Abstract
Keywords
1. Context
Leishmaniasis is a series of chronic zoonotic infectious diseases characterized by broad-spectrum clinical manifestations ranging from simple cutaneous ulcers to severe forms such as multiple visceral organ involvement (1). Generally, infected hosts, like humans, experience three primary clinical forms: (1) visceral leishmaniasis (VL), (2) cutaneous leishmaniasis (CL), and (3) mucocutaneous leishmaniasis (MCL) (2, 3). Pentavalent antimonials, oral miltefosine, amphotericin B, liposomal amphotericin B, and paromomycin are most commonly used for chemotherapy, although they are not always effective and may exert various adverse side effects and drug resistance (4). Therefore, leishmaniasis vaccination and community immunization could be a more reasonable way to control or prevent Leishmania infection than current chemotherapy approaches (5). Most human patients with leishmaniasis will recover and become immune. Moreover, an ancient practical method, namely "leishmanization," provided further proof for leishmaniasis vaccine development (6).
Based on evidence regarding the historical path for vaccine development (Figure 1), the leishmaniasis vaccine can be universally categorized as first and second generations (7, 8). The initial vaccines containing unprocessed antigens from the body of the killed parasite have been administered with/without adjuvants such as BCG (Bacillus Calmette-Guerin). Although these vaccines are available, cost-effective, and easy to prepare, the standardization process limits their clinical use (9). Despite several clinical trials performed with these vaccines, their efficacy is still unknown (10). The second-generation candidates are based on chemically defined antigens. This classification includes recombinant proteins, DNA, and genetically engineered organisms. They tend to be more standardizable. Although some first-generation candidate vaccines, such as Leishvacin®, are safe (11) and immunogenic (12), their efficacy has not been fully elucidated (13). This study examines vaccine progressions and defects for finding effective solutions against leishmaniasis.
Historical path of vaccine development adapted from Delany et al., 2014 (7).
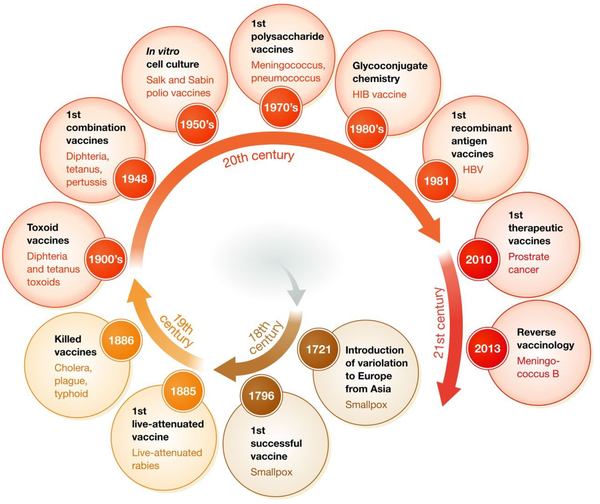
2. Leishmaniasis Vaccine Challenges
The development of a leishmaniasis vaccine remains a significant challenge (14). One restriction is the animal model, e.g., L. major murine model. Another significant restriction is the immune response adaptation (15). Although all the available effective vaccines against non-intracellular pathogens protect hosts via neutralizing antibodies, the designed vaccines for intracellular pathogens like Leishmania spp. are either not accessible or not completely successful (16). On the other hand, the lack of a decent interaction of immune protection is evident in leishmaniasis (14).
3. Vaccine Targets
Murine T helper (Th) cell clones are categorized into two main groups (Th1 and Th2) by different cytokine profiles (17). In the mouse model of L. major infection, these T helper cell subsets play critical roles in initiating and developing the pathological process. Sensitive mice develop Th1 and Th2 responses after infection, which leads to disease breakthrough and death (18). In this in-vivo model, the crucial role is played by IFN-γ and Th1 cells (18, 19). However, the same pattern is not observed in the Leishmania donovani, L. mexicana complexes, and other Leishmania species (20). Understanding leishmaniasis immunopathology and selecting candidate antigens are the most challenging matters in vaccine development (21).
During convolution, parasites have adapted to restrain or pervert the host's immune responses for their own favor (22). Specific Leishmania molecules play critical roles in this successful adaptation. The infectivity and survival of this parasite tie in the level of these molecules' expression. Virulence factors are studied as probable drug targets and vaccine candidates (23). Leishmania-activated C-kinase (LACK) is an antigen that induces a pro-inflammatory cytokine response of mononuclear cells in peripheral blood, significantly increasing IFN-γ and IL-10 (24). Antigens that induce regulatory responses may be suitable for further investigations (25).
New virulence factors identification can be accomplished at a vast, systemic scale by new tools such as bioinformatics software (Figure 2) (26). The vaccine advancement and immunotherapy strategies are intriguing. Recently, a DNA vaccine candidate could enhance the efficacy of liposomal Amphotericin B in L. donovani (27). This DNA vaccine was implicated in a combination of five minimalistic immunologically defined gene expression T helper cell 1 (MIDGE-Th1) vectors encoding different leishmanial antigens, including Kinotoplastida membrane protein-11 (KMP-11) and Cysteine proteinase type II (CPA) and type I (CPB) (28, 29).
Discovery of new virulence factors by applying both new experimental techniques (in vitro and in vivo study) and bioinformatics software (in silico study) adapted from Furman and Davis, 2015 (26).
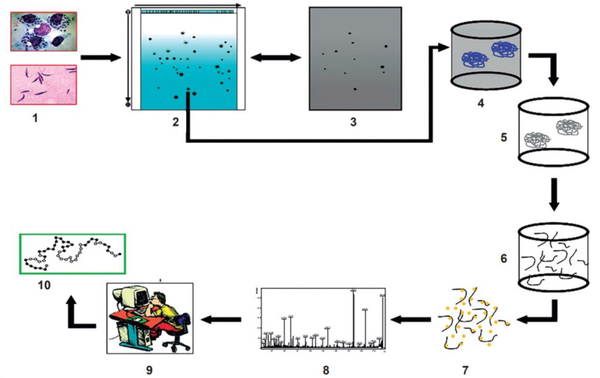
It is worth noting that all Leishmania spp. parasites can evade and even suppress the host's immune responses. These strategies restrict the vaccine's design and performance. Therefore, the parasite persists and establishes chronic infection. It was proven that cell-mediated immunity is the most important mechanism for establishing the protective and permanent immune response to leishmaniasis. The immune responses of Th1 and Th2 are associated with resistance and susceptibility to infection, respectively (30). Genetic variations of the host and parasite and other factors such as the location, inoculum size, and the number of infected bites received by the host are other factors influencing immune responses to leishmaniasis (31).
On the other hand, macrophages and/or dendritic cells (DCs) play critical roles as two dominant antigen-presenting cells (APCs), intervening in the resistance and susceptibility. These cells' performance could determine the initiation, development, and maintenance of protective immunity. It was proven that internalized amastigote forms in macrophages employ several mechanisms to alter cell-signaling pathways, finally suppressing inflammatory cytokine production (32). Protective immunity established by Th1 response and promoting early natural killer (NK) cell activities subsequent of IL-12 production by DCs (33). Nonetheless, the precise mechanisms of naive CD4+T cell differentiation are still unclear. The activity of granulocytes and other immune cells is increased during human VL (Figure 3) (34).
Immunological pathways against leishmaniasis adapted from Elshafie et al. 2011 (34).
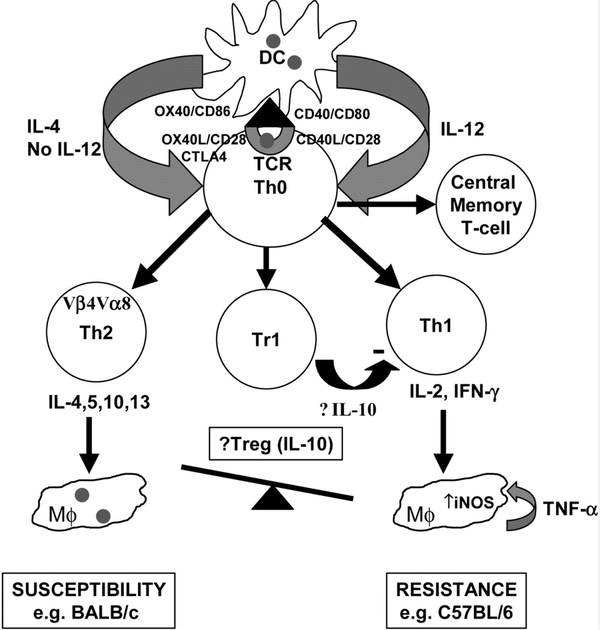
3.1. Approach 1: Recombinant Vaccines
Although there are different approaches to designing vaccines against leishmaniasis (Figure 4) (35), molecular approaches are investigated in this review. Recombinant protein vaccines are produced by genetically engineered cells (36). Numerous proteins have been evaluated as potential candidates. The first one is LEISH-F1, a protein conserved among several species of Leishmania, such as L. donovani and L. infantum/L. chagasi (4).
Different vaccines against leishmaniasis adapted from Srivastava et al. 2016 (35).
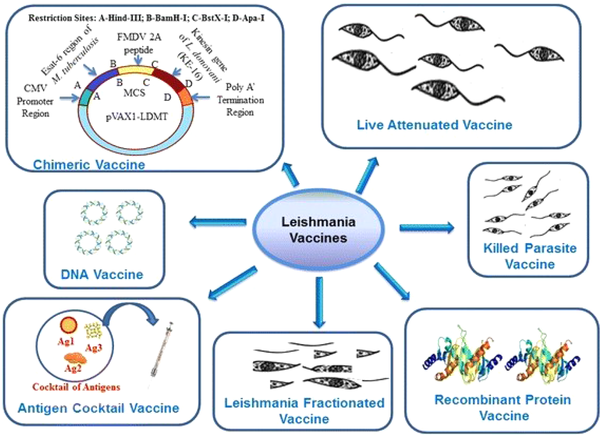
KMP-11 is another candidate that T cell anergy with IL-2 production has been found together with severe specific anti-KMP-11 (37). Recombinant Hydrophilic Acylated Surface Protein B1 (HASPB1) has demonstrated good protection in the animal model of VL (38). A notable (70%) delayed-type hypersensitivity (DTH) response to Leishmania antigen was revealed in vaccines compared to controls (39). Recent vaccines against leishmaniasis are listed in Table 1.
The List of Leishmaniasis Vaccines
Names | Infections | Type of Vaccine | Test Models | Mode of Protection | ||
---|---|---|---|---|---|---|
Protein | DNA | Partial | Complete | |||
KMP 11 | Leishmania mexicana | + | Rodent | + | ||
Leish F1 | L. donovani | + | Clinical trial | + | ||
Leish 111f | L. donovani | + | Rodent | + | ||
HASPB1 | L. donovani | + | Rodent | + | ||
Leish-f1+MPL-SE | L. infantum | + | Clinical trial | + | ||
rSMT | L. donovani | + | Rodent | + | ||
A2 | L. infantum | + | Rodent | + | ||
Leish-110f | L. infantum | + | Rodent | + | ||
LD91+LD72+ LD51+LD31 | L. donovani | + | Rodent | + | ||
(P-8 PGLC) | L. pifanoi | + | Dog | + | ||
rCDV-LACK, rCDV-TSA, and rCDV-LmSTI1 | L. Mexicana | + | Dog | + | ||
Gp63 | L. donovani | + | Rodent | + | ||
TRYP | L. infantum | + | Dog | + | ||
NH36 | L. donovani | + | Rodent | + | ||
Proteophosphoglycans (PPGs) | L. donovani | + | Rodent | + | ||
ORFF | L. donovani | + | Rodent | + | ||
LPG 3 | L. infantum | + | Rodent | + | ||
LEISHDNAVAX | L. infantum | + | Rodent | + |
Cysteine proteinase type III (CPC) is another target applied using a prime-boost strategy in susceptible mice (40). A clinical trial has evaluated Leish-111F. Three molecules are fused to form Leish-111F polyprotein, including L. major stress-inducible protein-1 (LmSTI1), L. braziliensis elongation and initiation factor (LeIF), and L. major homolog of eukaryotic thiol-specific antioxidant (TSA) (41). The high immunogenicity and promising protective efficacy of Leish-111F against L. infantum have been proven recently (42). In clinical trials, the Leish-111F vaccine is known as LeishF1 and has been evaluated in combination with MPL-SE adjuvant.
Leish-F1-MPL-SE was well-tolerated by volunteers with or without previous VL exposure, with no side effects (4). The acceptable protectivity effects of induction of Leish-111F formulated with special emulsion, provided infrastructure for designing clinical trials with Leish-111F/MPL-SE usage (Leish-111F-MPL-SE) (4). Up to 99.6% reduction in the parasite load in in-vivo models of immunized hamsters and mice approved that Leish-111F-MPL-SE induces appropriate immune response and protection against L. infantum infection.
The protective and immunostimulatory effects of prime-boost pORT-LACK/MVA-LACK have been proven. The recombinant L. major H2B protein and its amino- and carboxyl-terminal regions are two important candidates (43). BALB/c mice were vaccinated against L. infantum infection by a recombinant L. tarentolae that expressed L. donovani-specific A2 protein (44). Leishmania infantum sterol 24-c-methyltransferase (rSMT) has been introduced as an effective vaccine in an experimental model of VL (45).
Several unique antigenic proteins (S4, S6, L3, and L5) have been detected in the Leishmania ribosome, and the first study was performed in the cutaneous leishmaniasis in-vivo. The administration of L. major ribosomal proteins L3 (LmL3) or LmL5 combined with CpG-oligodeoxynucleotides (CpG-ODN) could protect BALB/c mice (46). Phlebotomus papatasi salivary protein 15 (PpSP15) is a vaccine candidate against L. major infection. Modality confers strong protection against L. major infection (47). Another in-vivo effort has recently been made to examine the efficacy of Leish-Tec®, an A2-based vaccine against visceral leishmaniasis (48).
3.2. Approach 2: Antigen Cocktail Vaccines
Entrapped leishmanial antigens, such as LD91 (91-kDa), LD72 (72-kDa), LD51 (51-kDa), and LD31 (31-kDa) in a cationic liposome as the adjuvant, were used as vaccines against leishmaniasis (49). The P-8 proteoglycolipid complex (P-8 PGLC), an amastigote antigen of L. pifanoi, could induce protection (50). Sterol 24-c-methyltransferase (SMT), cysteine proteinase B (CPB), KMP11, hydrophilic acylated surface protein B (K26/HASPB), A2, and nucleoside hydrolyses (NH) are suitable antigens for antigen cocktail vaccines. These antigens produce a Th1-type immune response (51). Also, the cocktail of rCDV-TSA, rCDV-LmSTI1, and rCDV-LACK has been investigated in the main reservoir (52). Recently, immunogenicity and protection effects of cationic liposomes containing imiquimod adjuvant have been assessed (53-55).
3.3. Approach 3: DNA and Chimerical DNA Vaccines
Due to their protein structure and antigenicity, DNA vaccines may be more effective against Leishmania than live-attenuated or killed vaccines (4). Although DNA vaccines lead to an immune response and protection against the antigen, their delivery is a significant concern (56). DNA vaccines have attracted researchers' attention as remarkable innovations in the immunization field due to their unique features (57). LACK is the most studied DNA vaccine against leishmaniasis. Heterogonous prime-boost with glycoprotein 63 (GP63) antigen provided durable protection against L. donovani correlated with intracellular immunity (58). GP63 is a major surface protein found in both forms (amastigote and promastigote) of parasites and is a good candidate for DNA vaccines (59).
The intramuscular DNA vaccine containing 10 antigens of Leishmania is a suitable DNA vaccine (60). One of the safe and immunogenic vaccines in outbred dogs is a prime/boost DNA/modified vaccinia virus Ankara vaccine, expressing recombinant Leishmania DNA encoding tryparedoxin peroxidase (TRYP) (61). Vaccination by nucleoside hydrolase-DNA vaccines against L. donovani has shown promising results as a novel approach for disease treatment and control (62).
The FMLSAP vaccine against L. donovani NH36 gene, induces a significant cross-protective response. For the prevention and control of infectious disease, the polytope approach of gene immunization is a valuable method to deliver T cell epitopes (63). The DNA encoding proteophosphoglycans (PPGs) gene was studied as a vaccine candidate. Leishmania antigens in cationic liposomes with non-coding pDNA-bearing immune-stimulatory lead to suitable protection against L. major (64). The open reading frame F (ORFF) protein, which is presented in both promastigote and amastigote forms, is another candidate for DNA vaccines (65).
Ubiquity conjugation of ORFF DNA leads to immune response (65). Lipophosphoglycan (LPG) is a parasite virulence factor with high immunogenicity (66). Recently, DNA vaccine performance has been assessed in L. amazonensis-infected animal models (67). The immunogenic effect of the multi-antigen T-cell epitope-enriched DNA vaccine, LEISHDNAVAX, has been demonstrated in the Leishmania-infected experimental model. DNA vaccination with LPG3 of L. infantum induces parasite-specific protective Th1 responses (66).
The L. donovani kinesis motor domain region is a possible vaccine candidate against VL (68). Intracellular transport of diverse cargoes and cell division is crucial in this system. Nearly 20% of VL patients will experience tuberculosis. A novel chimerical DNA vaccine candidate comprising the esat-6 gene of M. tuberculosis and the kinesis motor domain gene of L. donovani are attractive chimerical vaccines (69). As both M.tuberculosis and L. donovani are intracellular pathogens, the chimerical DNA construct leads to a cellular immune response. The novel chimerical DNA vaccine could be a potential immunoprotective candidate for conquering leishmaniasis (Figure 5) (69).
A novel chimeric DNA vaccine comprising the esat-6 gene of M. tuberculosis. B, The kinesin motor domain gene of Leishmania donovani adapted from Dey et al., 2009 (69).
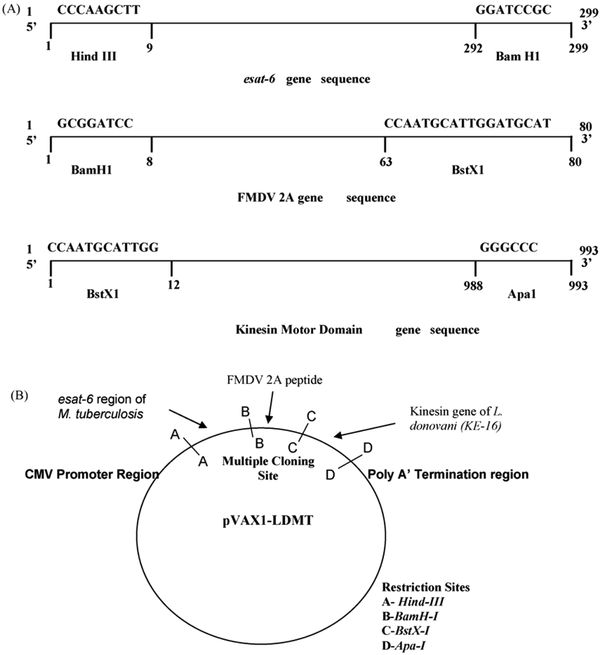
4. Conclusions
4.1. Future of Vaccines, Implications, and Restrictions
The primary step in vaccine advancement against diseases is shifting from laboratory testing to field studies. This process has various concerns, including safety and benefits in clinical usage. These constraints increase sophisticated human ethical concerns in the survey for appropriate vaccines. Moreover, vast knowledge of immunoprophylaxis and immunological memory maintenance is required to design scientific vaccine trials.
Acknowledgements
References
-
1.
Murray HW, Berman JD, Davies CR, Saravia NG. Advances in leishmaniasis. Lancet. 2005;366(9496):1561-77. [PubMed ID: 16257344]. https://doi.org/10.1016/S0140-6736(05)67629-5.
-
2.
Firouzeh N, Asadi A, Tavakoli Kareshk A. Nano amphotericin B: a good anti-leishmanial drug with effect on cathelicidin gene expression. J Parasit Dis. 2021;45(2):366-71. [PubMed ID: 34295035]. [PubMed Central ID: PMC8254690]. https://doi.org/10.1007/s12639-020-01308-3.
-
3.
Asadi A, Tavakoli Kareshk A, Sharifi I, Firouzeh N. Murine cathelicidin: as a host defensive response against Leishmania major infection. J Parasit Dis. 2020;44(3):633-8. [PubMed ID: 32801517]. [PubMed Central ID: PMC7410999]. https://doi.org/10.1007/s12639-020-01238-0.
-
4.
Chakravarty J, Kumar S, Trivedi S, Rai VK, Singh A, Ashman JA, et al. A clinical trial to evaluate the safety and immunogenicity of the LEISH-F1+MPL-SE vaccine for use in the prevention of visceral leishmaniasis. Vaccine. 2011;29(19):3531-7. [PubMed ID: 21414377]. https://doi.org/10.1016/j.vaccine.2011.02.096.
-
5.
Ikeogu NM, Akaluka GN, Edechi CA, Salako ES, Onyilagha C, Barazandeh AF, et al. Leishmania Immunity: Advancing Immunotherapy and Vaccine Development. Microorganisms. 2020;8(8). [PubMed ID: 32784615]. [PubMed Central ID: PMC7465679]. https://doi.org/10.3390/microorganisms8081201.
-
6.
Moafi M, Rezvan H, Sherkat R, Taleban R. Leishmania Vaccines Entered in Clinical Trials: A Review of Literature. Int J Prev Med. 2019;10:95. [PubMed ID: 31360342]. [PubMed Central ID: PMC6592111]. https://doi.org/10.4103/ijpvm.IJPVM_116_18.
-
7.
Delany I, Rappuoli R, De Gregorio E. Vaccines for the 21st century. EMBO Mol Med. 2014;6(6):708-20. [PubMed ID: 24803000]. [PubMed Central ID: PMC4203350]. https://doi.org/10.1002/emmm.201403876.
-
8.
Reed SG, Coler RN, Mondal D, Kamhawi S, Valenzuela JG. Leishmania vaccine development: exploiting the host-vector-parasite interface. Expert Rev Vaccines. 2016;15(1):81-90. [PubMed ID: 26595093]. [PubMed Central ID: PMC6019289]. https://doi.org/10.1586/14760584.2016.1105135.
-
9.
Evans KJ, Kedzierski L. Development of Vaccines against Visceral Leishmaniasis. J Trop Med. 2012;2012:892817. [PubMed ID: 21912561]. [PubMed Central ID: PMC3170777]. https://doi.org/10.1155/2012/892817.
-
10.
Noazin S, Khamesipour A, Moulton LH, Tanner M, Nasseri K, Modabber F, et al. Efficacy of killed whole-parasite vaccines in the prevention of leishmaniasis: a meta-analysis. Vaccine. 2009;27(35):4747-53. [PubMed ID: 19540273]. https://doi.org/10.1016/j.vaccine.2009.05.084.
-
11.
Marzochi KB, Marzochi MA, Silva AF, Grativol N, Duarte R, Confort EM, et al. Phase 1 study of an inactivated vaccine against American tegumentary leishmaniasis in normal volunteers in Brazil. Mem Inst Oswaldo Cruz. 1998;93(2):205-12. [PubMed ID: 9698895]. https://doi.org/10.1590/s0074-02761998000200014.
-
12.
De Luca PM, Mayrink W, Pinto JA, Coutinho SG, Santiago MA, Toledo VP, et al. A randomized double-blind placebo-controlled trial to evaluate the immunogenicity of a candidate vaccine against American tegumentary leishmaniasis. Acta Trop. 2001;80(3):251-60. [PubMed ID: 11700183]. https://doi.org/10.1016/s0001-706x(01)00181-4.
-
13.
Velez ID, Gilchrist K, Arbelaez MP, Rojas CA, Puerta JA, Antunes CM, et al. Failure of a killed Leishmania amazonensis vaccine against American cutaneous leishmaniasis in Colombia. Trans R Soc Trop Med Hyg. 2005;99(8):593-8. [PubMed ID: 15893351]. https://doi.org/10.1016/j.trstmh.2005.04.002.
-
14.
Kedzierski L, Zhu Y, Handman E. Leishmania vaccines: progress and problems. Parasitology. 2006;133 Suppl:S87-112. [PubMed ID: 17274851]. https://doi.org/10.1017/S0031182006001831.
-
15.
Howard JG, Liew FY. Mechanisms of acquired immunity in leishmaniasis. Philos Trans R Soc Lond B Biol Sci. 1984;307(1131):87-98. [PubMed ID: 6151691]. https://doi.org/10.1098/rstb.1984.0111.
-
16.
Zinkernagel RM. On natural and artificial vaccinations. Annu Rev Immunol. 2003;21:515-46. [PubMed ID: 12500980]. https://doi.org/10.1146/annurev.immunol.21.120601.141045.
-
17.
Mosmann TR, Cherwinski H, Bond MW, Giedlin MA, Coffman RL. Two types of murine helper T cell clone. I. Definition according to profiles of lymphokine activities and secreted proteins. J Immunol. 1986;136(7):2348-57. [PubMed ID: 2419430].
-
18.
Locksley RM, Heinzel FP, Sadick MD, Holaday BJ, Gardner KJ. Murine cutaneous leishmaniasis: susceptibility correlates with differential expansion of helper T-cell subsets. Ann Inst Pasteur Immunol. 1987;138(5):744-9. [PubMed ID: 2964247]. https://doi.org/10.1016/s0769-2625(87)80030-2.
-
19.
Moll H, Rollinghoff M. Resistance to murine cutaneous leishmaniasis is mediated by TH1 cells, but disease-promoting CD4+ cells are different from TH2 cells. Eur J Immunol. 1990;20(9):2067-74. [PubMed ID: 1976523]. https://doi.org/10.1002/eji.1830200927.
-
20.
McMahon-Pratt D, Alexander J. Does the Leishmania major paradigm of pathogenesis and protection hold for New World cutaneous leishmaniases or the visceral disease? Immunol Rev. 2004;201:206-24. [PubMed ID: 15361243]. https://doi.org/10.1111/j.0105-2896.2004.00190.x.
-
21.
Campos-Neto A. What about Th1/Th2 in cutaneous leishmaniasis vaccine discovery? Braz J Med Biol Res. 2005;38(7):979-84. [PubMed ID: 16007269]. https://doi.org/10.1590/s0100-879x2005000700001.
-
22.
Flora R, Aghazadeh-Dibavar S, Bandyopadhyay M, Dasgupta S. Immunosuppression during Leishmania donovani infection: a potential target for the development of therapy. Ann Parasitol. 2014;60(4):239-45. [PubMed ID: 25706420].
-
23.
Mottram JC, Coombs GH, Alexander J. Cysteine peptidases as virulence factors of Leishmania. Curr Opin Microbiol. 2004;7(4):375-81. [PubMed ID: 15358255]. https://doi.org/10.1016/j.mib.2004.06.010.
-
24.
Azeredo-Coutinho RB, Matos DC, Armoa GG, Maia RM, Schubach A, Mayrink W, et al. Contrasting human cytokine responses to promastigote whole-cell extract and the Leishmania analogue receptor for activated C kinase antigen of L. amazonensis in natural infection versus immunization. Clin Exp Immunol. 2008;153(3):369-75. [PubMed ID: 18627399]. [PubMed Central ID: PMC2527360]. https://doi.org/10.1111/j.1365-2249.2008.03705.x.
-
25.
Sacks D, Anderson C. Re-examination of the immunosuppressive mechanisms mediating non-cure of Leishmania infection in mice. Immunol Rev. 2004;201:225-38. [PubMed ID: 15361244]. https://doi.org/10.1111/j.0105-2896.2004.00185.x.
-
26.
Furman D, Davis MM. New approaches to understanding the immune response to vaccination and infection. Vaccine. 2015;33(40):5271-81. [PubMed ID: 26232539]. [PubMed Central ID: PMC4581990]. https://doi.org/10.1016/j.vaccine.2015.06.117.
-
27.
Seifert K, Juhls C, Salguero FJ, Croft SL. Sequential chemoimmunotherapy of experimental visceral leishmaniasis using a single low dose of liposomal amphotericin B and a novel DNA vaccine candidate. Antimicrob Agents Chemother. 2015;59(9):5819-23. [PubMed ID: 26055371]. [PubMed Central ID: PMC4538505]. https://doi.org/10.1128/AAC.00273-15.
-
28.
Lacerda DI, Cysne-Finkelstein L, Nunes MP, De-Luca PM, Genestra Mda S, Leon LL, et al. Kinetoplastid membrane protein-11 exacerbates infection with Leishmania amazonensis in murine macrophages. Mem Inst Oswaldo Cruz. 2012;107(2):238-45. [PubMed ID: 22415264]. https://doi.org/10.1590/s0074-02762012000200014.
-
29.
Mukhopadhyay S, Sen P, Majumder HK, Roy S. Reduced expression of lipophosphoglycan (LPG) and kinetoplastid membrane protein (KMP)-11 in Leishmania donovani promastigotes in axenic culture. J Parasitol. 1998;84(3):644-7. [PubMed ID: 9645879].
-
30.
Srivastava A, Singh N, Mishra M, Kumar V, Gour JK, Bajpai S, et al. Identification of TLR inducing Th1-responsive Leishmania donovani amastigote-specific antigens. Mol Cell Biochem. 2012;359(1-2):359-68. [PubMed ID: 21858498]. https://doi.org/10.1007/s11010-011-1029-5.
-
31.
Dunning N. Leishmania vaccines: from leishmanization to the era of DNA technology. Biosci Horiz. 2009;2(1):73-82. https://doi.org/10.1093/biohorizons/hzp004.
-
32.
Junghae M, Raynes JG. Activation of p38 mitogen-activated protein kinase attenuates Leishmania donovani infection in macrophages. Infect Immun. 2002;70(9):5026-35. [PubMed ID: 12183549]. [PubMed Central ID: PMC128247]. https://doi.org/10.1128/IAI.70.9.5026-5035.2002.
-
33.
Liese J, Schleicher U, Bogdan C. TLR9 signaling is essential for the innate NK cell response in murine cutaneous leishmaniasis. Eur J Immunol. 2007;37(12):3424-34. [PubMed ID: 18034422]. https://doi.org/10.1002/eji.200737182.
-
34.
Elshafie AI, Hlin E, Hakansson LD, Elghazali G, Safi SH, Ronnelid J, et al. Activity and turnover of eosinophil and neutrophil granulocytes are altered in visceral leishmaniasis. Int J Parasitol. 2011;41(3-4):463-9. [PubMed ID: 21172349]. https://doi.org/10.1016/j.ijpara.2010.11.005.
-
35.
Srivastava S, Shankar P, Mishra J, Singh S. Possibilities and challenges for developing a successful vaccine for leishmaniasis. Parasit Vectors. 2016;9(1):277. [PubMed ID: 27175732]. [PubMed Central ID: PMC4866332]. https://doi.org/10.1186/s13071-016-1553-y.
-
36.
Khamesipour A, Rafati S, Davoudi N, Maboudi F, Modabber F. Leishmaniasis vaccine candidates for development: a global overview. Indian J Med Res. 2006;123(3):423-38. [PubMed ID: 16778321].
-
37.
Basu R, Bhaumik S, Basu JM, Naskar K, De T, Roy S. Kinetoplastid membrane protein-11 DNA vaccination induces complete protection against both pentavalent antimonial-sensitive and -resistant strains of Leishmania donovani that correlates with inducible nitric oxide synthase activity and IL-4 generation: evidence for mixed Th1- and Th2-like responses in visceral leishmaniasis. J Immunol. 2005;174(11):7160-71. [PubMed ID: 15905560]. https://doi.org/10.4049/jimmunol.174.11.7160.
-
38.
Stager S, Smith DF, Kaye PM. Immunization with a recombinant stage-regulated surface protein from Leishmania donovani induces protection against visceral leishmaniasis. J Immunol. 2000;165(12):7064-71. [PubMed ID: 11120835]. https://doi.org/10.4049/jimmunol.165.12.7064.
-
39.
Nico D, Claser C, Borja-Cabrera GP, Travassos LR, Palatnik M, Soares IS, et al. Adaptive immunity against Leishmania nucleoside hydrolase maps its c-terminal domain as the target of the CD4+ T cell-driven protective response. PLoS Negl Trop Dis. 2010;4(11). e866. [PubMed ID: 21085470]. [PubMed Central ID: PMC2976684]. https://doi.org/10.1371/journal.pntd.0000866.
-
40.
Khoshgoo N, Zahedifard F, Azizi H, Taslimi Y, Alonso MJ, Rafati S. Cysteine proteinase type III is protective against Leishmania infantum infection in BALB/c mice and highly antigenic in visceral leishmaniasis individuals. Vaccine. 2008;26(46):5822-9. [PubMed ID: 18804512]. https://doi.org/10.1016/j.vaccine.2008.08.065.
-
41.
Coler RN, Reed SG. Second-generation vaccines against leishmaniasis. Trends Parasitol. 2005;21(5):244-9. [PubMed ID: 15837614]. https://doi.org/10.1016/j.pt.2005.03.006.
-
42.
Bertholet S, Goto Y, Carter L, Bhatia A, Howard RF, Carter D, et al. Optimized subunit vaccine protects against experimental leishmaniasis. Vaccine. 2009;27(50):7036-45. [PubMed ID: 19786136]. [PubMed Central ID: PMC2783536]. https://doi.org/10.1016/j.vaccine.2009.09.066.
-
43.
Meddeb-Garnaoui A, Toumi A, Ghelis H, Mahjoub M, Louzir H, Chenik M. Cellular and humoral responses induced by Leishmania histone H2B and its divergent and conserved parts in cutaneous and visceral leishmaniasis patients, respectively. Vaccine. 2010;28(7):1881-6. [PubMed ID: 20005858]. https://doi.org/10.1016/j.vaccine.2009.11.075.
-
44.
Mizbani A, Taheri T, Zahedifard F, Taslimi Y, Azizi H, Azadmanesh K, et al. Recombinant Leishmania tarentolae expressing the A2 virulence gene as a novel candidate vaccine against visceral leishmaniasis. Vaccine. 2009;28(1):53-62. [PubMed ID: 19818721]. https://doi.org/10.1016/j.vaccine.2009.09.114.
-
45.
Goto Y, Bogatzki LY, Bertholet S, Coler RN, Reed SG. Protective immunization against visceral leishmaniasis using Leishmania sterol 24-c-methyltransferase formulated in adjuvant. Vaccine. 2007;25(42):7450-8. [PubMed ID: 17804125]. [PubMed Central ID: PMC2077354]. https://doi.org/10.1016/j.vaccine.2007.08.001.
-
46.
Ramirez L, Santos DM, Souza AP, Coelho EA, Barral A, Alonso C, et al. Evaluation of immune responses and analysis of the effect of vaccination of the Leishmania major recombinant ribosomal proteins L3 or L5 in two different murine models of cutaneous leishmaniasis. Vaccine. 2013;31(9):1312-9. [PubMed ID: 23313653]. https://doi.org/10.1016/j.vaccine.2012.12.071.
-
47.
Katebi A, Gholami E, Taheri T, Zahedifard F, Habibzadeh S, Taslimi Y, et al. Leishmania tarentolae secreting the sand fly salivary antigen PpSP15 confers protection against Leishmania major infection in a susceptible BALB/c mice model. Mol Immunol. 2015;67(2 Pt B):501-11. [PubMed ID: 26298575]. https://doi.org/10.1016/j.molimm.2015.08.001.
-
48.
Testasicca MC, dos Santos MS, Machado LM, Serufo AV, Doro D, Avelar D, et al. Antibody responses induced by Leish-Tec(R), an A2-based vaccine for visceral leishmaniasis, in a heterogeneous canine population. Vet Parasitol. 2014;204(3-4):169-76. [PubMed ID: 24863572]. https://doi.org/10.1016/j.vetpar.2014.04.025.
-
49.
Bhowmick S, Ali N. Identification of novel Leishmania donovani antigens that help define correlates of vaccine-mediated protection in visceral leishmaniasis. PLoS One. 2009;4(6). e5820. [PubMed ID: 19503834]. [PubMed Central ID: PMC2686101]. https://doi.org/10.1371/journal.pone.0005820.
-
50.
Carrillo E, Ahmed S, Goldsmith-Pestana K, Nieto J, Osorio Y, Travi B, et al. Immunogenicity of the P-8 amastigote antigen in the experimental model of canine visceral leishmaniasis. Vaccine. 2007;25(8):1534-43. [PubMed ID: 17178178]. [PubMed Central ID: PMC2571115]. https://doi.org/10.1016/j.vaccine.2006.10.036.
-
51.
Kumar R, Goto Y, Gidwani K, Cowgill KD, Sundar S, Reed SG. Evaluation of ex vivo human immune response against candidate antigens for a visceral leishmaniasis vaccine. Am J Trop Med Hyg. 2010;82(5):808-13. [PubMed ID: 20439959]. [PubMed Central ID: PMC2861380]. https://doi.org/10.4269/ajtmh.2010.09-0341.
-
52.
Miura R, Kooriyama T, Yoneda M, Takenaka A, Doki M, Goto Y, et al. Efficacy of Recombinant Canine Distemper Virus Expressing Leishmania Antigen against Leishmania Challenge in Dogs. PLoS Negl Trop Dis. 2015;9(7). e0003914. [PubMed ID: 26162094]. [PubMed Central ID: PMC4498809]. https://doi.org/10.1371/journal.pntd.0003914.
-
53.
Mehravaran A, Rezaei Nasab M, Mirahmadi H, Sharifi I, Alijani E, Nikpoor AR, et al. Immunogenicity and protection effects of cationic liposome containing imiquimod adjuvant on leishmaniasis in BALB/c mice. Iran J Basic Med Sci. 2019;22(8):922-31. [PubMed ID: 31579449]. [PubMed Central ID: PMC6760488]. https://doi.org/10.22038/ijbms.2019.35739.8515.
-
54.
Mehravaran A, Mirahmadi H, Akhtari J. Liposomes containing the imiquimod adjuvant as a vaccine in the cutaneous leishmaniasis model. Nanomed J. 2020;7(1):29-39.
-
55.
Mehravaran A, Nasab MR, Mirahmadi H, Sharifi I, Alijani E, Nikpoor AR, et al. Protection induced by Leishmania Major antigens and the imiquimod adjuvant encapsulated on liposomes in experimental cutaneous leishmaniasis. Infect Genet Evol. 2019;70:27-35. [PubMed ID: 30738195]. https://doi.org/10.1016/j.meegid.2019.01.005.
-
56.
Liu MA, Wahren B, Karlsson Hedestam GB. DNA vaccines: recent developments and future possibilities. Hum Gene Ther. 2006;17(11):1051-61. [PubMed ID: 17032152]. https://doi.org/10.1089/hum.2006.17.1051.
-
57.
Dumonteil E. DNA Vaccines against Protozoan Parasites: Advances and Challenges. J Biomed Biotechnol. 2007;2007(6):90520. [PubMed ID: 17710244]. [PubMed Central ID: PMC1940056]. https://doi.org/10.1155/2007/90520.
-
58.
Mazumder S, Maji M, Das A, Ali N. Potency, efficacy and durability of DNA/DNA, DNA/protein and protein/protein based vaccination using gp63 against Leishmania donovani in BALB/c mice. PLoS One. 2011;6(2). e14644. [PubMed ID: 21311597]. [PubMed Central ID: PMC3032732]. https://doi.org/10.1371/journal.pone.0014644.
-
59.
Sinha S, Sundaram S, Singh AP, Tripathi A. A gp63 based vaccine candidate against Visceral Leishmaniasis. Bioinformation. 2011;5(8):320-5. [PubMed ID: 21383918]. [PubMed Central ID: PMC3046035]. https://doi.org/10.6026/97320630005320.
-
60.
Saldarriaga OA, Travi BL, Park W, Perez LE, Melby PC. Immunogenicity of a multicomponent DNA vaccine against visceral leishmaniasis in dogs. Vaccine. 2006;24(11):1928-40. [PubMed ID: 16310897]. https://doi.org/10.1016/j.vaccine.2005.10.052.
-
61.
Carson C, Antoniou M, Ruiz-Arguello MB, Alcami A, Christodoulou V, Messaritakis I, et al. A prime/boost DNA/Modified vaccinia virus Ankara vaccine expressing recombinant Leishmania DNA encoding TRYP is safe and immunogenic in outbred dogs, the reservoir of zoonotic visceral leishmaniasis. Vaccine. 2009;27(7):1080-6. [PubMed ID: 19095029]. [PubMed Central ID: PMC2663027]. https://doi.org/10.1016/j.vaccine.2008.11.094.
-
62.
Gamboa-Leon R, Paraguai de Souza E, Borja-Cabrera GP, Santos FN, Myashiro LM, Pinheiro RO, et al. Immunotherapy against visceral leishmaniasis with the nucleoside hydrolase-DNA vaccine of Leishmania donovani. Vaccine. 2006;24(22):4863-73. [PubMed ID: 16635538]. https://doi.org/10.1016/j.vaccine.2006.03.005.
-
63.
Souza LOP, Palatnik de Sousa CB. The Nucleoside hydrolase DNA vaccine VR1012NH36 in prophylactic vaccination against mice tegumentar leishmaniasis. Procedia Vaccinol. 2009;1(1):120-3. https://doi.org/10.1016/j.provac.2009.07.022.
-
64.
Mazumder S, Ravindran R, Banerjee A, Ali N. Non-coding pDNA bearing immunostimulatory sequences co-entrapped with leishmanial antigens in cationic liposomes elicits almost complete protection against experimental visceral leishmaniasis in BALB/c mice. Vaccine. 2007;25(52):8771-81. [PubMed ID: 18031874]. https://doi.org/10.1016/j.vaccine.2007.10.028.
-
65.
Sharma A, Madhubala R. Ubiquitin conjugation of open reading frame F DNA vaccine leads to enhanced cell-mediated immune response and induces protection against both antimony-susceptible and -resistant strains of Leishmania donovani. J Immunol. 2009;183(12):7719-31. [PubMed ID: 19933862]. https://doi.org/10.4049/jimmunol.0900132.
-
66.
Pirdel L, Hosseini AZ, Rasouli M. Immune response in susceptible BALB/c mice immunized with DNA encoding Lipophosphoglycan 3 of Leishmania infantum. Parasite Immunol. 2014;36(12):700-7. [PubMed ID: 25244583]. https://doi.org/10.1111/pim.12147.
-
67.
Campos BL, Silva TN, Ribeiro SP, Carvalho KI, Kallas EG, Laurenti MD, et al. Analysis of iron superoxide dismutase-encoding DNA vaccine on the evolution of the Leishmania amazonensis experimental infection. Parasite Immunol. 2015;37(8):407-16. [PubMed ID: 26040192]. https://doi.org/10.1111/pim.12206.
-
68.
Sivakumar R, Dey A, Sharma P, Singh S. Expression and characterization of a recombinant kinesin antigen from an old Indian strain (DD8) of Leishmania donovani and comparing it with a commercially available antigen from a newly isolated (KE16) strain of L. donovani. Infect Genet Evol. 2008;8(3):313-22. [PubMed ID: 18374635]. https://doi.org/10.1016/j.meegid.2008.02.004.
-
69.
Dey A, Kumar U, Sharma P, Singh S. Immunogenicity of candidate chimeric DNA vaccine against tuberculosis and leishmaniasis. Vaccine. 2009;27(37):5152-60. [PubMed ID: 19559111]. https://doi.org/10.1016/j.vaccine.2009.05.100.