Abstract
Background:
Obstructive sleep apnea-hypopnea syndrome (OSAHS) can impact multiple organ systems in children.Objectives:
To investigate the effects of OSAHS on T-lymphocyte subgroups and natural killer (NK) cell activity in the peripheral blood of affected children.Methods:
A total of 85 children with OSAHS were enrolled into an experimental group (OSAHS) and 76 healthy children were placed in a control group (CON) to compare peripheral blood levels of CD3+, CD4+, CD8+, and NK cell activity using flow cytometry. Meanwhile, their polysomnography results were monitored to analyze the correlation between T-lymphocyte subgroups/NK cell activity and lowest pulse oxygen saturation (LSaO2)/apnea-hypopnea index (AHI).Results:
Compared to the CON group, the CD3+ percentage in the OSAHS group showed no significant difference (65.98 ± 6.54 vs 64.36 ± 5.32; P > 0.05), but the CD4+ percentage, CD4+/CD8+ ratio, and NK cell activity decreased markedly (33.52 ± 3.04 vs 35.26 ± 3.68,1.29 ± 0.14 vs 1.43 ± 0.26, and 11.47 ± 4.58 vs 12.69 ± 2.36, respectively; P < 0.05). In addition, the CD8+ percentage increased significantly (26.18 ± 4.76 vs 24.36 ± 2.32; P < 0.05). Linear regression analysis indicated that the CD3+ percentage was not related to LSaO2/AHI (P > 0.05), but the CD4+ and CD8+ percentages, CD4+/CD8+ ratio, and NK cell activity were linearly related to LSaO2 and AHI (P < 0.05).Conclusions:
OSAHS can affect the cellular immunity of children. The AHI and LSaO2 may be involved in cellular immunity function.Keywords
1. Background
The third edition of the International Classification of Sleep Disorders separates sleep-related apnea into obstructive sleep apnea (OSA) syndrome, central sleep apnea syndrome, sleep-related hypoxia, and sleep-related hypoxemia (1). Obstructive sleep apnea-hypopnea syndrome (OSAHS) tends to impact preschool children (2), possibly because children at this age are in the productive period of tonsil and adenoid hyperplasia, which occupies a significant amount of space in the upper respiratory tract (3). Children in this developmental stage may restore their physical strength via good sleep, thus promoting intelligence and growth. Children with OSAHS may repeatedly suffer from hypoxia and hypercapnia due to frequent apnea and hypopnea during sleep, resulting in potential damage to multiple organsystems as well as the immune function of the body. Adenoid and tonsil hypertrophy is the main cause of OSAHS in children. Thus, removal of the adenoids and tonsils is an effective treatment method for children with OSAHS. However, adenoids and tonsils play important roles in childhood immune defense, so the pros and cons of actively treating OSAHS has become the focus of many researchers and parents (4-8).
T-lymphocytes are a large cell population with important regulatory functions in the body’s immune system. The total and subpopulation numbers are important indicators for the evaluation of cellular immune function (9). Each T-lymphocyte subset interacts to maintain the balance of immune function. At present, it is believed that one of the more reliable methods for understanding the cellular immune status of the body is to analyze T-lymphocyte subset changes in peripheral blood. The most common method for classifying T-lymphocyte subsets is to separate them into different functional subpopulations based on cell surface markers using monoclonal antibodies. Mature T-lymphocytes express CD3+ molecules on the surface, and mature T-lymphocytes are divided into two subgroups (CD4+ T cells and CD8+ T cells) according to their surface markers. CD4+ T cells are T helper cells, and CD8+ T cells are T suppressor cells. These two cell types interact to maintain the stability of the body’s immune function (10). Monitoring of T helper cells and T suppressor cells can determine whether the functional status of the human immune system is normal.
Natural killer (NK) cells play immunological surveillance and regulation roles in the body, providing a natural defense against pathogens and serving as an important evaluation index for non-specific immunity (11). Detecting T-lymphocyte subsets and NK cell activity can also help to clarify the status of the body’s immune system function.
Although some scholars (12) have found that OSAHS exerts certain impacts on the immune function of children, the evidence remains inconclusive. Many researchers believe that these patients may have varying degrees of immune decline. To investigate the effects of OSAHS on the immune function of children, we detected the respective percentages of T-lymphocyte subsets (CD3+, CD4+, and CD8+) and NK cell activity in the peripheral blood of 85 children with OSAHS admitted to our department from December 2015 to December 2018. The relationship between immune function and the course/severity of the disease was analyzed in order to objectively evaluate the humoral immune function of children with OSAHS and provide a reference for whether the clinical treatment of this disease is active.
2. Methods
2.1. Subjects
OSAHS group: From December 2015 to December 2018, a total of 85 children with OSAHS were hospitalized in our department for snoring during night time sleep and open mouth breathing, including 57 boys and 28 girls aged 3 – 10 years with a mean age of 5.7 years. The children were divided into three subgroups according to the severity of the syndrome, namely a mild syndrome subgroup (MID, 33 cases), a moderate syndrome subgroup (MOD, 27 cases), and a severe syndrome subgroup (SEV, 25 cases). Moreover, the children were also separated into a < 3 years subgroup (< 3, 53 cases) and a ≥ 3 years subgroup (≥ 3, 32 cases) according to the duration of the disease.
2.2. Inclusion Criteria
1) All children were monitored by overnight polysomnography (PSG). An obstructive apnea index (OAI) > 1 time/h or apnea hypopnea index (AHI) > 5 times/h was defined as ‘abnormal’. Minimum arterial oxygen saturation (LSaO2) of less than 92% was defined as ‘hypoxemia’. Any child who met the above two criteria was diagnosed with OSAHS. Classification of OSAHS was performed as follows: MID (AHI 5 - 10 times/h or OAI 1 - 5 times/h, LSaO2 85 - 91%), MOD (10 times/h < HI ≤ 20 times/h or 5 times/h <OAI ≤ 10 times/h, LSaO2 75 - 84%), and SEV (AHI > 20 times/h or OAI > 10 times/h, LSaO2 < 75%).
2) All children with OSAHS underwent lateral nasopharyngeal radiography or electronic nasopharyngoscopy, which suggested that the bilateral tonsils were in grade II to III. The adenoids were hypertrophied and blocked more than half of the nostrils, so OSAHS was determined to be caused by adenoid hyperplasia and/or tonsil hypertrophy. Among them, 14 cases were of simple tonsil hypertrophy, 10 cases were of simple adenoid hypertrophy, and 61 cases were of tonsil-adenoid hypertrophy.
3) There were no abnormalities in the birth, feeding, and growth histories of any children diagnosed with OSAHS.
2.3. Exclusion Criteria
1) A history of recent or repeated upper respiratory tract infections.
2) Abnormalities in the congenital anatomy, such as th emouth, nose, or pharynx, that affect ventilation.
3) A history of allergic rhinitis, asthma, or other diseases that affect immune function.
4) A history of chronic diseases in important organs, such as the heart, brain, or kidneys.
CON group: At the same time, a total of 76 healthy children without related or systemic diseases were enrolled in our hospital, including 54 boys and 22 girls aged 3 – 10 years with an average age of 6.8 years. The selected cases had no history of cold four weeks prior to the study; no history of allergic rhinitis, asthma, or other diseases that affect immune function; and no history of nasal polyps, adenoids, tonsil hypertrophy, obesity, or other systemic diseases. General examinations of the two groups (thorax X-ray, electrocardiogram, coagulation function, blood routine, and complete biochemical analysis) were in the normal ranges. The general data, such as age, sex, and body mass index between the two groups, are shown in Table 1.
Comparison of General Data Between Group OSAHS and Group CON
Group | Age (y) | Gender (n) | BMI (kg/m2) | |
---|---|---|---|---|
Boy | Girl | |||
OSAHS | 6.59 ± 1.56 | 57 | 28 | 16.34 ± 2.35 |
CON | 7.04 ± 2.48 | 54 | 22 | 17.02 ± 4.02 |
P a | 0.183 | 0.732 | 0.131 |
This study was conducted in accordance with the Declaration of Helsinki andapproved by the Ethics Committee of Fujian Medical University. Written informed consent was obtained from all participants’ guardians.
2.4. Instruments and Reagents
Flow cytometer C 500 Beckman. Lymphocyte subset detection kit CYTO-STAT tetraCHROME Beckman. Lysing Solution OptiLyse C Beckman.
2.5. Sleep Monitoring
A multi-channel sleep monitor produced by ResMed (ApneaLinkTMPlus; San Diego, CA, USA) was used for at least 7h of monitoring from going to sleep at night to awakening the next morning. The monitoring items included nose and mouth airflow, chest and abdomen movement, and oxygen saturation (SpO2). The AHI, LSpO2, and mean blood oxygen saturation (MSpO2) were calculated based on the monitoring results.
2.6. Collection and Determination of Specimens
Specimen collection: In the OSAHS group, 2 mL of venous blood was collected by an assigned nurse on the second day after admission and injected into a heparin sodium-covered anticoagulation tube. Blood from the CON group was collected by an outpatient nurse when fasting on the day of the physical examination. The blood collection time for both groups was 7:00 - 8:00 in the morning. The collected blood samples were tested for lymphocyte subsets by the same flow cytometer in our laboratory.
Working principle: Antibodies were selected from the Beckman Coulter Cytolymphocyte Subgroup Reagent CYTO-STAT CD45-FITC/CD4-RD1/ CD8-ECD/CD3-PC5 (LOT 7536243f) and CD45-FITC/CD56-RD1/CD19-ECD/ CD3-PC5(LOT7580190F). Different antibodies were labeled with fluorescein. When the fluorescent antibody specifically bound to the antigen molecule on the cell surface through the antigen-antibody reaction, the cells were stained with fluorescein. The flow cytometer focuses the shaped laser beam to irradiate the high-speed flow cells that have been stained and measures the intensity of scattered light and fluorescence generated on the stained cells for analyzing the expression of the corresponding protein on the cell surface to achieve rapid quantitative determination of the cells.
Experimental procedure: A flow cytometer tube was filled with 100 µL of anticoagulated blood and 10 µL of the corresponding antibody, vortexed, and left to stand in darkness at room temperature for 20 min. OptiLyse C was then added to the tube, vortexed, and left to stand at room temperature for 10 min to lyse the red blood cells. Next, 500 µL of phosphate-buffered saline (PBS) was added to the tube, equilibrated at room temperature for 5 min, and centrifuged for 5 min (2000 rpm/min). The supernatant was discarded, resuspended in 500 µL of PBS, thoroughly vortexed and mixed, and detected by flow cytometry.
2.7. Statistical Analysis
SPSS Statistics 17.0 software (IBM SPSS, Armonk, NY, USA) was used for data analysis. The measurement data meeting the normal distribution were expressed as means ± standard deviations (x ± s). The t-test was used for comparisons between the two independent sample groups, and the Student-Newman-Keuls test was used for pairwise comparisons between the two groups. The chi-square test was used to compare the count data between the two groups with P < 0.05 considered as statistically significant. Pearson linear correlation analysis was used for two variables with relevant trends with α = 0.05 (bilateral) as the test level and P < 0.05 considered as statistically significant.
3. Results
3.1. Comparison of CD3+, CD4+, CD8+, and CD4+/CD8+ Percentages as Well as NK Cell Activity
Compared to the CON group, there was no significant difference in the CD3+ percentage in the peripheral blood of the OSAHS group (P > 0.05). However, the CD4+ percentage, CD4+/CD8+ ratio, and NK cell activity significantly decreased while the CD8+ percentage in the peripheral blood significantly increased (P < 0.05; Table 2, Figures 1, and 2).
Comparison of T-Lymphocyte Subsets and NK Cell Activity in Peripheral Blood of Two Groups (Mean ± SD, %)
Analysis of T-lymphocyte subsets and NK cell activity in peripheral blood of group CON (healthy children without related diseases and systemic diseases). A: CD45-FITC, B: CD3+%: 69.5%, C: CD3+CD4+%: 26.5%, D: CD3+CD8+%: 33.7%, E: CD4/CD8 Ratio:0.79, F: CD56+NK: 9.7%. The test results of children with OSAHS suggest that the CD4/ CD8 ratio is obviously inverted.
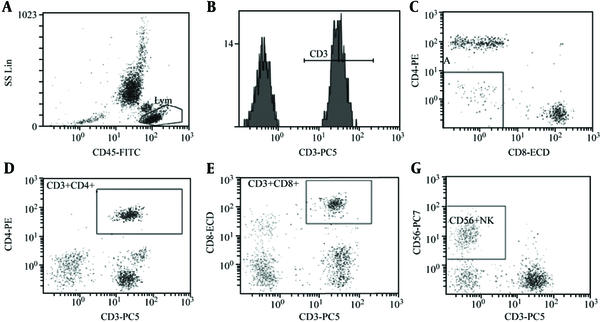
Analysis of T-lymphocyte subsets and NK cell activity in peripheral blood of group OSAHS (children with OSAHS). A: CD45-FITC, B: CD3+%: 56.7%, C: CD3+CD4+%: 34.9%, D: CD3+CD8+%:16.6%, E: CD4/CD8 Ratio: 2.1, F: CD56+NK:11.0%.
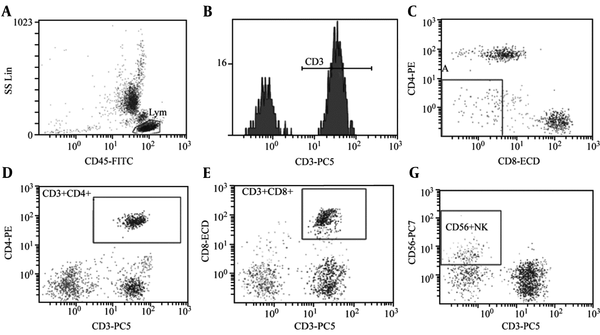
3.2. Comparison of CD3+, CD4+, CD8+, and CD4+/CD8+ Percentages as Well as NK Cell Activity in Different OSAHS Subgroups
There were no significant differences in the peripheral blood CD3+ percentage and NK cell activity among the different subgroups of the OSAHS group (P > 0.05). The CD4+ percentage and CD4+/CD8+ratio in the peripheral blood of the MID group were significantly higher than those of the MOD and SEV groups (P < 0.05). The CD4+ percentage and CD4+/CD8+ ratio in the peripheral blood between the MOD and SEV groups showed no statistically significant differences (P > 0.05). The CD8+ percentage in the peripheral blood of the SEV group was significantly higher than that of the MID group (P < 0.05). The CD8+ percentage in the peripheral blood of the MOD group was slightly higher than that of the MID group, but the difference was not statistically significant (P> 0.05; Table 3).
Comparison of T-Lymphocyte Subsets and NK Cell Activity in Peripheral Blood Among Different Subgroups of OSAHS Group (Mean ± SD, %)
3.3. Comparison of CD3+, CD4+, CD8+, and CD4+/CD8+ Percentages as Well as NK Cell Activity Between Subgroups < 3 And ≥ 3
There were no significant differences in the CD3+, CD4+, CD8+, and CD4+/CD8+ percentages as well as NK cell activity between subgroup < 3 and subgroup ≥ 3 (P > 0.05; Table 4).
Comparison of T-Lymphocyte Subsets and NK Cell Activity in Peripheral Blood Between Subgroup < 3 and Subgroup ≥ 3 (Mean ± SD, %)
Subgroup (y) | CD3+ | CD4+ | CD8+ | CD4+/CD8+ | NK |
---|---|---|---|---|---|
< 3 | 68.02 ± 7.43 | 28.34 ± 7.14 | 30.76 ± 6.37 | 0.92 ± 0.52 | 11.07 ± 4.54 |
≥ 3 | 66.76 ± 6.05 | 26.02 ± 5.32 | 32.93 ± 5.53 | 0.75 ± 0.29 | 9.72 ± 5.26 |
P a | 0.648 | 0.731 | 0.136 | 0.113 | 0.222 |
3.4. Correlations among the CD3+, CD4+, CD8+, and CD4+/CD8+ Percentages as Well as NK Cell Activity with AHI/LSaO2 in The OSAHS Group
There was no correlation between the CD3+ percentage in the peripheral blood and AHI in the OSAHS group (P > 0.05). However, there were significant linear correlations in CD4+, CD8+, CD4+/CD8+, and NK cell activity with AHI in the OSAHS group (P < 0.05), among which CD4+, CD4+/CD8+, and NK cell activity were negatively correlated with AHI while CD8+ was positively correlated with AHI (Table 5). There was no correlation between CD3+ in the peripheral blood and LSaO2 in the OSAHS group (P > 0.05). The CD4+, CD8+, and CD4+/CD8+ percentages as well as NK cell activity were significantly correlated with LSaO2 (P < 0.05), among which CD4+, CD4+/CD8+, and NK cell activity were positively correlated with LSaO2 while the CD8+ percentage was negatively correlated with LSaO2 (Table 5).
Correlation Of T-Lymphocyte Subsets and NK Cell Activity in Peripheral Blood with AHI and Lsao2 in Group OSAHS (r)
4. Discussion
OSAHS is a common and easily overlooked sleep-disordered breathing disease. Tonsils and adenoids have important immune defense functions in childhood, though the main method of treating OSAHS in children is to remove their tonsils and adenoids. Childhood is a critical period of growth and development. OSAHS in children can affect multiple organs and systems, especially the immune system, these children often suffer from slow ENT diseases such as rhinosinusitis, conductive deafness, speech disorders, etc. (13), which has attracted significant attention in recent years.
In the case of good health, the proportions of lymphocyte subgroups are in a relatively stable range. Once the value is not in this stable range, the body's immunity will be destroyed. At present, there is no uniform reference value range for peripheral blood lymphocyte subgroups, and studies have shown that normal situation CD4+/CD8+ > 1, the younger the age, CD4+/CD8+ is bigger. CD4+/CD8+ < 1 indicates that the T-lymphocyte subsets are abnormal (14).
Some scholars have found that the immune function of children with OSAHS may be damaged to varying degrees, especially the white blood cell system being involved in humoral and cellular immunity (15). In this study, the CD4+ percentage in peripheral blood and CD4+/CD8+ ratio in children with OSAHS were significantly lower than those in normal children, indicating that the cellular immune function of children with OSAHS has been damaged to some extent, consistent with the conclusions of Dyugovskaya (16). In addition, this study grouped the children with OSAHS into different groups according to the severity of the disease to compare the status of T-lymphocyte subsets and found that as the disease worsened, the effects of OSAHS on T-lymphocyte subsets in the peripheral blood of such children were more obvious, and their immune function was more damaged.
The causes of OSAHS influence on cellular immune function may be as follows: 1) Children suffer from hypoxemia caused by repeated sleep apnea at night, which may further affect the immune function by affecting neuroendocrine system activity (17). 2) Children with chronic hypoxia and hypercapnia suffer from chronic damage to various systems and organs, resulting in low immune function. 3) Children with long-term open-mouth breathing during night time sleep may cause cold dry air to directly enter the respiratory tract, increasing the likelihood of bacterial or viral infection and resulting in low immune function. In addition, tonsils and adenoids often cause immune responses due to antigen stimulation, thus resulting in lymphoid tissue hyperplasia, further aggravating OSAHS symptoms and creating a vicious circle. However, the exact mechanism underlying how OSAHS affects immune function remains unclear.
Regarding the effect of OSAHS on non-specific immunity in children, the results of this study showed that NK cell activity in children with OSAHS was significantly lower than that in normal children, suggesting that OSAHS may cause a decline in non-specific immune function. The reasons may be as follows: 1) The number and activity of NK cells in children with OSAHS are decreased due to a night time sleep structural disorder that can be gradually reversed with sleep recovery, suggesting that this immune change is related to sleep (18). 2) The surface of NK cells can express receptors corresponding to both inhibitory and activating functions. The inhibitory receptors mainly include CD94/NKG2A and killing immunoglobulin-like receptors (2DL and 3DLl), and the activating receptors primarily comprise NKG2D and natural cytotoxic receptors (NKp30, NKp44, and NKp46). Studies have confirmed that hypoxic microenvironments can downregulate the expression of NKG2D while NKG2A expression remains unaffected, which in turn leads to an imbalance in the expression of NKG2D/NKG2A receptor molecules and can be one of the reasons for the decrease in NK cell activity (19). 3) The decrease in interleukin 2 activity during hypoxia can also reduce NK cell activity and impair cellular immune function (20, 21). There were no significant differences in NK cell activity among the different subgroups of children with OSAHS in this study. Therefore, identifying the potential limit to the effects of OSAHS on NK cell activity in children requires further investigation.
Regarding the influence of disease course on the immune function of children with OSAHS, this study found that the T-lymphocyte subsets and NK cell activity in the peripheral blood of different subgroups (based on the course of disease, < 3 years and ≥ 3 years) showed no statistical significance, which was inconsistent with the expected results. The possible reasons for this finding are as follows: 1) Most children with OSAHS paid insufficient attention to the disease and could not accurately describe the specific onset period. 2) The number of cases in this study was small, and the rationality of defining the age of disease onset between different subgroups still requires further exploration. 3) The immune function of children with OSAHS may be affected by many factors.
Among the PSG indexes, AHI is a qualitative and quantitative index of sleep disorders, and LSaO2 can reflect the maximum degree of hypoxia in children during nighttime sleep. In this study, the proportions of T-lymphocyte subsets and NK cell activity in the peripheral blood of children with OSAHS were identified and utilized in correlation analyses with AHI and LSaO2. The results revealed that the percentages of CD4+, CD4+/CD8+, and NK cell activity decreased while the percentage of CD8+ increased, which was related to clinical severity and consistent with most existing studies (22).
In summary, OSAHS can affect the immune function of affected children. The serum T-lymphocyte subsets and NK cell activity levels were linearly correlated with PSG indexes, such as AHI and LSaO2, indicating that AHI and LSaO2 are two of the factors that may affect the cellular immune function of children with OSAHS. Although there is no significant difference in the immune function of children with different courses of disease, as the disease gets worse, the immune function of these children is obviously affected. Therefore, once the diagnosis is clear, regardless of the length of the disease, the disease should be treated according to severity of the disease. Moreover, timely intervention, prevention, and treatment of complications should be carried out to ensure the healthy growth of such children.
The following shortcomings exist in this study: 1) The number of cases was small. 2) We did not perform post-treatment studies on the T-lymphocyte subsets and NK cell activity in the peripheral blood of children with OSAHS. Although the aforementioned studies have confirmed that OSAHS can lead to a decline in the body’s immune function, further studies on the immune function of children with OSAHS may provide more powerful evidence and will comprise the next step of our study.
Acknowledgements
References
-
1.
American Academy of Sleep Medicine. International classification of sleep disorders. 3rd ed. M Darien, IL: American Academy of Sleep Medicine; 2014.
-
2.
Johnston J, McLaren H, Mahadevan M, Douglas RG. Clinical characteristics of obstructive sleep apnea versus infectious adenotonsillar hyperplasia in children. Int J Pediatr Otorhinolaryngol. 2019;116:177-80. [PubMed ID: 30554693]. https://doi.org/10.1016/j.ijporl.2018.11.004.
-
3.
Kiroglu AF, Noyan T, Oger M, Kara T. Oxidants and antioxidants in tonsillar and adenoidal tissue in chronic adenotonsillitis and adenotonsillar hypertrophy in children. Int J Pediatr Otorhinolaryngol. 2006;70(1):35-8. [PubMed ID: 15961164]. https://doi.org/10.1016/j.ijporl.2005.05.006.
-
4.
Zhang F, Tian Z, Peng S, Li J, Yang X, Mo H, et al. Exposure to intermittent hypoxia impairs learning and memory ability in rats. Sleep Biol Rhythms. 2018;16(3):331-6. https://doi.org/10.1007/s41105-018-0161-y.
-
5.
Gorman D, Ogston S, Hussain SS. Improvement in symptoms of obstructive sleep apnoea in children following tonsillectomy versus tonsillotomy: a systematic review and meta-analysis. Clin Otolaryngol. 2017;42(2):275-82. [PubMed ID: 27506317]. https://doi.org/10.1111/coa.12717.
-
6.
Akinnusi M, Jaoude P, Kufel T, El-Solh AA. Toll-like receptor activity in patients with obstructive sleep apnea. Sleep Breath. 2013;17(3):1009-16. [PubMed ID: 23239459]. https://doi.org/10.1007/s11325-012-0791-2.
-
7.
Kaditis AG, Alonso Alvarez ML, Boudewyns A, Alexopoulos EI, Ersu R, Joosten K, et al. Obstructive sleep disordered breathing in 2- to 18-year-old children: diagnosis and management. Eur Respir J. 2016;47(1):69-94. [PubMed ID: 26541535]. https://doi.org/10.1183/13993003.00385-2015.
-
8.
Santos FP, Weber R, Fortes BC, Pignatari SS. Short and long term impact of adenotonsillectomy on the immune system. Braz J Otorhinolaryngol. 2013;79(1):28-34. [PubMed ID: 23503904]. https://doi.org/10.5935/1808-8694.20130006.
-
9.
Renrick AN, Thounaojam MC, Dudimah DF, Thomas P, Pellom ST, Uzhachenko RV, et al. Bortezomib enhances expression of effector molecules in antitumor CD8+ T lymphocytes by modulating Notch-NF-kB-miR-155 crosstalk. Cancer Res. 2016;76(14):4161. https://doi.org/10.1158/1538-7445.am2016-4161.
-
10.
Wong YC, McCaughan GW, Bowen DG, Bertolino P. The CD8 T-cell response during tolerance induction in liver transplantation. Clin Transl Immunology. 2016;5(10). e102. [PubMed ID: 27867515]. [PubMed Central ID: PMC5099425]. https://doi.org/10.1038/cti.2016.53.
-
11.
Peppa D, Gill US, Reynolds G, Easom NJ, Pallett LJ, Schurich A, et al. Up-regulation of a death receptor renders antiviral T cells susceptible to NK cell-mediated deletion. J Exp Med. 2013;210(1):99-114. [PubMed ID: 23254287]. [PubMed Central ID: PMC3549717]. https://doi.org/10.1084/jem.20121172.
-
12.
Zhang Z, Wang C. Immune status of children with obstructive sleep apnea/hypopnea syndrome. Pak J Med Sci. 2017;33(1):195-9. [PubMed ID: 28367199]. [PubMed Central ID: PMC5368308]. https://doi.org/10.12669/pjms.331.11959.
-
13.
Dai ZY, Huang DY, Zhou CY. Effects of partial tonsillectomy on the immune functions of children with obstructive sleep apnea-hypopnea syndrome at early stage. Genet Mol Res. 2014;13(2):3895-902. [PubMed ID: 24615085]. https://doi.org/10.4238/2014.January.24.15.
-
14.
Ding Y, Zhou L, Xia Y, Wang W, Wang Y, Li L, et al. Reference values for peripheral blood lymphocyte subsets of healthy children in China. J Allergy Clin Immunol. 2018;142(3):970-973 e8. [PubMed ID: 29746882]. https://doi.org/10.1016/j.jaci.2018.04.022.
-
15.
Capdevila OS, Kheirandish-Gozal L, Dayyat E, Gozal D. Pediatric obstructive sleep apnea: complications, management, and long-term outcomes. Proc Am Thorac Soc. 2008;5(2):274-82. [PubMed ID: 18250221]. [PubMed Central ID: PMC2645258]. https://doi.org/10.1513/pats.200708-138MG.
-
16.
Dyugovskaya L, Lavie P, Hirsh M, Lavie L. Activated CD8+ T-lymphocytes in obstructive sleep apnoea. Eur Respir J. 2005;25(5):820-8. [PubMed ID: 15863638]. https://doi.org/10.1183/09031936.05.00103204.
-
17.
Section on Pediatric Pulmonology; Subcommittee on Obstructive Sleep Apnea Syndrome; American Academy of Pediatrics. Clinical practice guideline: diagnosis and management of childhood obstructive sleep apnea syndrome. Pediatrics. 2002;109(4):704-12. [PubMed ID: 11927718]. https://doi.org/10.1542/peds.109.4.704.
-
18.
Landis CA, Lentz MJ, Tsuji J, Buchwald D, Shaver JL. Pain, psychological variables, sleep quality, and natural killer cell activity in midlife women with and without fibromyalgia. Brain Behav Immun. 2004;18(4):304-13. [PubMed ID: 15157947]. https://doi.org/10.1016/j.bbi.2003.11.001.
-
19.
Verheyden S, Demanet C. NK cell receptors and their ligands in leukemia. Leukemia. 2008;22(2):249-57. [PubMed ID: 18046448]. https://doi.org/10.1038/sj.leu.2405040.
-
20.
Konjevic G, Vuletic A, Mirjacic Martinovic K. Natural killer cell receptors: alterations and therapeutic targeting in malignancies. Immunol Res. 2016;64(1):25-35. [PubMed ID: 26374324]. https://doi.org/10.1007/s12026-015-8695-4.
-
21.
Huang YS, Guilleminault C, Hwang FM, Cheng C, Lin CH, Li HY, et al. Inflammatory cytokines in pediatric obstructive sleep apnea. Medicine (Baltimore). 2016;95(41). e4944. [PubMed ID: 27741107]. [PubMed Central ID: PMC5072934]. https://doi.org/10.1097/MD.0000000000004944.
-
22.
Tan HL, Gozal D, Wang Y, Bandla HP, Bhattacharjee R, Kulkarni R, et al. Alterations in circulating T-cell lymphocyte populations in children with obstructive sleep apnea. Sleep. 2013;36(6):913-22. [PubMed ID: 23729935]. [PubMed Central ID: PMC3648675]. https://doi.org/10.5665/sleep.2724.