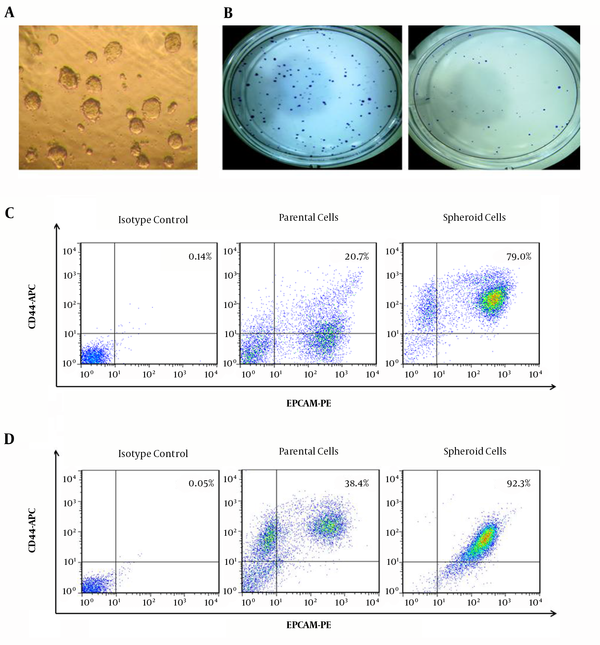
Spheroid cells expressed higher levels of EpCAM and CD44 molecules in comparison to parent cells. The results have demonstrated that 92% of tumor spheroid cells-derived HT-29 cell line were CD44+/EpCAM+, while matched parent cells only expressed 38% of CD44+/EpCAM+ markers. In case of the primary tumor, 79% of spheroid cells were positive for CD44/EPCAM, while 20% of parental cell showed CD44+/EPCAM+ (Figure 1C and 1D). These results suggested that colonospheres formed by the colon cancer cells have enriched population of CSCs.
To examine the clonogenic potential of the spheroid and parent cells from both primary and cell line, we plated and cultured the different cell population at clonal density for 7 days. The results of experiments indicated that spheroid cells possess a higher clonogenic activity than parent cells (P < 0.05). Our data showed that colony formation ability of spheroid cells from primary tumor was 185 ± 19 colonies/well vs. 76 ± 8 colonies/well parent cells (Figure 1B). Similar results were observed in colony formation ability of the spheroid derived HT-29 (196 ± 13 colonies/well vs. 58 ± 6 colonies/well) as compared with parent cells.
4.2. Spheroid Cells Exhibit Stemness Properties
To evaluate the stemness properties, expression level of markers related to stem cell pathways including Klf4, Sox2, Nanog, C-myc and Oct4 were determined by RT-PCR in both spheroid and parent cells. As shown in Figure 2, spheroid cells derived primary and cell line expressed high levels of stemness genes as compared to parent cells (P < 0.05). Primary tumor derived spheroid cells also showed significantly increased level of these genes compared with the cell line derived spheroid cell (Figure 2).
A. Stemness Genes Expression of Spheroid and Parental Cells in Primary Tumors and B, HT-29 Cell Line; Assessed by Real-Time PCR *P < 0.05 (A) C-myc (B) Klf4 (C) Nanog (D) Oct4 (E) Sox2.
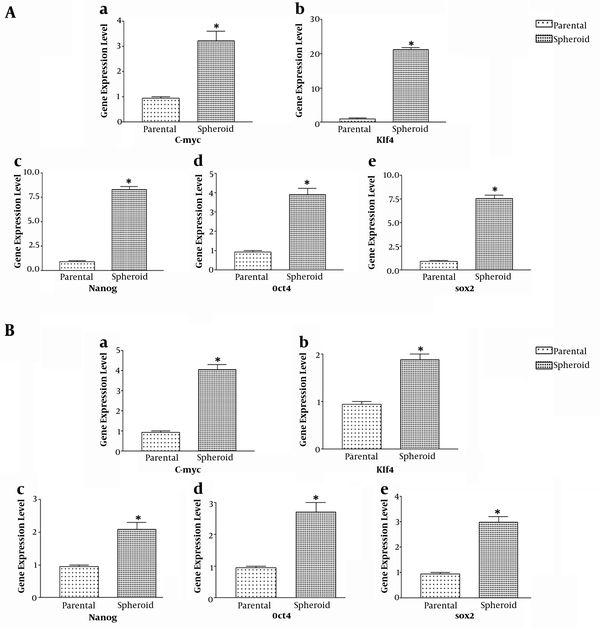
4.3. Spheroid Cells Exhibit Tumorigenic Potential in Vivo
In line with previous studies, subcutaneous injection of low numbers of spheres confirmed the spheroid cells retain the capacity to initiate tumor growth in nude mice (13, 14) (Figure 3A). Our data showed that as few as 1000 spheroid cells from primary tumor were sufficient to obtain tumor growth; however, 1 ×106 parent cells were capable to form tumor in nude mice. Additionally, it was shown that at least 2,500 cells from spheroid cell derived HT-29 cell line were capable to form tumor (Table 2). The data, therefore, indicate that spheroid cells formed by the colon cancer cell are enriched in CSCs. Histopathological examination of xenografts that implicated from spheroid and parental cells showed that these tumors closely resemble the original human tumor (Figure 3B and 3C).
Tumorigenicity of Spheroid Cells in Vivo
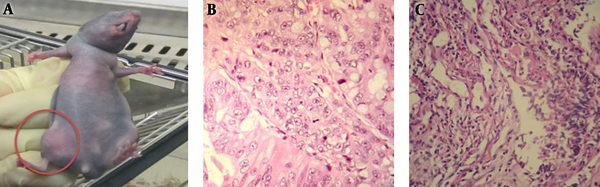
Tumorigenicity of Colonospheres and Parental Cells From Primary and HT-29 Cell Lines in Nude Micea
Cell Type | Patients | Cell Number Injected | Tumor Incidence | Engraftment-Derived Spheres |
---|---|---|---|---|
Spheroid cells (HT-29) | - | 1 × 103 | 0/3 | - |
2.5 × 103 | 3/3 | Yes | ||
5 × 103 | 3/3 | Yes | ||
Parental cells (HT-29) | - | 5 × 105 | 0/3 | - |
1 × 106 | 3/3 | Yes | ||
Spheroid cells (primary tumor) | P1, P2, P3 | 1 × 103 | 1/3* | Yes |
2.5 × 103 | 3/3 | Yes | ||
5 × 103 | 3/3 | Yes | ||
Parental cells (primary tumor) | P1, P2, P3 | 5 × 105 | 0/3 | - |
1 × 106 | 3/3 | Yes |
4.4. Differential miRNA Expression Profile in Colon CSCs and Non-Stem Cells
We used a PCR array to evaluate the differential miRNA expression profile in colon CSCs and non-stem cells. A total of 739 human miRNAs were examined. There are 39 differentially expressed miRNAs, 24 of them had lower mean expression in the cancer stem cells samples. By contrast, 15 miRNAs had higher expression levels in CSCs samples (Figure 4). Of these, miR-495, miR-125, miR-199a were the most significantly up-regulated miRNA. Interestingly, miRNA expression profile in primary cancer stem cells compared to HT-29 derived cancer stem cells was different. Expression of some miRNAs including miR-125, miR-124, miR- 495, miR- 497, miR-663, miR-214, miR-10b, and miR-199a significantly increased in comparison to primary tumor derived CSCs. By contrast, down-regulation of miR-128, miR-126, miR-205, and miR-200b without noticeable changes in primary tumor derived CSCs were observed.
Differential miRNA Expression Profile in Colon Cancer Stem and Non-Stem Cells in Primary Tumors (Left) and HT-29 (Right)
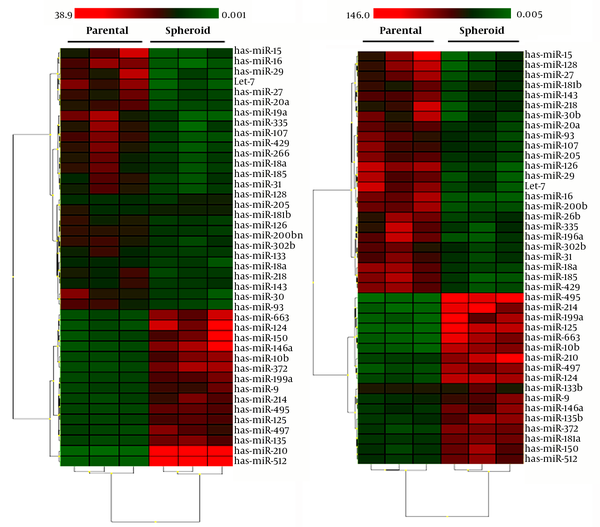
5. Discussion
In contrast to traditional models of cancer biology, the cancer stem cell model proposes that only a few fractions of cells within a tumor microenvironment have the potential to initiate and sustain tumor growth known as cancer stem cells (CSCs) (15). Genetics and epigenetic control as well as signaling from neighboring cells regulate stem cells properties. MiRNA levels control self-renewal, pluripotency and differentiation properties of stem cells. Enhancement of epigenetic abnormalities due to failure in repair errors leads to some signaling cascades that support tumorigenesis and creation of cancer stem cells (16). Isolated cancer stem cells from primary and HT-29 cancer cells express important factors in stemness-keeping and decisive properties of progenitor cells including Sox-2, Oct-4 and Nanog that were previously reported to be involved in stemness maintenance in human CSCs (17).
Various stages of colon cancer have showed different miRNA expression profiles. These data show that miRNAs regulate special phenotype and biological properties of CSCs (18). So, miRNAs profiling in colon CSCs in comprasion to non-stem cells help to clarify miRNAs roles in progression of cancer.
We isolated CSCs according to previous studies, enriched CSCs with spheres creation and documented using CD44+ and EPCAM+. Here, we profiled primary and HT-29 cancer stem cells compared to parent colorectal cancer samples and found 39 differentially expressed miRNAs, twenty four of which had lower mean expression in the cancer stem cells samples. By contrast, 15 miRNAs had higher expression levels in CSCs samples suggesting their function to block tumor suppressor genes or to activate oncogenes in colorectal cancer. One of the over expressed miRNAs in CSCs was 181a that correlates with induction of TGF-β epithelial to mesenchymal transition (EMT) through smad-7 transcription factor (19). Similarly, miR-302 was down-regulated in CSCs that block TGF-β mediated EMT (20). Another miRNA which promotes EMT is miR-125b that was up-regulated in CSCs showing that TGF-β EMT pathway is commonly activated in CSCs and plays important roles in CSCs properties. MiR-125b also inhibits apoptosis by affecting p53 and its target genes p21 and Puma (21). Another up-regulated miRNA that is involved in apoptosis inhibition is miR-372 through down-regulation of a tumor suppressor gene, LATS-2 (22).
CSCs indicate some special characteristics such as continuous cell proliferation and ability to colony formation which are regulated by miRNAs. In the current study miR-495, miR-214 and miR199a up-regulated in CSCs and were involved in cell proliferation that was previously reported in breast and ovarian cancers (23-25). MiR-495 promotes cell proliferation and oncogenesis via down-regulation of E-cadherin and REDD-1 (23) but miR-214 affects the p53-Nanog axis (24). Furthermore, enhanced proliferation and maintenance of HT-29 derived CSCs compared to primary tumor derived CSCs could be related to miRNAs including miR-495, miR-214 and miR-199a.
Cancer stem cells involve in invasion and metastasis through different genes regulated by miRNAs. MiR-9, miR-10b and miR-663 have critical roles in invasion and migration. MiR-9 has a dual function and plays a critical role in local recurrence of cancer (26). By contrast, some studies showed that it decreases cell viability and increases apoptotic activity through blocking anti-apoptotic gene, MTHFD-2 (27). MiR-10b targets KLF4 leading to increased migration and invasion as well as enhancing pro-metastatic gene products including RhoC, urokinase plasminogen activator receptor (uPAR), α3-integrin, and MT1-MMP (28). One of the other important up-regulated miRNAs in CSCs derived from both primary and HT-29 cancer cells was miR-146a. Previous studies indicated that miR-146a may play important roles in cell survival and multi drug resistance (29).
In this study, miR-429, miR-196, miR-218, miR-18a, and miR-26b as well as miR-15 and miR-16 were decreased in CSCs. MiR-429 modulate migration through ets-1 (30). On the other hand, miR-218 targets oncogene, Bmi-1 (31). Therefore, down-regulation of miR-429 and miR-218 increase stem cells proliferation and migration. Also, a previous study showed mir-18a acts as tumor suppressor by targeting K-ras which plays a critical role in cancer cells’ growth and cell proliferation (32). One of the most important down-regulated miRNAs is miR-26b that causes the significant suppression of the cell growth and the induction of apoptosis in cancer cells (33). Other important miRNAs including miR-15, miR-16, miR-181b and miR-196a induce apoptosis by targeting bcl-2 and their down-regulation led to maintenance of cancer stem cells (34-36).
In summary, we showed that different miRNA expression in both primary and HT-29 colon CSCs indicate stemness maintenance of colon CSCs may be largely regulated by miRNAs. This study adds further evidence to prove miRNAs’ roles in colon cancer invasion and recurrence and the findings of the current study may contribute to the treatment of colon cancer.
Acknowledgements
References
-
1.
Siegel R, Naishadham D, Jemal A. Cancer statistics, 2012. CA Cancer J Clin. 2012;62(1):10-29. [PubMed ID: 22237781]. https://doi.org/10.3322/caac.20138.
-
2.
Yee YK, Tan VP, Chan P, Hung IF, Pang R, Wong BC. Epidemiology of colorectal cancer in Asia. J Gastroenterol Hepatol. 2009;24(12):1810-6. [PubMed ID: 20002940]. https://doi.org/10.1111/j.1440-1746.2009.06138.x.
-
3.
Mousavi SM, Gouya MM, Ramazani R, Davanlou M, Hajsadeghi N, Seddighi Z. Cancer incidence and mortality in Iran. Ann Oncol. 2009;20(3):556-63. [PubMed ID: 19073863]. https://doi.org/10.1093/annonc/mdn642.
-
4.
Abdul Khalek FJ, Gallicano GI, Mishra L. Colon cancer stem cells. Gastrointest Cancer Res. 2010;(Suppl 1):S16-23. [PubMed ID: 21472043].
-
5.
Bartel DP. MicroRNAs: genomics, biogenesis, mechanism, and function. Cell. 2004;116(2):281-97. [PubMed ID: 14744438].
-
6.
Liu C, Tang DG. MicroRNA regulation of cancer stem cells. Cancer Res. 2011;71(18):5950-4. [PubMed ID: 21917736]. https://doi.org/10.1158/0008-5472.CAN-11-1035.
-
7.
Otsubo T, Akiyama Y, Hashimoto Y, Shimada S, Goto K, Yuasa Y. MicroRNA-126 inhibits SOX2 expression and contributes to gastric carcinogenesis. PLoS One. 2011;6(1). e16617. [PubMed ID: 21304604]. https://doi.org/10.1371/journal.pone.0016617.
-
8.
Ji J, Yamashita T, Budhu A, Forgues M, Jia HL, Li C, et al. Identification of microRNA-181 by genome-wide screening as a critical player in EpCAM-positive hepatic cancer stem cells. Hepatology. 2009;50(2):472-80. [PubMed ID: 19585654]. https://doi.org/10.1002/hep.22989.
-
9.
Voorhoeve PM, le Sage C, Schrier M, Gillis AJ, Stoop H, Nagel R, et al. A genetic screen implicates miRNA-372 and miRNA-373 as oncogenes in testicular germ cell tumors. Cell. 2006;124(6):1169-81. [PubMed ID: 16564011]. https://doi.org/10.1016/j.cell.2006.02.037.
-
10.
O'Donnell KA, Wentzel EA, Zeller KI, Dang CV, Mendell JT. c-Myc-regulated microRNAs modulate E2F1 expression. Nature. 2005;435(7043):839-43. [PubMed ID: 15944709]. https://doi.org/10.1038/nature03677.
-
11.
Calin GA, Croce CM. MicroRNA signatures in human cancers. Nat Rev Cancer. 2006;6(11):857-66. [PubMed ID: 17060945]. https://doi.org/10.1038/nrc1997.
-
12.
Tran N, McLean T, Zhang X, Zhao CJ, Thomson JM, O'Brien C, et al. MicroRNA expression profiles in head and neck cancer cell lines. Biochem Biophys Res Commun. 2007;358(1):12-7. [PubMed ID: 17475218]. https://doi.org/10.1016/j.bbrc.2007.03.201.
-
13.
Vermeulen L, Todaro M, de Sousa Mello F, Sprick MR, Kemper K, Perez Alea M, et al. Single-cell cloning of colon cancer stem cells reveals a multi-lineage differentiation capacity. Proc Natl Acad Sci U S A. 2008;105(36):13427-32. [PubMed ID: 18765800]. https://doi.org/10.1073/pnas.0805706105.
-
14.
Todaro M, Alea MP, Di Stefano AB, Cammareri P, Vermeulen L, Iovino F, et al. Colon cancer stem cells dictate tumor growth and resist cell death by production of interleukin-4. Cell Stem Cell. 2007;1(4):389-402. [PubMed ID: 18371377]. https://doi.org/10.1016/j.stem.2007.08.001.
-
15.
Reya T, Morrison SJ, Clarke MF, Weissman IL. Stem cells, cancer, and cancer stem cells. Nature. 2001;414(6859):105-11. [PubMed ID: 11689955]. https://doi.org/10.1038/35102167.
-
16.
Garg M. MicroRNAs, stem cells and cancer stem cells. World J Stem Cells. 2012;4(7):62-70. [PubMed ID: 22993663]. https://doi.org/10.4252/wjsc.v4.i7.62.
-
17.
Caruso S, Bazan V, Rolfo C, Insalaco L, Fanale D, Bronte G, et al. MicroRNAs in colorectal cancer stem cells: new regulators of cancer stemness? Oncogenesis. 2012;1. e32. [PubMed ID: 23552465]. https://doi.org/10.1038/oncsis.2012.33.
-
18.
Wang Y, Medvid R, Melton C, Jaenisch R, Blelloch R. DGCR8 is essential for microRNA biogenesis and silencing of embryonic stem cell self-renewal. Nat Genet. 2007;39(3):380-5. [PubMed ID: 17259983]. https://doi.org/10.1038/ng1969.
-
19.
Parikh A, Lee C, Joseph P, Marchini S, Baccarini A, Kolev V, et al. microRNA-181a has a critical role in ovarian cancer progression through the regulation of the epithelial-mesenchymal transition. Nat Commun. 2014;5:2977. [PubMed ID: 24394555]. https://doi.org/10.1038/ncomms3977.
-
20.
Subramanyam D, Lamouille S, Judson RL, Liu JY, Bucay N, Derynck R, et al. Multiple targets of miR-302 and miR-372 promote reprogramming of human fibroblasts to induced pluripotent stem cells. Nat Biotechnol. 2011;29(5):443-8. [PubMed ID: 21490602]. https://doi.org/10.1038/nbt.1862.
-
21.
Amir S, Ma AH, Shi XB, Xue L, Kung HJ, Devere White RW. Oncomir miR-125b suppresses p14(ARF) to modulate p53-dependent and p53-independent apoptosis in prostate cancer. PLoS One. 2013;8(4). e61064. [PubMed ID: 23585871]. https://doi.org/10.1371/journal.pone.0061064.
-
22.
Cho WJ, Shin JM, Kim JS, Lee MR, Hong KS, Lee JH, et al. miR-372 regulates cell cycle and apoptosis of ags human gastric cancer cell line through direct regulation of LATS2. Mol Cells. 2009;28(6):521-7. [PubMed ID: 19937137]. https://doi.org/10.1007/s10059-009-0158-0.
-
23.
Hwang-Verslues WW, Chang PH, Wei PC, Yang CY, Huang CK, Kuo WH, et al. miR-495 is upregulated by E12/E47 in breast cancer stem cells, and promotes oncogenesis and hypoxia resistance via downregulation of E-cadherin and REDD1. Oncogene. 2011;30(21):2463-74. [PubMed ID: 21258409]. https://doi.org/10.1038/onc.2010.618.
-
24.
Xu CX, Xu M, Tan L, Yang H, Permuth-Wey J, Kruk PA, et al. MicroRNA miR-214 regulates ovarian cancer cell stemness by targeting p53/Nanog. J Biol Chem. 2012;287(42):34970-8. [PubMed ID: 22927443]. https://doi.org/10.1074/jbc.M112.374611.
-
25.
Song G, Zeng H, Li J, Xiao L, He Y, Tang Y, et al. miR-199a regulates the tumor suppressor mitogen-activated protein kinase kinase kinase 11 in gastric cancer. Biol Pharm Bull. 2010;33(11):1822-7. [PubMed ID: 21048306].
-
26.
Zhou X, Marian C, Makambi KH, Kosti O, Kallakury BV, Loffredo CA, et al. MicroRNA-9 as potential biomarker for breast cancer local recurrence and tumor estrogen receptor status. PLoS One. 2012;7(6). e39011. [PubMed ID: 22723919]. https://doi.org/10.1371/journal.pone.0039011.
-
27.
Selcuklu SD, Donoghue MT, Rehmet K, de Souza Gomes M, Fort A, Kovvuru P, et al. MicroRNA-9 inhibition of cell proliferation and identification of novel miR-9 targets by transcriptome profiling in breast cancer cells. J Biol Chem. 2012;287(35):29516-28. [PubMed ID: 22761433]. https://doi.org/10.1074/jbc.M111.335943.
-
28.
Tian Y, Luo A, Cai Y, Su Q, Ding F, Chen H, et al. MicroRNA-10b promotes migration and invasion through KLF4 in human esophageal cancer cell lines. J Biol Chem. 2010;285(11):7986-94. [PubMed ID: 20075075]. https://doi.org/10.1074/jbc.M109.062877.
-
29.
Khorrami S, Zavaran Hosseini A, Mowla SJ, Soleimani M, Rakhshani N, Malekzadeh R. MicroRNA-146a induces immune suppression and drug-resistant colorectal cancer cells. Tumour Bioll. 2017;39(5). https://doi.org/10.1177/1010428317698365.
-
30.
Sun T, Wang C, Xing J, Wu D. miR-429 modulates the expression of c-myc in human gastric carcinoma cells. Eur J Cancer. 2011;47(17):2552-9. [PubMed ID: 21684154]. https://doi.org/10.1016/j.ejca.2011.05.021.
-
31.
He G, Hu J, Li T, Ma X, Meng J, Jia M, et al. Arrhythmogenic effect of sympathetic histamine in mouse hearts subjected to acute ischemia. Mol Med. 2012;18:1-9. [PubMed ID: 21989948]. https://doi.org/10.2119/molmed.2011.00225.
-
32.
Tsang WP, Kwok TT. The miR-18a* microRNA functions as a potential tumor suppressor by targeting on K-Ras. Carcinogenesis. 2009;30(6):953-9. [PubMed ID: 19372139]. https://doi.org/10.1093/carcin/bgp094.
-
33.
Ma YL, Zhang P, Wang F, Moyer MP, Yang JJ, Liu ZH, et al. Human embryonic stem cells and metastatic colorectal cancer cells shared the common endogenous human microRNA-26b. J Cell Mol Med. 2011;15(9):1941-54. [PubMed ID: 20831567]. https://doi.org/10.1111/j.1582-4934.2010.01170.x.
-
34.
Cimmino A, Calin GA, Fabbri M, Iorio MV, Ferracin M, Shimizu M, et al. miR-15 and miR-16 induce apoptosis by targeting BCL2. Proc Natl Acad Sci U S A. 2005;102(39):13944-9. [PubMed ID: 16166262]. https://doi.org/10.1073/pnas.0506654102.
-
35.
Liu S, Wicha MS. Targeting breast cancer stem cells. J Clin Oncol. 2010;28(25):4006-12. [PubMed ID: 20498387]. https://doi.org/10.1200/JCO.2009.27.5388.
-
36.
Braig S, Mueller DW, Rothhammer T, Bosserhoff AK. MicroRNA miR-196a is a central regulator of HOX-B7 and BMP4 expression in malignant melanoma. Cell Mol Life Sci. 2010;67(20):3535-48. [PubMed ID: 20480203]. https://doi.org/10.1007/s00018-010-0394-7.