Abstract
Keywords
1. Context
The first patient infected with the novel coronavirus emerged on December 30, 2019, in a sea-food restaurant in Wuhan, Hubei province, P.R. China. Then, the infection spread to most countries of the world, up to 200 countries (1-3). The World Health Organization (WHO) named the pathogen, initially called 2019-nCoV, as SARS-CoV-2 on February 12. The virus causes Coronavirus Disease 2019 (COVID-2019). The WHO report declared the virus as responsible for a pandemic on March 11, 2020 (4, 5). The initial whole-genome sequence of 2019-nCoV (COVID-19) was available at GenBank (Wuhan-Hu-1, GenBank accession number MN908947) of the NCBI on January 10, 2020 (2, 6). In the phylogenetic analysis, COVID-19 belongs to the realm of Riboviria, the phylum of incertae sedis, the order of Nidovirales s. the family of Coronaviridae, the subfamily of Orthocoronavirinae, and the genera of betacoronavirus (7-10). It shares ancestry with bat coronavirus HKU9-1, and is similar to Severe Acute Respiratory Syndrome (SARS) coronaviruses (8). In terms of infectivity, the genomic analysis reveals how the spike protein of SARS-CoV-2 reacts with the human angiotensin-converting enzyme 2 (ACE2) receptor (11-14). ACE2 is found in cardiomyocytes, cardiac fibroblasts, and coronary endothelial cells. Importantly, ACE2 serves as a regulator for heart function (Figure 1) (11, 12, 15, 16).
The major potential drugs mechanisms against SARS-CoV-2, This graphical abstract demonstrates the life cycle of SARS-CoV-2 in the host cells. The target of potential drugs exhibited by red banned icon in virus life cycle
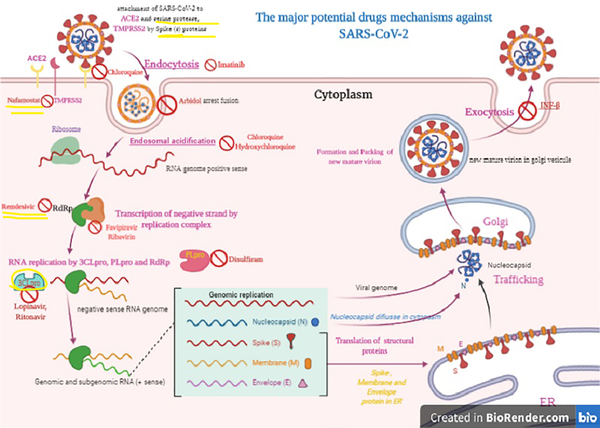
The Corona Viruses (CoVs) contain non-segmented, single‐stranded, positive‐sense RNA (+ssRNA; ~30 kb) genomes (9). SARS-CoV-2 has the largest RNA genome within the family, containing approximately 30 kilobases (kb) genome (9, 17). The genomic pattern and organization of SARS-CoV-2 are similar to those of other beta-coronaviruses (7, 8). Despite being classified as a beta-coronavirus, SARS-CoV-2 differs from SARS-CoV and MERS-CoV (7). SARS-CoV-2 gene nucleotide similarity to MERS-CoV and SARS-CoV is less than 80% and 89.10%, respectively (2, 7, 10). A minimum of six Open Reading Frames (ORFs) are present in the genome (7). Several proteins are formed from these ORF products via cleavage through the proteolysis process. PLpro and 3CLpro carry out these proteolysis reactions (18). 3CLpro plays a principal role in the replication of virus particles (7, 18). Therefore, 3CLpro presents the best potential target for anti-coronavirus inhibitors and should be investigated further for drug design (18). Other ORFs adjacent to the 3’ end can encode no less than four major structural proteins: spike (S), membrane (M), envelope (E), and nucleocapsid (N) proteins (7).
Scientists are endeavoring to find drugs to treat COVID-19. These attempts are currently focused on at least 30 drugs such as natural agents, Western drugs, and traditional Chinese therapeutic agents, each of which is potentially efficient against COVID-19. Clinical studies have been rapidly conducted on some of these agents, and the results are indicative of their initial efficacy against COVID-19 (19-22). The current study aimed to review the possible and proposed drugs for the treatment of COVID-19. A summary of information about these drugs is provided in Table 1. It is clear that extensive clinical research is needed to conclusively confirm the effect of these drugs on COVID-19. The drugs studied in the present survey may also have side effects; thus, in prescribing these drugs, it is necessary to evaluate the cost-benefit ratio. Some side effects of these drugs aggravate the symptoms of coronavirus. In these cases, more care is needed in prescribing the drug. Some other side effects of the drug may be negligible in the face of virus-related mortality.
Main Available Information About Possible Drugs in the Treatment of Coronavirus
Drug Name | Usage | Dose | Daily Usage | Treatment Period | Description | Side Effects |
---|---|---|---|---|---|---|
Interferon-α | Nebulization | 200,000–400,000 IU/kg | Twice a day | 5-7 days | Frequently employed for hepatitis treatment | Flu-like syndrome; Fever; Chills; Generalized aches; Pain; Headache; Poor appetite; Fatigue; Drowsiness; Low blood counts |
Spray | 8000 IU/injection; 1/1-2 hours | 8-10 spray/day | 5-7 days | Use for high-risk patients with close contact with patients who were suspected to be infected with COVID-19 or during the primary stages of manifestations in the upper respiratory tract | ||
Lopinavir/litonavir | Oral | 200 mg /capsule; two capsules each time | 2 times/day (4 capsules/day) | 5-7 days | Use for adult patients infected with COVID-19 pneumonia/(HIV) infection in children over 14 days of age | Diarrhea; Headache; Weakness; Nausea; Stomach upset; Drowsiness; Dizziness; Nausea; Vomiting; Stomach pain; Diarrhea; Weakness; Rash |
Ribavirin | Oral | 500 mg each time | 2-3 times per day | Acting by the mechanism of nucleoside analog | Nausea; Flu-like symptoms; Weakness; Low white blood cell counts; Fever; Chills or shaking; Headache; Feeling anxious or irritable Muscle pain; Vomiting; Loss of appetite | |
Chloroquine, Chloroquine phosphate, and Hydroxychloroquine | Oral | Chloroquine: 300 mg; Hydroxychloroquine: 400 mg or 200 mg | Chloroquine: twice a day; Hydroxychloroquine: 400 mg two times on the first day, 200 mg two times a day for a course of four days | 1-4 days | Broad-spectrum antiviral effects, but most commonly used as an antimalarial drug; Obstructing COVID-19 infection by inhibiting viral enzymes such as DNA and RNA polymerase or viral processes including assembly, protein glycosylation, and transport of newly synthesized virus particles | Headache; Nausea; Loss of appetite; Diarrhea; Stomach pain; Rash; Itching |
Arbidol | Oral | 200 mg | 3 times a day | 10 days | Generally used in Russia and China; inhibiting membrane fusion; In vitro anti-COVID-19 effects at a concentration of 10-30 µM | None |
Favipiravir | Oral | 500 mg on the initial day; Two doses of 600 mg for 13 days in combination with aerosol inhalation of interferon-alpha (five million units two times a day) | 14 | Specifically inhibiting the activity of RNA-dependent RNA polymerase (RdRP) in influenza virus and demonstrable effects against various viruses like yellow fever virus, Zika virus, foot-and-mouth disease virus, and West Nile virus | Reduced body weight; Vomiting; Reduced locomotor activity | |
Remdesivir | Oral | 200 mg on the first day; 100 mg once a day as the maintenance dose | 10-day | Classified as nucleotide analogs (1′-cyano-substituted adenosine nucleotide analog); Against various RNA viruses; Reducing viral RNA production by interfering with the activity of viral RNA-dependent RNA polymerase and evading proofreading by viral exoribonuclease (ExoN); Causing premature termination | Increased liver enzymes; Nausea; Vomiting | |
Darunavir | Oral | 300 μM | Antiretroviral protease inhibitor; Treatment and prevention of human immunodeficiency virus (HIV) infection/acquired immunodeficiency syndrome (AIDS) | Nausea; Vomiting; Diarrhea; Stomach pain Headache; Rash | ||
Azithromycin | Oral | 500 mg on the first day; 250 mg daily for five days | 6 days | Combination of hydroxychloroquine (200 mg X 3 daily for a course of 10 days) in addition to Azithromycin as a broad-spectrum antibiotic | Diarrhea or loose stool; Nausea; Abdominal pain; Stomach upset; Vomiting; Constipation; Dizziness; Tiredness | |
Teicoplanin | 8.78 µM dose of 400 mg per day | Treatment of Staphylococcal infections; Inhibitory concentration (IC50) of 1.66 µM | Rashes; Fever; Pruritus; Diarrhea; Nausea; Vomiting | |||
Corticosteroid | Treating viral pneumonia | Ibuprofen increases the expression of ACE2, possibly worsening the symptoms of patients with COVID-19; Glaucoma; Fluid retention Swelling in lower legs; High blood pressure; Psychological effects | ||||
Convalescent plasma | Used for Spanish influenza epidemic; Argentine hemorrhagic fever; severe acute respiratory syndrome, and; Ebola; | Mild allergic reactions; Problems with the heart or lungs; Infection |
2. Method
All articles in PubMed containing keywords COVID-19, virus, and drug were collected using the search builder software. Then, filtering was performed based on the presence of keywords in the title and abstract of the articles. Finally, from the articles in the created library, the related information was extracted and compared, and conclusions were made.
2.1. Antiviral Treatment
The antiviral treatment options under examination include Interferon α (IFN-α), Lopinavir/ritonavir, Chloroquine, Chloroquine phosphate, Hydroxychloroquine, Ribavirin, Arbidol, Favipiravir, Remdesivir, Darunavir, Imatinib, etc. Some of these medicines have been suggested for use in the most recent version of the Guidelines for the Treatment of COVID-19 by the WHO (23, 24). Each of these candidate products is considered in detail as follows.
2.2. Interferon-α
Interferon-α (IFN-α) is a broad-spectrum antiviral immune protein (19). It is frequently applied for hepatitis treatment; however, it can reportedly suppress the in vitro reproduction of SARS-CoV (25). Viral load can be diminished by interferon-α at the early stages of infection, which subsequently proceeds to relieve clinical manifestations and truncate the period of infection (19, 26). The major administration method is vapor inhalation, and the recommended usage is as follows:
1. Nebulization: 200,000-400,000 IU/kg or 2-4 μg/kg interferon-α in 2 mL sterile water is used; nebulization is carried out twice a day for a course of 5-7 days (27, 28);
2. Spray: This method is used for high-risk patients with close contact with patients who are suspected of being infected with COVID-19 or during the primary stages of manifestations in the upper respiratory tract. The guideline is to administer 1-2 sprays for each of the two nasal cavities and 8–10 sprays on the oropharynx. A dose of 8000 IU should be used in each injection, and each injection is carried out once every 1-2 hours; a total of 8-10 sprays per day are applied for 5-7 days (27, 28).
2.3. Lopinavir/Ritonavir
Lopinavir/Ritonavir has been used in adult patients infected with COVID-19 pneumonia (29). The efficacy and side effects have not been determined. Lopinavir/Ritonavir is a drug used to treat human immunodeficiency virus (HIV) infection in children over 14 days of age when used in combination with other protocols (30-32). The efficiency dosage of Lopinavir/Ritonavir is 200 mg/50 mg/capsule, two capsules each time, two times/day with oral administration (21, 27, 29, 33, 34) (Table 2).
Major Potential Drug Properties Against COVID-19
Classification | Reasoning for Utilize | Mechanism of Action | Proof/Try | Probable Side Effects | |
---|---|---|---|---|---|
Chloroquine | Antimalarial | In vitro activity with immunomodulating actions | stopping viral enzymes and inhibiting processes of viral DNA and RNA polymerase, viral protein glycosylation, virus assembly, new virus particle transport, and virus release, Inhibition of cell receptor ACE2 | Pre-clinical analysis for the process of definitive decision against COVID-19. There have been some limited uses in clinical assessments | Risk of cardiac arrhythmias and retinal damage; It can be dangerous in G6PD deficiency and diabetic conditions |
Hydroxychloroquine | Antimalarial agent | In vitro activity with immunomodulating actions | Stopping of viral enzymes and inhibiting the processes of viral DNA and RNA polymerase, viral protein glycosylation, virus assembly, new virus particle transport, and virus release. Inhibition of cell receptor ACE2 | Pre-clinical analysis for the process of definitive decision against COVID-19. There have been some limited uses in clinical assessments. It can be more effective from chloroquine. | Risk of cardiac arrhythmias and retinal damage. It can be dangerous in G6PD deficiency and diabetic conditions. |
Lopinavir; Ritonavir | HIV protease inhibitor | In vitro and animal models | Binding to the main protease of the virus, Mpro; it can be suppress coronavirus activity. | Pre-clinical and a retrospective cohort study has been done. | Risk of cardiac arrhythmias |
Remdesivir | Nucleoside analog | In vitro activity | It acts as an inhibitor of RNA-dependent RNA polymerases (RdRps) | Several clinical trials are currently being conducted | Gastrointestinal side effects |
2.4. Ribavirin
Ribavirin is a broad-spectrum antiviral drug act by the mechanism of nucleoside analog (30, 35). Ribavirin is administrated via intravenous infusion (36, 37). For adults, the efficiency dose of Ribavirin is 500 mg, 2-3 times per day when used along with IFN-α or Lopinavir/ritonavir (37, 38).
2.5. Chloroquine, Chloroquine Phosphate, and Hydroxychloroquine
Chloroquine is a broad-spectrum antiviral drug, but it is most commonly used as an antimalarial drug (39). Chloroquine was discovered to obstruct COVID-19 infection by inhibiting viral enzymes such as DNA and RNA polymerase or viral processes, including assembly, protein glycosylation, transport of newly synthesized virus particles, and virus release at a low-micromolar concentration (24, 40). The half-maximal effective concentration (EC50) and half-cytotoxic concentration (CC50) of Chloroquine were found to be 1.13 μM and greater than 100 mM, respectively (24, 41). The phosphate salt portion of chloroquine is called chloroquine phosphate. Indeed chloroquine, which as a quinolone interferes with the biosynthesis of nucleic acids, is, in fact, used for the treatment of malarial infections or inflammation (42, 43). It is administered orally with an efficacy dose of 500 mg (300 mg for chloroquine), twice a day for adults (42, 43). Hydroxychloroquine has in vitro activity against COVID-19 by inhibiting viral enzymes such as DNA and RNA polymerase or viral processes, including assembly, protein glycosylation, and transport of newly synthesized virus particles (21, 41, 43-45). Hydroxychloroquine is administered as oral tablets. Its efficacy dose is 400 mg two times on the first day, followed by 200 mg two times a day for four days (21, 41, 43-45) (Table 2).
2.6. Arbidol
Arbidol is a Russian-made antiviral drug for influenza infection, generally used in Russia and China (46). Arbidol inhibits membrane fusion (46). Arbidol impedes the interaction between the virus and its target host cells through inhibiting the viral capsid-target cell membrane fusion, thereby hindering the entry of the virus into the target cell (46). Arbidol has an in vitro anti-COVID-19 effect at a concentration of 10-30 µM (47).
It is administered orally, and its efficacy dose for adults is 200 mg three times a day. The maximum period of treatment is 10 days (47).
2.7. Favipiravir
Favipiravir specifically inhibits the activity of RNA-dependent RNA polymerase (RdRP) in the influenza virus and has a demonstrable effect against various viruses like yellow fever virus, Zika virus, foot-and-mouth disease virus, and West Nile virus; it is, therefore, a candidate for producing broad-spectrum effects against SARS-CoV-2 (48, 49). Favipiravir is currently undergoing clinical trials and in vitro analysis in treating COVID-19 (21, 47). Favipiravir is considered a prodrug that becomes active following metabolization and conversion into the phosphoribosylated form (favipiravir-RTP) in cells. This drug inhibits the activity of viral RNA polymerase when recognized by this enzyme as substrate (21, 47, 49). It is administered orally, and its efficacy dose is 500 mg on the initial day of treatment followed by two doses of 600 mg for 13 days, in combination with aerosol inhalation of interferon-alpha (five million units two times a day) (21, 47).
2.8. Remdesivir
A novel drug against viruses, remdesivir, is classified as a nucleotide analog (1’-cyano-substituted adenosine nucleotide analog) with a broad-spectrum antiviral effect, especially against various RNA viruses (50). As a prodrug, remdesivir is converted into its active form GS-441524 (51). GS-441524, as an analog of the adenosine nucleotide, can reduce viral RNA production by interfering with the activity of viral RNA-dependent RNA polymerase and evading proofreading by viral exoribonuclease (ExoN) (50, 51). Premature termination is observed following the incorporation of remdesivir into nascent viral RNA chains. This drug was first developed by Gilead Sciences and is used for the treatment of Ebola, Marburg, and COVID-19 infections (21, 27, 40). Remdesivir can potentially treat COVID-19. Remdesivir can effectively block COVID-19 infection at low micromolar concentrations > 100 μ (21, 27, 52).
It has been shown that this drug can inhibit SARS-CoV [half-maximal inhibitory concentration (IC50) = 0.069 μM] and MERS-CoV (IC50 = 0.074 μM) replication (27, 52, 53). In Vero E6 cells, an EC50 of 0.77 μM was calculated for remdesivir, while the EC90 was 1.76 μM in the inhibition of COVID-19 (53-55). The administration route is intravenous (IV) the efficacy dose is 200 mg on the first day as the loading dose, and 100 mg once a day as the maintenance dose, and the therapeutic duration is 10 days (Table 2) (27, 53-55).
2.9. Darunavir
Darunavir is an antiretroviral protease inhibitor. Darunavir is an N, N-disubstituted benzenesulfonamide harboring an unsubstituted amino group at the 4-position used in the treatment and prevention of HIV infection and Acquired Immunodeficiency Syndrome (AIDS) (56, 57). In vitro experiments indicated that viral replication is significantly inhibited by 300 μM darunavir (29, 58). Darunavir is being studied as a possible treatment for SARS-CoV-2 due to in vitro evidence supporting its ability to combat the infection (58, 59). Clinical trials are underway and expected to conclude in August 2020 (58, 59).
2.10. Other Potential Drugs
The other candidates for treating COVID-19 include saquinavir, indinavir, shikonin, ebselen, atazanavir, carfilzomib, tipranavir, fosamprenavir, cyclosporin A, presatovir, enzaplatovir, abacavir, carmofur, bortezomib, maribavir, raltegravir, elvitegravir, montelukast, deoxyrhapontin, polydatin, chalcone, disulfiram, tideglusib, PX- 12, TDZD-8, and cinanserin (60-64). Imatinib, as an inhibitor of type II transmembrane serine protease (TMSPSS2) and BCR-ABL kinase, inhibits the fusion of virions with the endosomal membrane (62, 64). Furthermore, Chinese herbs including Rhizoma Polygoni Cuspidati and Radix Sophorae Tonkinensis possibly have active ingredients that may be efficient against COVID-19 (65-67).
2.11. Antibiotic Therapy/Azithromycin/Teicoplanin
Antibiotic resistance may lead to more COVID-19 deaths in relation to bacterial superinfections (68). In response to this risk, antibiotic therapy is used in supportive care (69). In one experimental protocol, a combination of hydroxychloroquine (200 mg X 3 daily for a course of 10 days), in addition to Azithromycin as a broad-spectrum antibiotic (500 mg on the first day, followed by 250 mg daily for five days), was used in COVID-19 infection in France (44, 70).
Teicoplanin is a glycopeptide antibiotic that is ordinarily utilized for the treatment of Staphylococcal infections. It was discovered to be active against SARS-CoV in vitro; therefore, it could be a candidate for being included in the list of therapeutic agents used against COVID-19. The inhibitory concentration (IC50) of this drug was 1.66 µM, being much lower than the concentration in human blood (8.78 µM dose of 400 mg per day) (71, 72).
2.12. Corticosteroid Therapy
Corticosteroid therapy is an unsuitable choice to treat viral pneumonia; however, it may be advantageous if used in the early acute phase of infection (73, 74). Corticosteroid therapy is a risk factor in systemic use in patients suffering from refractory shock or acute respiratory distress syndrome (73, 74). Caution should be practiced during usage (73, 74). The Food and Drug Administration (FDA) is assessing the utilization of nonsteroidal anti-inflammatory drugs (NSAIDs) in patients showing COVID-19 symptoms (75, 76). These drugs potentially have various side effects. For example, ibuprofen has been found to increase the expression of ACE2, which may worsen the symptoms in patients inflicted with COVID-19 (75-77). Corticosteroid therapy examples include acetaminophen used for temperature control and methylprednisolone used as an appropriate drug for patients with rapid disease progression (4, 76, 78, 79).
2.13. COVID-19 Convalescent Plasma
The therapeutic approach of “convalescent-plasma therapy” includes the plasma transfusion of components, including antibodies from the people who have recovered from COVID-19 into the patients who are suffering from an infection or deemed to be at high risk (80, 81). Convalescent plasma has a long history. It was first attempted on a few patients in 1918 during the Spanish influenza epidemic, and it also decreased the mortality rate from 16% to about 1% among patients suffering from the Argentine hemorrhagic fever. Furthermore, the use of plasma therapy reduced the hospitalization period among patients in Hong Kong suffering from the severe acute respiratory syndrome, and this therapy has also been employed for Ebola treatment (82-84). In convalescent-plasma therapy for COVID-19, plasma must only be collected from individuals who have recovered from COVID-19, based on the premise that plasma transfusion protocols may contain antibodies against the virus (80, 81, 85). This method is not intended for the prevention of infection (80). The optimal ratio of > 1:320 of neutralizing antibody titers may be transferred. Currently, there is a clinical report from Shenzhen Third People's Hospital, China, from January 20, 2020, to March 25, 2020, where the best results have been documented, and clinical manifestations were compared with patients before and after convalescent plasma transfusion (80, 86).
3. Conclusion
Given the deteriorating conditions in the world due to COVID-19 infection, as well as the possible delays in the production of specific drugs and vaccines, examining the effects of drugs already used in the treatment of viral diseases can help manage the current situation. Although these drugs can have a variety of side effects, in many cases, these side effects can be negligible for saving the patient's life. The true effect of these drugs on COVID-19 disease can be confirmed after careful, extensive studies.
References
-
1.
Zhu N, Zhang D, Wang W, Li X, Yang B, Song J, et al. A Novel Coronavirus from Patients with Pneumonia in China, 2019. N Engl J Med. 2020;382(8):727-33. [PubMed ID: 31978945]. [PubMed Central ID: PMC7092803]. https://doi.org/10.1056/NEJMoa2001017.
-
2.
Wu F, Zhao S, Yu B, Chen YM, Wang W, Song ZG, et al. A new coronavirus associated with human respiratory disease in China. Nature. 2020;579(7798):265-9. [PubMed ID: 32015508]. [PubMed Central ID: PMC7094943]. https://doi.org/10.1038/s41586-020-2008-3.
-
3.
Tang JW, Tambyah PA, Hui DSC. Emergence of a novel coronavirus causing respiratory illness from Wuhan, China. J Infect. 2020;80(3):350-71. [PubMed ID: 32001309]. [PubMed Central ID: PMC7127306 interests/personal relationships which may be considered as potential competing interests]. https://doi.org/10.1016/j.jinf.2020.01.014.
-
4.
Wong J, Goh QY, Tan Z, Lie SA, Tay YC, Ng SY, et al. Preparing for a COVID-19 pandemic: a review of operating room outbreak response measures in a large tertiary hospital in Singapore. Can J Anaesth. 2020;67(6):732-45. [PubMed ID: 32162212]. [PubMed Central ID: PMC7090449]. https://doi.org/10.1007/s12630-020-01620-9.
-
5.
Rothan HA, Byrareddy SN. The epidemiology and pathogenesis of coronavirus disease (COVID-19) outbreak. J Autoimmun. 2020;109:102433. [PubMed ID: 32113704]. [PubMed Central ID: PMC7127067]. https://doi.org/10.1016/j.jaut.2020.102433.
-
6.
Corman V, Bleicker T, Brünink S, Drosten C, Zambon M. Diagnostic detection of 2019-nCoV by real-time RT-PCR. World Health Organization; 2020.
-
7.
Chen Y, Liu Q, Guo D. Emerging coronaviruses: Genome structure, replication, and pathogenesis. J Med Virol. 2020;92(4):418-23. [PubMed ID: 31967327]. [PubMed Central ID: PMC7167049]. https://doi.org/10.1002/jmv.25681.
-
8.
Lu R, Zhao X, Li J, Niu P, Yang B, Wu H, et al. Genomic characterisation and epidemiology of 2019 novel coronavirus: implications for virus origins and receptor binding. Lancet. 2020;395(10224):565-74. https://doi.org/10.1016/s0140-6736(20)30251-8.
-
9.
Chan JF, Kok KH, Zhu Z, Chu H, To KK, Yuan S, et al. Genomic characterization of the 2019 novel human-pathogenic coronavirus isolated from a patient with atypical pneumonia after visiting Wuhan. Emerg Microbes Infect. 2020;9(1):221-36. [PubMed ID: 31987001]. [PubMed Central ID: PMC7067204]. https://doi.org/10.1080/22221751.2020.1719902.
-
10.
Guo YR, Cao QD, Hong ZS, Tan YY, Chen SD, Jin HJ, et al. The origin, transmission and clinical therapies on coronavirus disease 2019 (COVID-19) outbreak - an update on the status. Mil Med Res. 2020;7(1):11. [PubMed ID: 32169119]. [PubMed Central ID: PMC7068984]. https://doi.org/10.1186/s40779-020-00240-0.
-
11.
Li X, Geng M, Peng Y, Meng L, Lu S. Molecular immune pathogenesis and diagnosis of COVID-19. J Pharm Anal. 2020;10(2):102-8. [PubMed ID: 32282863]. [PubMed Central ID: PMC7104082]. https://doi.org/10.1016/j.jpha.2020.03.001.
-
12.
Hoffmann M, Kleine-Weber H, Krüger N, Müller M, Drosten C, Pöhlmann S. The novel coronavirus 2019 (2019-nCoV) uses the SARS-coronavirus receptor ACE2 and the cellular protease TMPRSS2 for entry into target cells. bioRxiv. 2020. https://doi.org/10.1101/2020.01.31.929042.
-
13.
Walls AC, Park Y, Tortorici M, Wall A, McGuire AT, Veesler D. Structure, Function, and Antigenicity of the SARS-CoV-2 Spike Glycoprotein. Cell. 2020. https://doi.org/10.1101/2020.02.19.956581.
-
14.
Liu C, Yang Y, Gao Y, Shen C, Ju B, Liu C, et al. Viral Architecture of SARS-CoV-2 with Post-Fusion Spike Revealed by Cryo-EM. bioRxiv. 2020. https://doi.org/10.1101/2020.03.02.972927.
-
15.
Li W, Moore MJ, Vasilieva N, Sui J, Wong SK, Berne MA, et al. Angiotensin-converting enzyme 2 is a functional receptor for the SARS coronavirus. Nature. 2003;426(6965):450-4. [PubMed ID: 14647384]. [PubMed Central ID: PMC7095016]. https://doi.org/10.1038/nature02145.
-
16.
Hoffmann M, Kleine-Weber H, Schroeder S, Kruger N, Herrler T, Erichsen S, et al. SARS-CoV-2 Cell Entry Depends on ACE2 and TMPRSS2 and Is Blocked by a Clinically Proven Protease Inhibitor. Cell. 2020;181(2):271-280 e8. [PubMed ID: 32142651]. [PubMed Central ID: PMC7102627]. https://doi.org/10.1016/j.cell.2020.02.052.
-
17.
Chan JF, Yuan S, Kok KH, To KK, Chu H, Yang J, et al. A familial cluster of pneumonia associated with the 2019 novel coronavirus indicating person-to-person transmission: a study of a family cluster. Lancet. 2020;395(10223):514-23. [PubMed ID: 31986261]. [PubMed Central ID: PMC7159286]. https://doi.org/10.1016/S0140-6736(20)30154-9.
-
18.
Tahir Ul Qamar M, Alqahtani SM, Alamri MA, Chen LL. Structural basis of SARS-CoV-2 3CL(pro) and anti-COVID-19 drug discovery from medicinal plants. J Pharm Anal. 2020;10(4):313-9. [PubMed ID: 32296570]. [PubMed Central ID: PMC7156227]. https://doi.org/10.1016/j.jpha.2020.03.009.
-
19.
Zumla A, Chan JF, Azhar EI, Hui DS, Yuen KY. Coronaviruses - drug discovery and therapeutic options. Nat Rev Drug Discov. 2016;15(5):327-47. [PubMed ID: 26868298]. [PubMed Central ID: PMC7097181]. https://doi.org/10.1038/nrd.2015.37.
-
20.
Zhou Y, Hou Y, Shen J, Huang Y, Martin W, Cheng F. Network-based drug repurposing for novel coronavirus 2019-nCoV/SARS-CoV-2. Cell Discov. 2020;6:14. [PubMed ID: 32194980]. [PubMed Central ID: PMC7073332]. https://doi.org/10.1038/s41421-020-0153-3.
-
21.
Belhadi D, Peiffer-Smadja N, Lescure F, Yazdanpanah Y, Mentré F, Laouénan C. A brief review of antiviral drugs evaluated in registered clinical trials for COVID-19. medRxiv. 2020. https://doi.org/10.1101/2020.03.18.20038190.
-
22.
Liu X, Wang X. Potential inhibitors for 2019-nCoV coronavirus M protease from clinically approved medicines. bioRxiv. 2020. https://doi.org/10.1101/2020.01.29.924100.
-
23.
Jin YH, Cai L, Cheng ZS, Cheng H, Deng T, Fan YP, et al. A rapid advice guideline for the diagnosis and treatment of 2019 novel coronavirus (2019-nCoV) infected pneumonia (standard version). Mil Med Res. 2020;7(1):4. [PubMed ID: 32029004]. [PubMed Central ID: PMC7003341]. https://doi.org/10.1186/s40779-020-0233-6.
-
24.
Cortegiani A, Ingoglia G, Ippolito M, Giarratano A, Einav S. A systematic review on the efficacy and safety of chloroquine for the treatment of COVID-19. J Crit Care. 2020;57:279-83. [PubMed ID: 32173110]. [PubMed Central ID: PMC7270792]. https://doi.org/10.1016/j.jcrc.2020.03.005.
-
25.
Loutfy MR, Blatt LM, Siminovitch KA, Ward S, Wolff B, Lho H, et al. Interferon alfacon-1 plus corticosteroids in severe acute respiratory syndrome: a preliminary study. JAMA. 2003;290(24):3222-8. [PubMed ID: 14693875]. https://doi.org/10.1001/jama.290.24.3222.
-
26.
Chen F, Chan KH, Jiang Y, Kao RY, Lu HT, Fan KW, et al. In vitro susceptibility of 10 clinical isolates of SARS coronavirus to selected antiviral compounds. J Clin Virol. 2004;31(1):69-75. [PubMed ID: 15288617]. [PubMed Central ID: PMC7128415]. https://doi.org/10.1016/j.jcv.2004.03.003.
-
27.
Sheahan TP, Sims AC, Leist SR, Schafer A, Won J, Brown AJ, et al. Comparative therapeutic efficacy of remdesivir and combination lopinavir, ritonavir, and interferon beta against MERS-CoV. Nat Commun. 2020;11(1):222. [PubMed ID: 31924756]. [PubMed Central ID: PMC6954302]. https://doi.org/10.1038/s41467-019-13940-6.
-
28.
Shen KL, Yang YH. Diagnosis and treatment of 2019 novel coronavirus infection in children: a pressing issue. World J Pediatr. 2020;16(3):219-21. [PubMed ID: 32026147]. [PubMed Central ID: PMC7091265]. https://doi.org/10.1007/s12519-020-00344-6.
-
29.
Lin S, Shen R, He J, Li X, Guo X. Molecular Modeling Evaluation of the Binding Effect of Ritonavir, Lopinavir and Darunavir to Severe Acute Respiratory Syndrome Coronavirus 2 Proteases. bioRxiv. 2020. https://doi.org/10.1101/2020.01.31.929695.
-
30.
Chu CM, Cheng VC, Hung IF, Wong MM, Chan KH, Chan KS, et al. Role of lopinavir/ritonavir in the treatment of SARS: initial virological and clinical findings. Thorax. 2004;59(3):252-6. [PubMed ID: 14985565]. [PubMed Central ID: PMC1746980]. https://doi.org/10.1136/thorax.2003.012658.
-
31.
Pham K, Li D, Guo S, Penzak S, Dong X. Development and in vivo evaluation of child-friendly lopinavir/ritonavir pediatric granules utilizing novel in situ self-assembly nanoparticles. J Control Release. 2016;226:88-97. [PubMed ID: 26849919]. https://doi.org/10.1016/j.jconrel.2016.02.001.
-
32.
Su B, Wang Y, Zhou R, Jiang T, Zhang H, Li Z, et al. Efficacy and Tolerability of Lopinavir/Ritonavir- and Efavirenz-Based Initial Antiretroviral Therapy in HIV-1-Infected Patients in a Tertiary Care Hospital in Beijing, China. Front Pharmacol. 2019;10:1472. [PubMed ID: 31920659]. [PubMed Central ID: PMC6920196]. https://doi.org/10.3389/fphar.2019.01472.
-
33.
Cao B, Wang Y, Wen D, Liu W, Wang J, Fan G, et al. A Trial of Lopinavir-Ritonavir in Adults Hospitalized with Severe Covid-19. N Engl J Med. 2020;382(19):1787-99. [PubMed ID: 32187464]. [PubMed Central ID: PMC7121492]. https://doi.org/10.1056/NEJMoa2001282.
-
34.
Yao TT, Qian JD, Zhu WY, Wang Y, Wang GQ. A systematic review of lopinavir therapy for SARS coronavirus and MERS coronavirus-A possible reference for coronavirus disease-19 treatment option. J Med Virol. 2020;92(6):556-63. [PubMed ID: 32104907]. [PubMed Central ID: PMC7217143]. https://doi.org/10.1002/jmv.25729.
-
35.
Peiris JS, Chu CM, Cheng VC, Chan KS, Hung IF, Poon LL, et al. Clinical progression and viral load in a community outbreak of coronavirus-associated SARS pneumonia: a prospective study. Lancet. 2003;361(9371):1767-72. [PubMed ID: 12781535]. [PubMed Central ID: PMC7112410]. https://doi.org/10.1016/s0140-6736(03)13412-5.
-
36.
Wu J, Liu J, Zhao X, Liu C, Wang W, Wang D, et al. Clinical Characteristics of Imported Cases of Coronavirus Disease 2019 (COVID-19) in Jiangsu Province: A Multicenter Descriptive Study. Clin Infect Dis. 2020;71(15):706-12. [PubMed ID: 32109279]. [PubMed Central ID: PMC7108195]. https://doi.org/10.1093/cid/ciaa199.
-
37.
Zhang C, Huang S, Zheng F, Dai Y. Controversial treatments: An updated understanding of the coronavirus disease 2019. J Med Virol. 2020. [PubMed ID: 32219882]. [PubMed Central ID: PMC7228369]. https://doi.org/10.1002/jmv.25788.
-
38.
Elfiky AA. Anti-HCV, nucleotide inhibitors, repurposing against COVID-19. Life Sci. 2020;248:117477. [PubMed ID: 32119961]. [PubMed Central ID: PMC7089605]. https://doi.org/10.1016/j.lfs.2020.117477.
-
39.
Fox RI. Mechanism of action of hydroxychloroquine as an antirheumatic drug. Semin Arthritis Rheum. 1993;23(2 Suppl 1):82-91. [PubMed ID: 8278823]. https://doi.org/10.1016/s0049-0172(10)80012-5.
-
40.
Wang M, Cao R, Zhang L, Yang X, Liu J, Xu M, et al. Remdesivir and chloroquine effectively inhibit the recently emerged novel coronavirus (2019-nCoV) in vitro. Cell Res. 2020;30(3):269-71. [PubMed ID: 32020029]. [PubMed Central ID: PMC7054408]. https://doi.org/10.1038/s41422-020-0282-0.
-
41.
Colson P, Rolain JM, Lagier JC, Brouqui P, Raoult D. Chloroquine and hydroxychloroquine as available weapons to fight COVID-19. Int J Antimicrob Agents. 2020;55(4):105932. [PubMed ID: 32145363]. [PubMed Central ID: PMC7135139]. https://doi.org/10.1016/j.ijantimicag.2020.105932.
-
42.
Gao J, Tian Z, Yang X. Breakthrough: Chloroquine phosphate has shown apparent efficacy in treatment of COVID-19 associated pneumonia in clinical studies. Biosci Trends. 2020;14(1):72-3. [PubMed ID: 32074550]. https://doi.org/10.5582/bst.2020.01047.
-
43.
Yao X, Ye F, Zhang M, Cui C, Huang B, Niu P, et al. In Vitro Antiviral Activity and Projection of Optimized Dosing Design of Hydroxychloroquine for the Treatment of Severe Acute Respiratory Syndrome Coronavirus 2 (SARS-CoV-2). Clin Infect Dis. 2020;71(15):732-9. [PubMed ID: 32150618]. [PubMed Central ID: PMC7108130]. https://doi.org/10.1093/cid/ciaa237.
-
44.
Gautret P, Lagier JC, Parola P, Hoang VT, Meddeb L, Mailhe M, et al. Hydroxychloroquine and azithromycin as a treatment of COVID-19: results of an open-label non-randomized clinical trial. Int J Antimicrob Agents. 2020;56(1):105949. [PubMed ID: 32205204]. [PubMed Central ID: PMC7102549]. https://doi.org/10.1016/j.ijantimicag.2020.105949.
-
45.
Singh AK, Singh A, Shaikh A, Singh R, Misra A. Chloroquine and hydroxychloroquine in the treatment of COVID-19 with or without diabetes: A systematic search and a narrative review with a special reference to India and other developing countries. Diabetes Metab Syndr. 2020;14(3):241-6. [PubMed ID: 32247211]. [PubMed Central ID: PMC7102587]. https://doi.org/10.1016/j.dsx.2020.03.011.
-
46.
Blaising J, Polyak SJ, Pecheur EI. Arbidol as a broad-spectrum antiviral: an update. Antiviral Res. 2014;107:84-94. [PubMed ID: 24769245]. [PubMed Central ID: PMC7113885]. https://doi.org/10.1016/j.antiviral.2014.04.006.
-
47.
Chen C, Zhang Y, Huang J, Yin P, Cheng Z, Wu J, et al. Favipiravir versus Arbidol for COVID-19: A Randomized Clinical Trial. medRxiv. 2020. https://doi.org/10.1101/2020.03.17.20037432.
-
48.
Furuta Y, Komeno T, Nakamura T. Favipiravir (T-705), a broad spectrum inhibitor of viral RNA polymerase. Proc Jpn Acad Ser B Phys Biol Sci. 2017;93(7):449-63. [PubMed ID: 28769016]. [PubMed Central ID: PMC5713175]. https://doi.org/10.2183/pjab.93.027.
-
49.
Delang L, Abdelnabi R, Neyts J. Favipiravir as a potential countermeasure against neglected and emerging RNA viruses. Antiviral Res. 2018;153:85-94. [PubMed ID: 29524445]. https://doi.org/10.1016/j.antiviral.2018.03.003.
-
50.
Agostini ML, Andres EL, Sims AC, Graham RL, Sheahan TP, Lu X, et al. Coronavirus Susceptibility to the Antiviral Remdesivir (GS-5734) Is Mediated by the Viral Polymerase and the Proofreading Exoribonuclease. mBio. 2018;9(2). [PubMed ID: 29511076]. [PubMed Central ID: PMC5844999]. https://doi.org/10.1128/mBio.00221-18.
-
51.
Brown AJ, Won JJ, Graham RL, Dinnon K3, Sims AC, Feng JY, et al. Broad spectrum antiviral remdesivir inhibits human endemic and zoonotic deltacoronaviruses with a highly divergent RNA dependent RNA polymerase. Antiviral Res. 2019;169:104541. [PubMed ID: 31233808]. [PubMed Central ID: PMC6699884]. https://doi.org/10.1016/j.antiviral.2019.104541.
-
52.
Ko WC, Rolain JM, Lee NY, Chen PL, Huang CT, Lee PI, et al. Arguments in favour of remdesivir for treating SARS-CoV-2 infections. Int J Antimicrob Agents. 2020;55(4):105933. [PubMed ID: 32147516]. [PubMed Central ID: PMC7135364]. https://doi.org/10.1016/j.ijantimicag.2020.105933.
-
53.
de Wit E, Feldmann F, Cronin J, Jordan R, Okumura A, Thomas T, et al. Prophylactic and therapeutic remdesivir (GS-5734) treatment in the rhesus macaque model of MERS-CoV infection. Proc Natl Acad Sci U S A. 2020;117(12):6771-6. [PubMed ID: 32054787]. [PubMed Central ID: PMC7104368]. https://doi.org/10.1073/pnas.1922083117.
-
54.
Wu C, Liu Y, Yang Y, Zhang P, Zhong W, Wang Y, et al. Analysis of therapeutic targets for SARS-CoV-2 and discovery of potential drugs by computational methods. Acta Pharm Sin B. 2020;10(5):766-88. [PubMed ID: 32292689]. [PubMed Central ID: PMC7102550]. https://doi.org/10.1016/j.apsb.2020.02.008.
-
55.
Lai CC, Shih TP, Ko WC, Tang HJ, Hsueh PR. Severe acute respiratory syndrome coronavirus 2 (SARS-CoV-2) and coronavirus disease-2019 (COVID-19): The epidemic and the challenges. Int J Antimicrob Agents. 2020;55(3):105924. [PubMed ID: 32081636]. [PubMed Central ID: PMC7127800]. https://doi.org/10.1016/j.ijantimicag.2020.105924.
-
56.
Back D, Sekar V, Hoetelmans RM. Darunavir: pharmacokinetics and drug interactions. Antivir Ther. 2008;13(1):1-13. [PubMed ID: 18389894].
-
57.
McKeage K, Perry CM, Keam SJ. Darunavir: a review of its use in the management of HIV infection in adults. Drugs. 2009;69(4):477-503. [PubMed ID: 19323590]. https://doi.org/10.2165/00003495-200969040-00007.
-
58.
Hu Z, Song C, Xu C, Jin G, Chen Y, Xu X, et al. Clinical characteristics of 24 asymptomatic infections with COVID-19 screened among close contacts in Nanjing, China. Sci China Life Sci. 2020;63(5):706-11. [PubMed ID: 32146694]. [PubMed Central ID: PMC7088568]. https://doi.org/10.1007/s11427-020-1661-4.
-
59.
Dhama K, Sharun K, Tiwari R, Dadar M, Malik YS, Singh KP, et al. COVID-19, an emerging coronavirus infection: advances and prospects in designing and developing vaccines, immunotherapeutics, and therapeutics. Hum Vaccin Immunother. 2020;16(6):1232-8. [PubMed ID: 32186952]. [PubMed Central ID: PMC7103671]. https://doi.org/10.1080/21645515.2020.1735227.
-
60.
Dong L, Hu S, Gao J. Discovering drugs to treat coronavirus disease 2019 (COVID-19). Drug Discov Ther. 2020;14(1):58-60. [PubMed ID: 32147628]. https://doi.org/10.5582/ddt.2020.01012.
-
61.
Chang Y, Tung Y, Lee K, Chen T, Hsiao Y, Chang H, et al. Potential therapeutic agents for COVID-19 based on the analysis of protease and RNA polymerase docking. PrePrints. 2020. https://doi.org/10.20944/preprints202002.0242.v1.
-
62.
Chandel V, Raj S, Rathi B, Kumar D. In Silico Identification of Potent COVID-19 Main Protease Inhibitors from FDA Approved Antiviral Compounds and Active Phytochemicals through Molecular Docking: A Drug Repurposing Approach. PrePrints. 2020. https://doi.org/10.20944/preprints202003.0349.v1.
-
63.
Dayer MR. Old Drugs for Newly Emerging Viral Disease, COVID-19: Bioinformatic Prospective. arXiv. 2020.
-
64.
Redaelli S, Piazza R, Rostagno R, Magistroni V, Perini P, Marega M, et al. Activity of bosutinib, dasatinib, and nilotinib against 18 imatinib-resistant BCR/ABL mutants. J Clin Oncol. 2009;27(3):469-71. [PubMed ID: 19075254]. https://doi.org/10.1200/JCO.2008.19.8853.
-
65.
Wu Z, McGoogan JM. Characteristics of and Important Lessons From the Coronavirus Disease 2019 (COVID-19) Outbreak in China: Summary of a Report of 72314 Cases From the Chinese Center for Disease Control and Prevention. JAMA. 2020;323(13):1239-42. [PubMed ID: 32091533]. https://doi.org/10.1001/jama.2020.2648.
-
66.
Ang L, Lee HW, Choi JY, Zhang J, Soo Lee M. Herbal medicine and pattern identification for treating COVID-19: a rapid review of guidelines. Integr Med Res. 2020;9(2):100407. [PubMed ID: 32289016]. [PubMed Central ID: PMC7104236]. https://doi.org/10.1016/j.imr.2020.100407.
-
67.
Luo H, Tang QL, Shang YX, Liang SB, Yang M, Robinson N, et al. Can Chinese Medicine Be Used for Prevention of Corona Virus Disease 2019 (COVID-19)? A Review of Historical Classics, Research Evidence and Current Prevention Programs. Chin J Integr Med. 2020;26(4):243-50. [PubMed ID: 32065348]. [PubMed Central ID: PMC7088641]. https://doi.org/10.1007/s11655-020-3192-6.
-
68.
Amsden GW. Anti-inflammatory effects of macrolides--an underappreciated benefit in the treatment of community-acquired respiratory tract infections and chronic inflammatory pulmonary conditions? J Antimicrob Chemother. 2005;55(1):10-21. [PubMed ID: 15590715]. https://doi.org/10.1093/jac/dkh519.
-
69.
Nadeem Ahmed M, Muyot MM, Begum S, Smith P, Little C, Windemuller FJ. Antibiotic prescription pattern for viral respiratory illness in emergency room and ambulatory care settings. Clin Pediatr (Phila). 2010;49(6):542-7. [PubMed ID: 20075029]. https://doi.org/10.1177/0009922809357786.
-
70.
Wang D, Hu B, Hu C, Zhu F, Liu X, Zhang J, et al. Clinical Characteristics of 138 Hospitalized Patients With 2019 Novel Coronavirus-Infected Pneumonia in Wuhan, China. JAMA. 2020;323(11):1061-9. [PubMed ID: 32031570]. [PubMed Central ID: PMC7042881]. https://doi.org/10.1001/jama.2020.1585.
-
71.
Baron SA, Devaux C, Colson P, Raoult D, Rolain JM. Teicoplanin: an alternative drug for the treatment of COVID-19? Int J Antimicrob Agents. 2020;55(4):105944. [PubMed ID: 32179150]. [PubMed Central ID: PMC7102624]. https://doi.org/10.1016/j.ijantimicag.2020.105944.
-
72.
Zhang J, Ma X, Yu F, Liu J, Zou F, Pan T, et al. Teicoplanin potently blocks the cell entry of 2019-nCoV. bioRxiv. 2020. https://doi.org/10.1101/2020.02.05.935387.
-
73.
Cao B, Gao H, Zhou B, Deng X, Hu C, Deng C, et al. Adjuvant Corticosteroid Treatment in Adults With Influenza A (H7N9) Viral Pneumonia. Crit Care Med. 2016;44(6):e318-28. [PubMed ID: 26934144]. https://doi.org/10.1097/CCM.0000000000001616.
-
74.
Diaz E, Martin-Loeches I, Canadell L, Vidaur L, Suarez D, Socias L, et al. Corticosteroid therapy in patients with primary viral pneumonia due to pandemic (H1N1) 2009 influenza. J Infect. 2012;64(3):311-8. [PubMed ID: 22240033]. https://doi.org/10.1016/j.jinf.2011.12.010.
-
75.
Wang Y, Jiang W, He Q, Wang C, Wang B, Zhou P, et al. Early, low-dose and short-term application of corticosteroid treatment in patients with severe COVID-19 pneumonia: single-center experience from Wuhan, China. medRxiv. 2020. https://doi.org/10.1101/2020.03.06.20032342.
-
76.
Shang L, Zhao J, Hu Y, Du R, Cao B. On the use of corticosteroids for 2019-nCoV pneumonia. Lancet. 2020;395(10225):683-4. [PubMed ID: 32122468]. [PubMed Central ID: PMC7159292]. https://doi.org/10.1016/S0140-6736(20)30361-5.
-
77.
Chen C, Qi F, Shi K, Li Y, Li J, Chen Y, et al. Thalidomide combined with low‐dose short‐term glucocorticoid in the treatment of COVID-19 pneumonia. Clin Trans Med. 2020.
-
78.
Young BE, Ong SWX, Kalimuddin S, Low JG, Tan SY, Loh J, et al. Epidemiologic Features and Clinical Course of Patients Infected With SARS-CoV-2 in Singapore. JAMA. 2020;323(15):1488-94. [PubMed ID: 32125362]. [PubMed Central ID: PMC7054855]. https://doi.org/10.1001/jama.2020.3204.
-
79.
Yang X, Yu Y, Xu J, Shu H, Xia J, Liu H, et al. Clinical course and outcomes of critically ill patients with SARS-CoV-2 pneumonia in Wuhan, China: a single-centered, retrospective, observational study. Lancet Resp Med. 2020;8(5):475-81. https://doi.org/10.1016/s2213-2600(20)30079-5.
-
80.
Chen L, Xiong J, Bao L, Shi Y. Convalescent plasma as a potential therapy for COVID-19. Lancet Infect Dis. 2020;20(4):398-400. https://doi.org/10.1016/s1473-3099(20)30141-9.
-
81.
Tanne JH. Covid-19: FDA approves use of convalescent plasma to treat critically ill patients. BMJ. 2020;368:m1256. [PubMed ID: 32217555]. https://doi.org/10.1136/bmj.m1256.
-
82.
Petrosillo N, Nicastri E, Lanini S, Capobianchi MR, Di Caro A, Antonini M, et al. Ebola virus disease complicated with viral interstitial pneumonia: a case report. BMC Infect Dis. 2015;15:432. [PubMed ID: 26471197]. [PubMed Central ID: PMC4608352]. https://doi.org/10.1186/s12879-015-1169-4.
-
83.
Zhou B, Zhong N, Guan Y. Treatment with convalescent plasma for influenza A (H5N1) infection. N Engl J Med. 2007;357(14):1450-1. [PubMed ID: 17914053]. https://doi.org/10.1056/NEJMc070359.
-
84.
Arabi Y, Balkhy H, Hajeer AH, Bouchama A, Hayden FG, Al-Omari A, et al. Feasibility, safety, clinical, and laboratory effects of convalescent plasma therapy for patients with Middle East respiratory syndrome coronavirus infection: a study protocol. Springerplus. 2015;4:709. [PubMed ID: 26618098]. [PubMed Central ID: PMC4653124]. https://doi.org/10.1186/s40064-015-1490-9.
-
85.
Shen C, Wang Z, Zhao F, Yang Y, Li J, Yuan J, et al. Treatment of 5 Critically Ill Patients With COVID-19 With Convalescent Plasma. JAMA. 2020;323(16):1582-9. [PubMed ID: 32219428]. [PubMed Central ID: PMC7101507]. https://doi.org/10.1001/jama.2020.4783.
-
86.
Huang C, Wang Y, Li X, Ren L, Zhao J, Hu Y, et al. Clinical features of patients infected with 2019 novel coronavirus in Wuhan, China. Lancet. 2020;395(10223):497-506. https://doi.org/10.1016/s0140-6736(20)30183-5.