Abstract
Background:
Cystic fibrosis (CF) is an autosomal recessive disease characterized by respiratory, gastrointestinal, and reproductive tract problems. Patients with CF often suffer from chronic pulmonary infections due to the Pseudomonas aeruginosa, which frequently ends in pulmonary exacerbations. With their anti-inflammatory, anti-virulence, and anti-biofilm properties, macrolides such as azithromycin (AZM) play a beneficial role in the treatment of CF.Objectives:
We evaluated the ability of biofilm formation among P. aeruginosa isolates from CF patients and compared the anti-bacterial and anti-biofilm effect of the AZM on them.Methods:
Minimum inhibitory concentration (MIC) and minimum bactericidal concentration (MBC) of AZM against 21 P. aeruginosa isolates from Iranian CF patients were determined. Then, we investigated the ability of biofilm formation by P. aeruginosa and also anti-biofilm effect of AZM on biofilm producing P. aeruginosa isolates.Results:
The results showed a high level of AZM MIC (≤ 512 µg/mL) and MBC (≤ 2048 µg/mL) against P. aeruginosa isolates. Most isolates (70%) were biofilm producers, 20% being strong producers. The AZM anti-biofilm effect showed that sub-MIC concentration (≤ 64 µg/mL) could inhibit biofilm production.Conclusions:
P. aeruginosa isolated from CF patients showed a high level of MIC and MBC for AZM that indicated a weak anti-bacterial effect of AZM. However, AZM inhibited biofilm formation in low doses; this might lead to dangerous chronic pulmonary infection by P. aeruginosa.Keywords
Cystic Fibrosis Pseudomonas aeruginosa Azithromycin Anti-Biofilm Anti-Bacterial Effect Biofilm
1. Background
Cystic fibrosis (CF) is a common multisystem autosomal recessive disease, with an unknown prevalence in Iran. However, about 1200 patients have been registered in the Iranian CF Center Registry System so far (1). Mutation of the cystic fibrosis transmembrane conductive regulator (CFTR) gene that affects chloride ion channel function creates thicker and stickier mucus in the lungs, pancreas, and other organs, resulting in cystic fibrosis. Although CF occurs in numerous organs, it is mostly presented in the upper and lower airways, pancreas, bowel, and reproductive tracts (1).
For many patients, pulmonary problems are the worst in terms of symptoms, and the required treatments (1). Meanwhile, Pseudomonas aeruginosa, an opportunistic pathogen, is the most important cause of pulmonary infection in CF patients, which mainly leads to lung destruction and the patient’s death (2). By late adolescence, 80% of CF patients have been already chronically infected (2).
Unique lung environment condition in CF patients with dehydrated mucus, high osmotic pressure, and high salt concentration, leads to alginate over-production and results in emerging mucoid phenotypes of P. aeruginosa, and ultimately its biofilm formation (2). Biofilm is defined as communities of microbes associated with surfaces or interfaces; bacteria growing in biofilm are much more resistant to antibiotics and can cause lethal chronic infections (2). Evidence suggests that biofilm formation is associated with therapeutic failure, especially in chronic infection (3, 4). Furthermore, P. aeruginosa shows resistance to a variety of antibiotics via its innate and acquired resistance mechanisms, so finding an effective antibiotic for its eradication becomes very difficult (5).
In recent years, the efficacy of macrolides in P. aeruginosa pulmonary infection in bronchiectasis, Chronic Obstructive Pulmonary Disease (COPD), and CF patients have been reported (6). Several studies have demonstrated that macrolides through many mechanisms decrease exacerbations, improve lung function, and exert their beneficial anti-inflammatory activity and anti-virulence effects (6, 7). It has been reported that long-term daily administration of azithromycin (AZM) significantly decreases symptoms and inflammatory parameters and increases the survival of CF patients suffering from chronic P. aeruginosa lung infection (8). A similar effect has been reported in CF and COPD patients with chronic P. aeruginosa infection in a controlled study using AZM 250 mg/day for 3 months and then 500 mg orally three times per week, over 12 months (9). Also, the impact of AZM on biofilm and mechanism of its effect has been considered, and it has been shown that AZM prevents and abolishes the biofilm matrix through inhibiting the quorum sensing, which is necessary for maturation of the biofilm (5, 7).
Although the role of AZM as a quorum sensing inhibitor has been already shown, several studies have reported that P. aeruginosa may be resistant to AZM by MexCD-OprJ efflux pump during biofilm formation (5).
Furthermore, heterogeneous responses to the environment within an isogenic bacterial population could lead to the appearance of phenotypic variants called persister cells. P. aeruginosa strains isolated from CF patients typically show high levels of persister cells. Interestingly, the persister cells are not genetically resistant to antibiotics but are tolerant to high concentrations of antibiotics and make the treatment of infection in CF lung even more complicated (5, 10).
2. Objectives
In the present study, we evaluated the ability of biofilm formation in P. aeruginosa isolated from CF patients that might associate with chronic lung infection and antibiotic treatment failure. In CF patients, chronic lung infection of P. aeruginosa mainly leads to lung destruction and death. Also, we evaluated and compared anti-bacterial and anti-biofilm effects of AZM on these isolates to demonstrate the efficiency mechanism. To our knowledge, up to now, there is no study on anti-biofilm effects of AZM against P. aeruginosa isolated from Iranian CF patients.
3. Methods
3.1. Clinical Isolates
Of 55 CF patients registered to CF Center in Tehran Children’s Medical Center between June and December 2018 who had a history of P. aeruginosa lung infection, 21 P. aeruginosa isolates were clinically collected. All isolates were maintained at -70°C in Tryptic soy broth (TSB) (Merck, Germany) containing 10% dimethyl sulfoxide (v/v) (Sigma, USA) before using. All ethical aspects of this project have been complied with the principles laid down in the Declaration of Helsinki.
P. aeruginosa ATCC 27853 and P. aeruginosa PAO1 were used as the quality control for antibiotic susceptibility and biofilm assay, respectively.
3.2. Anti-Bacterial Susceptibility Testing
Anti-bacterial susceptibility profiles of the P. aeruginosa isolates were determined by the disk diffusion method according to the recommendations of the Clinical and Laboratory Standards Institute (CLSI, 2018 ed) (11). The antibiotic disks (MAST Diagnostics, Merseyside, UK) were azithromycin (15 μg), ceftazidime (30 μg), ciprofloxacin (5 μg), aztreonam (30 μg), meropenem (10 μg), and tobramycin (10 μg). All tests were repeated at least 3 times, and the averages of the inhibition zones were reported.
3.3. Minimum Inhibitory Concentration Determination
The minimum inhibitory concentration (MIC) of AZM (donated by Tehran Chemie, Iran) was estimated by the broth microdilution method (11). Briefly, a final bacterial inoculum of 5 × 106 log-phase CFU/mL was used as the inoculum. AZM solution (10 mg/mL) was prepared by ethanol 95% dilutions of 4 - 2048 µg/mL in microtiter plates (SPL Plastic Labware, Korea). The concentration of antibiotic resulting in no visible growth was taken as the MIC. All tests were repeated at least 3 times, and the averages of MIC values were reported. The last two columns of each microtiter plate were used as controls (medium control and bacterial growth control).
3.4. AZM Minimum Bactericidal Concentration Determination
The minimum bactericidal concentration (MBC) of AZM was determined according to CLSI guidelines (11). Briefly, from microtiter plate wells with a concentration equal and higher than MIC, inoculated on Tryptic soy Agar (TSA). All tests were repeated at least 3 times, and the averages of MBC were reported.
3.5. Biofilm Assay
For biofilm assay, a flat-bottomed 96-well cell culture microtiter plate (SPL Plastic Labware, Korea) was used. As described previously, 100 μL of TSB medium supplemented with 0.2% glucose was added to each well and then 100 μL of 108 CFU/mL (24 hour P. aeruginosa culture) were inoculated in each well and then were incubated at 37°C for 20 hours.
After incubation, the planktonic cells were removed, and plates were rinsed thrice using pre-warmed (37°C) physiological saline. The plates were dried for 15 min in a sterile setting. Then, as supplementary 200 μL of TSB with 0.2% glucose and 50 μL of 2,3,5-triphenyl-tetrazolium chloride (TTC) solutions (0.5%) (Merck, Germany) were added to each well, plates were incubated in the dark for 6 hours at 37°C and 150 rpm. After this period, the absorbance was measured at 405 nm. The assay was performed in 6 replicates in 2 different days for each experiment (12).
3.6. AZM Minimal Biofilm Inhibitory Concentration Determination
The minimal biofilm inhibitory concentration (MBIC) was determined by microtiter plate and according to CLSI guidelines (11). Briefly, after filling the wells with 100 μL of TSB with 0.2% glucose, a sub-MIC of AZM was used for serial dilutions (1 - 512 µg/mL). The last two columns of each microtiter plate were used as controls (medium control and bacterial growth control). By using a 24-hour culture of biofilm-producing P. aeruginosa isolates, 100 μL of suspension with 0.5 McFarland standard concentration was inoculated in each well, except medium controls, and then were incubated at 37°C for 20 hours.
Similar to the method described above, removing planktonic cells, washing, and drying were carried out, and then 170 μL of TSB with 0.2% glucose and 30 μL of 1%TTC solution were added to each well. Plates were incubated in dark for 5 hours at 37°C and 150 rpm. After this period the absorbance was measured at 490 nm. The assay was performed in 3 replicates in 2 different days for each experiment (12).
4. Results
4.1. Clinical Isolates
Among 21 P. aeruginosa clinical isolates, 42.54% were collected from male patients and 57.4% from female ones. Most of the isolates (90%) were obtained by throat swab, and the remaining by sputum culture.
4.2. Anti-Bacterial Susceptibility
The antibiotic susceptibility results showed a low rate of antibiotic resistance and no MDR isolates among P. aeruginosa isolates. Results showed only 20% resistance to aztreonam, 15% to tobramycin, and 5% to ciprofloxacin.
The majority of the isolates (75%) showed heterogeneous azithromycin resistance. Heterogeneous macrolides resistance is defined as the isolates with colonies in the inhibition zone of erythromycin, azithromycin, and clarithromycin disks.
4.3. AZM MIC Determination
Figure 1 shows Distribution of MIC (µg/mL) in the 21 P. aeruginosa isolates. All strains showed high resistance to AZM expressing in high-level of MIC (64 ≤ MIC ≤ 512 µg/mL), indicating undesirable anti-bacterial effect.
Distribution of MIC (µg/mL) in P. aeruginosa isolates from CF patients
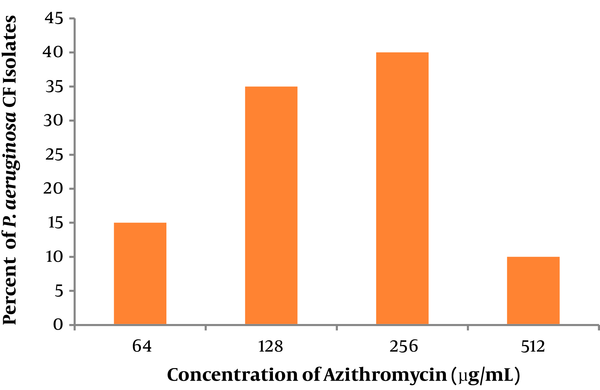
4.4. AZM MBC Determination
All strains showed high resistance to AZM expressed in high-level of MBC (512 ≤ MIC ≤ 2048 µg/mL), indicating undesirable bactericidal effect. Figure 2 shows the comparison of MIC (µg/ml) and MBC (µg/ml) among P. aeruginosa isolates from CF patients.
Comparison of MIC (µg/mL) and MBC (µg/mL) among P. aeruginosa isolates from CF patients
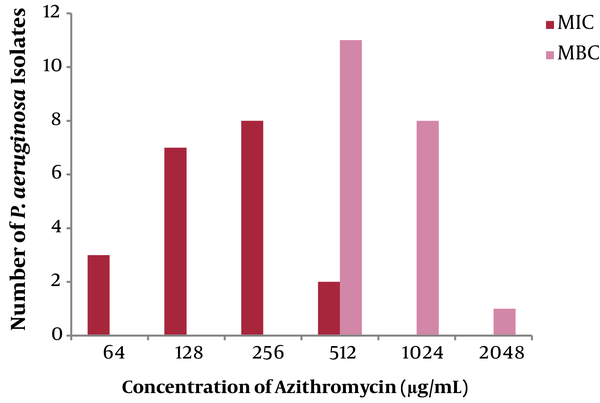
4.5. Biofilm Assay
Results showed that 70% of P. aeruginosa isolates were biofilm producers (20% were strong-biofilm producers and 50% moderate biofilm-forming isolates), and 30% of them were non-biofilm producers. On the other hand, most of the isolates produced biofilm that correlated with chronic infection.
4.6. AZM MBIC Determination
Results showed that for biofilm-producing P. aeruginosa isolates, sub-MIC concentration of AZM (8 ≤ MBIC ≤ 64 µg/mL) inhibited the biofilm formation. It indicates a robust anti-biofilm effect of AZM in a low dose.
Figure 3 shows comparison of MIC (µg/mL) and MBIC (µg/mL) among biofilm producing P. aeruginosa isolates from CF patients.
Comparison of MIC (µg/mL) and MBIC (µg/mL) among biofilm producing P. aeruginosa isolates from CF patients

5. Discussion
Cystic fibrosis is a genetic, systemic, and life-shortening disease characterized by various organ dysfunctions, mostly progressive lung disease and pancreatic insufficiency (1). P. aeruginosa is the main bacteria associated with pulmonary disease in patients with CF. P. aeruginosa in biofilm is much more resistant to antibiotics and host immune system compared to planktonic cells that is difficult to eradicate bacteria resulting in respiratory failure, and finally, death (13-15). We observed that 70% of P. aeruginosa isolates produce biofilm, and 20% were strong biofilm producers. Recently, other studies in Iran have reported similar results (16, 17). Emaneini et al. showed 83.5% of P. aeruginosa isolates were biofilm producing strain amongst which 25.88% were determined as strong biofilm producers (16). Pournajaf et al. reported that 78.3% of all isolates were able to biofilm formation and of which, 57.1% were strong biofilm producers (17). The difference in rate of strong biofilm producers may be related to the type of acute or chronic infection of CF patients or time of infection. In our study, P. aeruginosa was obtained from CF patients who had a history of P. aeruginosa lung infection.
The results showed low antibiotic resistance among P. aeruginosa isolates as well as no MDR isolates. Our anti-bacterial susceptibility testing showed only 20% resistance to aztreonam, 15% to tobramycin, and 5% to ciprofloxacin. But other studies conducted in Iran, showed different antibiotic resistant patterns (16, 17). Emaneini et al. reported that less than half of P. aeruginosa isolates were determined as MDR but Pournajaf et al. reported low rates of MDR (16, 17). This difference in antibiotic resistance pattern may be caused by the different common strains in any region and antibiotic treatment regimens.
Our findings show a high level of AZM MIC for P. aeruginosa CF isolates within the range of 64 and 512 μg/mL, and 128 μg/mL for P. aeruginosa PAO1 that are very similar to other studies, indicating the undesirable anti-bacterial effect of AZM (7-19). In 2012, Lutz and et al. reported high level of AZM MIC against P. aeruginosa CF isolates (between 32 to 4096 mg/L) (19). Another study, demonstrated a high level of MIC (> 256 mg/L) in standard growth media (20). Also, we observed weak bactericidal effect of AZM for P. aeruginosa CF isolates. The MBC for P. aeruginosa CF isolates was within the range of 512 ≤ MIC ≤ 2048 µg/mL. This high rate of resistance can be due to multidrug-efflux pumps that efficiently remove macrolides from the cell, thus this bacterium being considered intrinsically resistant to macrolides such as AZM (19).
In the meantime, we observed that 75% of P. aeruginosa isolates showed heterogeneous azithromycin resistance phenotype, i.e., subpopulations of bacteria exhibit heterogeneous susceptibilities to a particular antibiotic within an isogenic population. The heterogeneity of bacterial cells might be the reason for the increasing resistance to antibiotics, and mostly, it occurs in response to high doses of antibiotics (5, 10, 21).
We studied anti-biofilm effect of the AZM on P. aeruginosa isolates from Iranian CF patients for the first time. Our results demonstrated that sub-MIC of AZM could inhibit production of biofilm among P. aeruginosa isolates. The MBIC results showed that for biofilm producer P. aeruginosa isolates, sub-MIC concentration of AZM (8 ≤ MBIC ≤ 64 µg/mL) inhibits biofilm formation. Previous studies support these results. They reported that sub-MIC of AZM has many pleiotropic effects on P. aeruginosa, including virulence inhibition, killing of stationary-phase and/or biofilm-forming cells, and synergism with other antimicrobials and serum complement (7, 19, 22). Moreover, sub-MIC of AZM prevents biofilm production in P. aeruginosa isolates from urinary tract infection (18, 23). Also, the anti-biofilm effect of AZM has been shown on other bacteria like Haemophilus influenzae, and not just P. aeruginosa (24).
Although AZM has not been approved for the treatment of infections caused by P. aeruginosa and no published breakpoints exist for this species, various studies have shown beneficial effect in patients with CF, COPD, and asthma (11, 25-27). In addition, the Cystic fibrosis foundation guideline and the American Pulmonary Clinical Practice Guidelines currently advocate routine use of AZM for all CF patients aged above 6 years irrespective of chronic P. aeruginosa infection (28). The 2018 revision of best practice guidelines of the European CF Society states that maintenance therapy with AZM can improve lung function and reduce pulmonary exacerbations in P. aeruginosa chronically infected patients (29).
The main limitation of this study was expensive materials and equipments and also there were a few participants in sampling. Due to the small number of volunteer patients for sampling, there was no difference between those who were in the acute or chronic infection stage. Also, among the patients participating in the sampling, some had a history of AZM use that could affect the test results. In addition, in P. aeruginosa isolates from CF patients, in the laboratory the antibiotic resistance might be different from that in vivo.
5.1. Conclusion
The high rate of biofilm in P. aeruginosa isolated from CF patients might be so disturbing. It seems the biofilm formation associated with antibiotic resistance and treatment failure; these two occurrences might lead to dangerous chronic infection. So, it appears that the prevention of biofilm production could reduce chronic infection. Furthermore P. aeruginosa pulmonary infection treatment might be achieved by using proper anti-biofilm or beneficial therapies on microbial biofilm as well as appropriate antibiotics regimen. AZM showed a high level of MIC and MBC and a weak anti-bacterial effect on P. aeruginosa isolated from CF patients.
Acknowledgements
References
-
1.
O'Sullivan BP, Freedman SD. Cystic fibrosis. The Lancet. 2009;373(9678):1891-904. https://doi.org/10.1016/s0140-6736(09)60327-5.
-
2.
Høiby N. Understanding bacterial biofilms in patients with cystic fibrosis: current and innovative approaches to potential therapies. Journal of Cystic Fibrosis. 2002;1(4):249-54. https://doi.org/10.1016/s1569-1993(02)00104-2.
-
3.
Perez LR, Costa MC, Freitas AL, Barth AL. Evaluation of biofilm production by Pseudomonas Aeruginosa isolates recovered from cystic fibrosis and non-cystic fibrosis patients. Braz J Microbiol. 2011;42(2):476-9. [PubMed ID: 24031658]. [PubMed Central ID: PMC3769813]. https://doi.org/10.1590/S1517-838220110002000011.
-
4.
Ciofu O, Rojo-Molinero E, Macia MD, Oliver A. Antibiotic treatment of biofilm infections. APMIS. 2017;125(4):304-19. [PubMed ID: 28407419]. https://doi.org/10.1111/apm.12673.
-
5.
Pang Z, Raudonis R, Glick BR, Lin TJ, Cheng Z. Antibiotic resistance in Pseudomonas aeruginosa: mechanisms and alternative therapeutic strategies. Biotechnol Adv. 2019;37(1):177-92. [PubMed ID: 30500353]. https://doi.org/10.1016/j.biotechadv.2018.11.013.
-
6.
Fan LC, Lin JL, Yang JW, Mao B, Lu HW, Ge BX, et al. Macrolides protect against Pseudomonas aeruginosa infection via inhibition of inflammasomes. Am J Physiol Lung Cell Mol Physiol. 2017;313(4):L677-86. [PubMed ID: 28684545]. https://doi.org/10.1152/ajplung.00123.2017.
-
7.
Imperi F, Leoni L, Visca P. Antivirulence activity of azithromycin in Pseudomonas aeruginosa. Front Microbiol. 2014;5:178. [PubMed ID: 24795709]. [PubMed Central ID: PMC4001013]. https://doi.org/10.3389/fmicb.2014.00178.
-
8.
Clement A, Tamalet A, Leroux E, Ravilly S, Fauroux B, Jais JP. Long term effects of azithromycin in patients with cystic fibrosis: A double blind, placebo controlled trial. Thorax. 2006;61(10):895-902. [PubMed ID: 16809416]. [PubMed Central ID: PMC2104771]. https://doi.org/10.1136/thx.2005.057950.
-
9.
Pomares X, Monton C, Espasa M, Casabon J, Monso E, Gallego M. Long-term azithromycin therapy in patients with severe COPD and repeated exacerbations. Int J Chron Obstruct Pulmon Dis. 2011;6:449-56. [PubMed ID: 22003290]. [PubMed Central ID: PMC3186743]. https://doi.org/10.2147/COPD.S23655.
-
10.
Rojo-Molinero E, Macia MD, Oliver A. Social Behavior of Antibiotic Resistant Mutants Within Pseudomonas aeruginosa Biofilm Communities. Front Microbiol. 2019;10:570. [PubMed ID: 30967851]. [PubMed Central ID: PMC6438888]. https://doi.org/10.3389/fmicb.2019.00570.
-
11.
Clinical and Laboratory Standards Institute (CLSI). M100, 27th ed. 2018.
-
12.
Sabaeifard P, Abdi-Ali A, Soudi MR, Dinarvand R. Optimization of tetrazolium salt assay for Pseudomonas aeruginosa biofilm using microtiter plate method. J Microbiol Methods. 2014;105:134-40. [PubMed ID: 25086178]. https://doi.org/10.1016/j.mimet.2014.07.024.
-
13.
Hoiby N, Bjarnsholt T, Moser C, Jensen PO, Kolpen M, Qvist T, et al. Diagnosis of biofilm infections in cystic fibrosis patients. APMIS. 2017;125(4):339-43. [PubMed ID: 28407432]. https://doi.org/10.1111/apm.12689.
-
14.
Layeghifard M, Li H, Wang PW, Donaldson SL, Coburn B, Clark ST, et al. Microbiome networks and change-point analysis reveal key community changes associated with cystic fibrosis pulmonary exacerbations. NPJ Biofilms Microbiomes. 2019;5:4. [PubMed ID: 30675371]. [PubMed Central ID: PMC6341074]. https://doi.org/10.1038/s41522-018-0077-y.
-
15.
Zolin A, Bossi A, Cirilli N, Kashirskaya N, Padoan R. Cystic Fibrosis Mortality in Childhood. Data from European Cystic Fibrosis Society Patient Registry. Int J Environ Res Public Health. 2018;15(9). [PubMed ID: 30223583]. [PubMed Central ID: PMC6163251]. https://doi.org/10.3390/ijerph15092020.
-
16.
Emaneini M, Kalantar-Neyestanaki D, Jabalameli L, Hashemi M, Beigverdi R, Jabalameli F. Molecular analysis and antimicrobial resistance pattern of distinct strains of Pseudomonas aeruginosa isolated from cystic fibrosis patients in Iran. Iranian Journal of Microbiology. 2019;11(2):89-107. https://doi.org/10.18502/ijm.v11i2.1068.
-
17.
Pournajaf A, Razavi S, Irajian G, Ardebili A, Erfani Y, Solgi S, et al. Integron types, antimicrobial resistance genes, virulence gene profile, alginate production and biofilm formation in Iranian cystic fibrosis Pseudomonas aeruginosa isolates. Infez Med. 2018;26(3):226-36. [PubMed ID: 30246765].
-
18.
Bala A, Kumar R, Harjai K. Inhibition of quorum sensing in Pseudomonas aeruginosa by azithromycin and its effectiveness in urinary tract infections. J Med Microbiol. 2011;60(Pt 3):300-6. [PubMed ID: 21127154]. https://doi.org/10.1099/jmm.0.025387-0.
-
19.
Lutz L, Pereira DC, Paiva RM, Zavascki AP, Barth AL. Macrolides decrease the minimal inhibitory concentration of anti-pseudomonal agents against Pseudomonas aeruginosa from cystic fibrosis patients in biofilm. BMC Microbiol. 2012;12:196. [PubMed ID: 22958421]. [PubMed Central ID: PMC3485169]. https://doi.org/10.1186/1471-2180-12-196.
-
20.
Buyck JM, Plesiat P, Traore H, Vanderbist F, Tulkens PM, Van Bambeke F. Increased susceptibility of Pseudomonas aeruginosa to macrolides and ketolides in eukaryotic cell culture media and biological fluids due to decreased expression of oprM and increased outer-membrane permeability. Clin Infect Dis. 2012;55(4):534-42. [PubMed ID: 22573850]. https://doi.org/10.1093/cid/cis473.
-
21.
Qin X, Zhou C, Zerr DM, Adler A, Addetia A, Yuan S, et al. Heterogeneous Antimicrobial Susceptibility Characteristics in Pseudomonas aeruginosa Isolates from Cystic Fibrosis Patients. mSphere. 2018;3(2). [PubMed ID: 29564400]. [PubMed Central ID: PMC5853491]. https://doi.org/10.1128/mSphere.00615-17.
-
22.
Wang X, Cai Y, Xing H, Wu W, Wang G, Li L, et al. Increased therapeutic efficacy of combination of azithromycin and ceftazidime on Pseudomonas aeruginosa biofilm in an animal model of ureteral stent infection. BMC Microbiol. 2016;16(1):124. [PubMed ID: 27341798]. [PubMed Central ID: PMC4921005]. https://doi.org/10.1186/s12866-016-0744-1.
-
23.
Xu ZG, Gao Y, He JG, Xu WF, Jiang M, Jin HS. Effects of azithromycin on Pseudomonas aeruginosa isolates from catheter-associated urinary tract infection. Exp Ther Med. 2015;9(2):569-72. [PubMed ID: 25574236]. [PubMed Central ID: PMC4280936]. https://doi.org/10.3892/etm.2014.2120.
-
24.
Starner TD, Shrout JD, Parsek MR, Appelbaum PC, Kim G. Subinhibitory concentrations of azithromycin decrease nontypeable Haemophilus influenzae biofilm formation and Diminish established biofilms. Antimicrob Agents Chemother. 2008;52(1):137-45. [PubMed ID: 17954687]. [PubMed Central ID: PMC2223912]. https://doi.org/10.1128/AAC.00607-07.
-
25.
Brusselle GG, Vanderstichele C, Jordens P, Deman R, Slabbynck H, Ringoet V, et al. Azithromycin for prevention of exacerbations in severe asthma (AZISAST): a multicentre randomised double-blind placebo-controlled trial. Thorax. 2013;68(4):322-9. [PubMed ID: 23291349]. https://doi.org/10.1136/thoraxjnl-2012-202698.
-
26.
Nichols DP, Happoldt CL, Bratcher PE, Caceres SM, Chmiel JF, Malcolm KC, et al. Impact of azithromycin on the clinical and antimicrobial effectiveness of tobramycin in the treatment of cystic fibrosis. J Cyst Fibros. 2017;16(3):358-66. [PubMed ID: 28025037]. [PubMed Central ID: PMC5492972]. https://doi.org/10.1016/j.jcf.2016.12.003.
-
27.
Maneshi A, Modaresi MR, Khalili H, Shojae L, Faghihi T. Nebulized Azithromycin Versus Oral Azithromycin as Anti-Inflammatory Therapy in Children with Cystic Fibrosis: A Prospective Randomized Open-Label Trial. Iranian Journal of Pediatrics. 2019;In Press(In Press). https://doi.org/10.5812/ijp.96977.
-
28.
Mogayzel PJ, Naureckas ET, Robinson KA, Mueller G, Hadjiliadis D, Hoag JB, et al. Cystic fibrosis pulmonary guidelines. Chronic medications for maintenance of lung health. Am J Respir Crit Care Med. 2013;187(7):680-9. [PubMed ID: 23540878]. https://doi.org/10.1164/rccm.201207-1160oe.
-
29.
Castellani C, Duff AJA, Bell SC, Heijerman HGM, Munck A, Ratjen F, et al. ECFS best practice guidelines: the 2018 revision. J Cyst Fibros. 2018;17(2):153-78. [PubMed ID: 29506920]. https://doi.org/10.1016/j.jcf.2018.02.006.