Abstract
Background:
Tuberculosis (TB) is an infectious disease caused by Mycobacterium tuberculosis.Objectives:
Development of the drug resistance is becoming a threat to disease control, which underscores need for new agents targeting M. tuberculosis.Materials and Methods:
In this study, analysis of gene expression was performed using real-time polymerase chain reaction (RT- PCR).Results:
Results of the current study showed that the minimum inhibitory concentration value of cinnamaldehyde against M. tuberculosis was 200 µg/mL. Moreover, RT-PCR data showed that a total of 25 genes were regulated by the cinnamaldehyde. Of these, 12 genes were up-regulated, and 13 genes were down-regulated.Conclusions:
Cinnamaldehyde is a pattern to expand the new anti-TB drugs, because the targets of the cinnamaldehyde are different from those of anti-tubercular agents.Keywords
Antimycobacterial Activity Cinnamaldehyde Medicinal Plant Mycobacterium tuberculosis
1. Background
The tuberculosis (TB) disease is still among world’s leading infectious diseases (1). Although accessible antimicrobial agents are effective at eradicating infections, there are issues with emergence of drug-resistant M. tuberculosis strains (2). Additionally, increased incidence of HIV infection has been shown to be associated with an increased mortality rate (3).
Some plants have been proven to be the sources of useful drugs. Cinnamaldehyde (Figure 1) has been elicited from several plants, such as Cinnamomum cassia. The cinnamaldehyde has been used in traditional medicine and has been demonstrated to suppress the growth of Clostridium botulinum (4), and Staphylococcus aureus (5). A few studies have reported on mechanisms underlying the effects of antimycobacterial agents (6).
Chemical Formula of Cinnamaldehdye
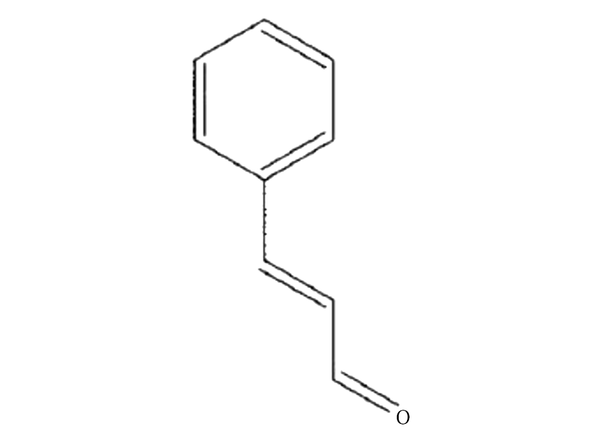
2. Objectives
The aim of this study was to determine antimycobacterial effects of cinnamaldehyde.
3. Materials and Methods
3.1. Bacterial Strains and Reagents
The cinnamaldehyde was supplied by Sigma-Aldrich chemical company. Mycobacterium tuberculosis was obtained from Zabol University of Medical Sciences.
3.2. Growth Curve of Mycobacterium tuberculosis With Different Concentrations of the Cinnamaldehyde
Mycobacterium tuberculosis was grown in a flask (100 mL) containing the Middlebrook 7H9 medium. Then, 1 mL of culture was placed in each of tubes and exposed to different concentrations of the cinnamaldehyde.
3.3. Cell Culture and Treatment by Quantitative Real-Time Polymerase Chain Reaction
Mycobacterium tuberculosis was grown in the Middlebrook 7H9 broth. The drug treatments were carried out by adding stock solution to one of the cultures.
3.4. Quantification of Gene Expression by Real-Time Polymerase Chain Reaction
Real-time PCR reactions were carried out using a Rotor-Gene 6000. Primers used are shown in Table 1. Analyses were carried out using the relative expression software tool (RESTª) software as described by Pfaffl et al. (7).
Primers Used in the Quantitative Real-Time Polymerase Chain Reaction Studies
Gene Name | Sense Primer | Antisense Primer |
---|---|---|
frdA | ACGAGCACAACAAAGGTACGA | AGGTGCAGCAGGTCTAGATTGA |
frdB | CTTGAATCTCGGTGAAAGAC | ACCGATTTGACCAGATCATC |
frdC | AGGAGATGCTGACTGAGAT | CTAGCGCCTCATTAAGATT |
frdD | GCAACCCGATCACCAAGCTAGAT | CATGGTCGAGCACGAACCGCATC |
narJ | CACCCACTACGCCAATATCT | AGCGGTACCACACTGACAC |
narI | GAGTCCTCGTCGGAGATATG | GAACCCGAGGTGAATATATC |
narH | GTTTGTGGGTTGACTATG | CGGTTTGCCATACATTGAAG |
narG | GCTAGTGATCGAACACAA | CAACCCCAAGTACAACA |
pks3 | TCCACACTCGCTACTAATAG | TATCCACCAGTACAGCACAT |
papA3 | CTTTGAGGTTGTCGCGACAC | CGACGAACACCAGCATAAT |
Mmpl10 | ACTCGGCGTATATCTGAAGG | CTGTCCATACGGGTCAAAGT |
rpsH | GAGCGTCAAGTCAGTATAGA | CAACTGATAGGTGGCGTCGT |
rpsS | CAAGGCTAAAACTCACACGA | GGACTTAACCCAACATATAA |
rplW | CTGCATCTGCGACTAAGTA | TCGATGGACAAGGTGTAGAA |
rplT | ACCTTCGAGGACCTGAAATT | CCTCCACCACAACTACGTAT |
rplO | GCAAGACAAGTGCTGATCAA | GAAGCGCTCAAAGAAACAAC |
rplD | GTGGACGGCGATTGAAAATT | AGCTGGCTGGGTGAACGC |
rplB | GGTAGCCAGCAAATAATACT | GCCAAGATGGTGTAGAGAAT |
infC | AGACCGTCGTCATAGAACAATAG | TTGGTCTCGTAATCGAGATAGT |
rho | AGGTCATCCAGCAGTATATG | TTGGTCACCGAAATAGAAGA |
argR | GGTGTTTGTGTTGTCAATAC | TAGCGCATCAGGATGAACAA |
argJ | GGATGACCTGCATAAGCAAT | GAAGCGCTCAAAGATACGTC |
argG | CCAAATGGCCAGATATCAAC | CGAGCACCTGTTGTTAGTG |
argF | CTACCACCGCCGTCTATATA | CCCTCTTCCACTGACATGAT |
argD | GCGATGTATCAGTCAGTAAG | GCATTGGCGACTACAATG |
4. Results
The bacteria were exposed to the different concentrations of cinnamaldehyde. The results in Figure 2 showed that 200 μg/mL of the cinnamaldehyde was the minimum inhibitory concentration (MIC) of the cinnamaldehyde, which inhibited the growth of M. tuberculosis. Therefore, M. tuberculosis was exposed to 200 μg/mL of the cinnamaldehyde in order to promote an alteration in gene expression of bacteria.
Real-time PCR was used to analyze gene expression in M. tuberculosis. Overall, there were 25 genes differentially regulated by the cinnamaldehyde. Among these, 12 genes exhibited a significant increase in the transcription and 13 exhibited a significant decrease in the transcription (Table 2).
Mycobacterium tuberculosis Exposed to Different Concentrations of Cinnamaldehdye
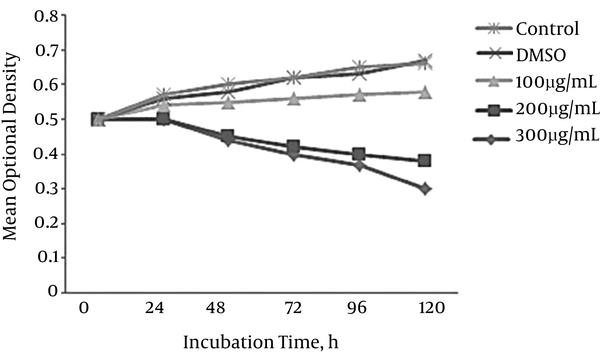
Gene Name | Description | Fold Change |
---|---|---|
frdA | Fumarate reductase subunit A | -3.1 |
frdB | Fumarate reductase subunit B | -3.4 |
frdC | Fumarate reductase subunit C | -3.2 |
frdD | Fumarate reductase subunit D | -3.3 |
narJ | Nitrate respiration | -3.3 |
narI | Nitrate respiration | -3.0 |
narH | Nitrate respiration | -3.5 |
narG | Nitrate respiration | -3.2 |
pks3 | Biosythesis of polyacyltrehalose | +3.9 |
papA3 | Biosythesis of polyacyltrehalose | +3.4 |
Mmpl10 | Biosythesis of polyacyltrehalose | +4.1 |
rpsH | Ribosome proteins | +3.5 |
rpsS | Ribosome proteins | +3.3 |
rplW | Ribosome proteins | +3.3 |
rplT | Ribosome proteins | +3.5 |
rplO | Ribosome proteins | +3.2 |
rplD | Ribosome proteins | +3.2 |
rplB | Ribosome proteins | +3.9 |
infC | Translation initiation factor IF-3 | +3.7 |
rho | Ribosome proteins | +3.4 |
argR | Arginine biosynthesis | -3.5 |
argJ | Arginine biosynthesis | -3.2 |
argG | Arginine biosynthesis | -3.1 |
argF | Arginine biosynthesis | -3.1 |
argD | Arginine biosynthesis | -3.4 |
5. Discussion
Four genes of frdA, frdB, frdC and frdD were down-regulated by 3.1-fold, 3.4-fold, 3.2-fold and 3.3-fold, respectively. The frdA genus has been found to be up-regulated in the M. tuberculosis in (7) as well as in studies that investigated the behaviour of the M. tuberculosis grown under carbon starvation (8). Studies on the behaviour of Mycobacterium phlei found that fumarate reductase (FRD) activity increased fourfold when the bacteria were grown under the low-oxygen conditions (9, 10). Fumarate reductase has reported to be a successful target in treatment of protozoan infections using a variety of compounds (11).
Nitrate reductase is a membrane-bound molybdenum-containing complex (12). Absorption of nitrogen into the mycobacterial metabolism is important for survival of M. tuberculosis (13). It is suggested that the narGHJI mediates nitrate absorption in M. tuberculosis (14).
The pks3 and mmpL10 genes were up-regulated by 3.9-fold and 4.0-fold, respectively. Suppression of papA3 gene expression from the M. tuberculosis resulted in loss of penta-acylated trehalose (PAT) (15-17). Pks3 is a polyketide synthase that is included in PAT biosynthesis of M. tuberculosis (18-20).
The rpsH and rpsS genes were up-regulated by 3.5-fold and 3.3-fold, respectively. Initiation factor infC was up-regulated by 3.7-fold and the transcription level of gene rho was also elevated by 3.4-fold. A work indicated that Clostridium difficile challenge to several concentrations of antibiotics resulted in a general up-regulation of translation machinery (21, 22).
L-arginine metabolism has indicated to be important for the M. tuberculosis (23). Some genes such as argR, argJ, argG, argF and argD were inhibited by 3.5-fold, 3.2-fold, 3.1-fold, 3.1-fold and 3.4-fold, respectively. Suppression of gene expression argR in Legionella pneumophila can affect gene transcript levels predicted to encode terminal steps of L-arginine biosynthesis (24). In Listeria monocytogenes, mutation of argD led to a decreased replication rates in Caco-2 cells (25). In Corynebacterium glutamicum, genes encoding L-arginine biosynthesis proteins showed a decreased expression in ammonium-limited chemostat cultures (26, 27).
5.1. Conclusions
The findings of the current study show that the cinnamaldehyde has potential antimycobacterial properties.
Acknowledgements
References
-
1.
Rohde KH, Abramovitch RB, Russell DG. Mycobacterium tuberculosis invasion of macrophages: linking bacterial gene expression to environmental cues. Cell Host Microbe. 2007;2(5):352-64. [PubMed ID: 18005756]. https://doi.org/10.1016/j.chom.2007.09.006.
-
2.
Pfaffl MW, Horgan GW, Dempfle L. Relative expression software tool (REST) for group-wise comparison and statistical analysis of relative expression results in real-time PCR. Nucleic Acids Res. 2002;30(9). eeee36. [PubMed ID: 11972351].
-
3.
Omura S, Miyadera H, Ui H, Shiomi K, Yamaguchi Y, Masuma R, et al. An anthelmintic compound, nafuredin, shows selective inhibition of complex I in helminth mitochondria. Proc Natl Acad Sci U S A. 2001;98(1):60-2. [PubMed ID: 11120889]. https://doi.org/10.1073/pnas.011524698.
-
4.
Bowles BL, Sackitey SK, Williams AC. Inhibitory effects of flavor compounds on Staphylococcus aureus. J Food Saf. 1995;15:337-47.
-
5.
Dennis PP. Effects of chloramphenicol on the transcriptional activities of ribosomal RNA and ribosomal protein genes in Escherichia coli. J Mol Biol. 1976;108(3):535-46. [PubMed ID: 798033].
-
6.
Betts JC, Lukey PT, Robb LC, McAdam RA, Duncan K. Evaluation of a nutrient starvation model of Mycobacterium tuberculosis persistence by gene and protein expression profiling. Mol Microbiol. 2002;43(3):717-31. [PubMed ID: 11929527].
-
7.
Raman S, Song T, Puyang X, Bardarov S, Jacobs WRJr, Husson RN. The alternative sigma factor SigH regulates major components of oxidative and heat stress responses in Mycobacterium tuberculosis. J Bacteriol. 2001;183(20):6119-25. [PubMed ID: 11567012]. https://doi.org/10.1128/JB.183.20.6119-6125.2001.
-
8.
Bowles BL, Miller AJ. Antibotulinal properties of selected aromatic and aliphatic aldehydes. J Food Prot. 1993;56(9):788-94.
-
9.
Golby P, Hatch KA, Bacon J, Cooney R, Riley P, Allnutt J, et al. Comparative transcriptomics reveals key gene expression differences between the human and bovine pathogens of the Mycobacterium tuberculosis complex. Microbiology. 2007;153(Pt 10):3323-36. [PubMed ID: 17906132]. https://doi.org/10.1099/mic.0.2007/009894-0.
-
10.
Raviglione MC. The TB epidemic from 1992 to 2002. Tuberculosis. 2003;83:4-14.
-
11.
Ormerod LP. Multidrug-resistant tuberculosis (MDR-TB): epidemiology, prevention and treatment. Br Med Bull. 2005;73-74:17-24. [PubMed ID: 15956357]. https://doi.org/10.1093/bmb/ldh047.
-
12.
Nunn P, Williams B, Floyd K, Dye C, Elzinga G, Raviglione M. Tuberculosis control in the era of HIV. Nat Rev Immunol. 2005;5(10):819-26. [PubMed ID: 16200083]. https://doi.org/10.1038/nri1704.
-
13.
Matsuda H, Tomohiro N, Ido Y, Kubo M. Anti-allergic effects of cnidii monnieri fructus (dried fruits of Cnidium monnieri) and its major component, osthol. Biol Pharm Bull. 2002;25(6):809-12. [PubMed ID: 12081154].
-
14.
de la Fuente A, Martin JF, Rodriguez-Garcia A, Liras P. Two proteins with ornithine acetyltransferase activity show different functions in Streptomyces clavuligerus: Oat2 modulates clavulanic acid biosynthesis in response to arginine. J Bacteriol. 2004;186(19):6501-7. [PubMed ID: 15375131]. https://doi.org/10.1128/JB.186.19.6501-6507.2004.
-
15.
Gordhan BG, Smith DA, Alderton H, McAdam RA, Bancroft GJ, Mizrahi V. Construction and phenotypic characterization of an auxotrophic mutant of Mycobacterium tuberculosis defective in L-arginine biosynthesis. Infect Immun. 2002;70(6):3080-4. [PubMed ID: 12011001].
-
16.
Hovel-Miner G, Faucher SP, Charpentier X, Shuman HA. ArgR-regulated genes are derepressed in the Legionella-containing vacuole. J Bacteriol. 2010;192(17):4504-16. [PubMed ID: 20622069]. https://doi.org/10.1128/JB.00465-10.
-
17.
Schnappinger D, Ehrt S, Voskuil MI, Liu Y, Mangan JA, Monahan IM, et al. Transcriptional Adaptation of Mycobacterium tuberculosis within Macrophages: Insights into the Phagosomal Environment. J Exp Med. 2003;198(5):693-704. [PubMed ID: 12953091]. https://doi.org/10.1084/jem.20030846.
-
18.
Dubourdieu M, DeMoss JA. The narJ gene product is required for biogenesis of respiratory nitrate reductase in Escherichia coli. J Bacteriol. 1992;174(3):867-72. [PubMed ID: 1732220].
-
19.
Emerson JE, Stabler RA, Wren BW, Fairweather NF. Microarray analysis of the transcriptional responses of Clostridium difficile to environmental and antibiotic stress. J Med Microbiol. 2008;57(Pt 6):757-64. [PubMed ID: 18480334]. https://doi.org/10.1099/jmm.0.47657-0.
-
20.
Nishimura T, Teramoto H, Inui M, Yukawa H. Corynebacterium glutamicum ArnR controls expression of nitrate reductase operon narKGHJI and nitric oxide (NO)-detoxifying enzyme gene hmp in an NO-responsive manner. J Bacteriol. 2014;196(1):60-9. [PubMed ID: 24142248]. https://doi.org/10.1128/JB.01004-13.
-
21.
Dubey VS, Sirakova TD, Kolattukudy PE. Disruption of msl3 abolishes the synthesis of mycolipanoic and mycolipenic acids required for polyacyltrehalose synthesis in Mycobacterium tuberculosis H37Rv and causes cell aggregation. Mol Microbiol. 2002;45(5):1451-9. [PubMed ID: 12207710].
-
22.
Gillespie J, Barton LL, Rypka EW. Influence of oxygen tension on the respiratory activity of Mycobacterium phlei. J Gen Microbiol. 1988;134(1):247-52. [PubMed ID: 3183614]. https://doi.org/10.1099/00221287-134-1-247.
-
23.
Hatzios SK, Schelle MW, Holsclaw CM, Behrens CR, Botyanszki Z, Lin FL, et al. PapA3 is an acyltransferase required for polyacyltrehalose biosynthesis in Mycobacterium tuberculosis. J Biol Chem. 2009;284(19):12745-51. [PubMed ID: 19276083]. https://doi.org/10.1074/jbc.M809088200.
-
24.
Joseph B, Przybilla K, Stuhler C, Schauer K, Slaghuis J, Fuchs TM, et al. Identification of Listeria monocytogenes genes contributing to intracellular replication by expression profiling and mutant screening. J Bacteriol. 2006;188(2):556-68. [PubMed ID: 16385046]. https://doi.org/10.1128/JB.188.2.556-568.2006.
-
25.
Malm S, Tiffert Y, Micklinghoff J, Schultze S, Joost I, Weber I, et al. The roles of the nitrate reductase NarGHJI, the nitrite reductase NirBD and the response regulator GlnR in nitrate assimilation of Mycobacterium tuberculosis. Microbiology. 2009;155(Pt 4):1332-9. [PubMed ID: 19332834]. https://doi.org/10.1099/mic.0.023275-0.
-
26.
Domenech P, Reed MB, Barry CE. Contribution of the Mycobacterium tuberculosis MmpL protein family to virulence and drug resistance. Infect Immun. 2005;73(6):3492-501. [PubMed ID: 15908378]. https://doi.org/10.1128/IAI.73.6.3492-3501.2005.
-
27.
Slayden RA, Knudson DL, Belisle JT. Identification of cell cycle regulators in Mycobacterium tuberculosis by inhibition of septum formation and global transcriptional analysis. Microbiology. 2006;152(Pt 6):1789-97. [PubMed ID: 16735741]. https://doi.org/10.1099/mic.0.28762-0.